ABSTRACT
The harlequin fish (Othos dentex) is the largest serranid found in the temperate waters of southern Australia. Acoustic telemetry was used to continuously track the movements and activity patterns of 10 harlequin fish (330–620 mm total length; 0.5–3 kg weight) for a 16-month period at a coastal reef site. Data showed that the harlequin fish is a site-attached, diurnal predator, with a relatively small home range in comparison with other temperate reef fishes from Australia and New Zealand. These characteristics indicate that the harlequin fish is susceptible to localised depletions from fishing, but that it can be protected within no-take marine protected areas and can be detected with appropriate daytime monitoring techniques. Individuals also displayed discrete depth preferences on the reef slope, evidence of a nocturnal home base, and homing ability following disturbance from an extreme storm event.
Introduction
The Serranidae is a worldwide family of marine fishes with hundreds of species occurring in the Australasian region alone, including Australia and New Zealand. However, unlike tropical Australasian waters, there are relatively few serranids in the cold temperate waters of southern Australia where the harlequin fish (Othos dentex) is the largest serranid (about 860 mm total length [TL]) and only member of its genus (Roberts & Gomon Citation2008; French et al. Citation2014). The harlequin fish is endemic to the region between southwestern Western Australia (WA) and western Victoria where it prefers shallow (<30 m depth) rocky reefs with drop-offs, crevices and caves in clear coastal waters (Kuiter Citation1999; Roberts & Gomon Citation2008). The harlequin fish lives to at least 42 years of age and is a gonochorist with no sex change (French et al. Citation2014), which is unusual among serranids yet interestingly also occurs in another southern Australian endemic serranid, the breaksea cod, Epinephelides armatus (Moore et al. Citation2007). The harlequin fish likely plays an important role as an apex predator of smaller fishes in temperate reef ecosystems of southern Australia (Bryars & Shepherd Citation2013).
The harlequin fish is incidentally captured on hook and line by fishers but is also targeted by recreational spearfishers (Fowler et al. Citation2009; Saunders et al. Citation2010; French et al. Citation2014). There is little protection for harlequin fish, with no limits on recreational take in South Australia (SA) and a bag limit (but no size limit) on recreational take in WA. The life history characteristics of high longevity and proposed pair spawning make the harlequin fish intrinsically vulnerable to fishing (Saunders et al. Citation2010; French et al. Citation2014). In addition, harlequin fish are susceptible to barotrauma and post-release survival rates may be low (S. Bryars, pers. obs.). Coastal habitat degradation could also be a threat in some regions; the disappearance of harlequin fish from Port Phillip Bay in Victoria has been linked to poor water quality (Gomon Citation2001). Consequently, the harlequin fish is considered to be of conservation and management concern. Further information is required to understand the potential impacts of human processes on populations and the potential role of no-take marine protected areas (MPAs) as a conservation management tool.
Harlequin fish are recorded on surveys using underwater visual census (UVC) (Shepherd & Baker Citation2008) and baited remote underwater video systems (BRUVS) (Harvey et al. Citation2012). However, information is lacking on the site fidelity and behaviour of the harlequin fish that may assist with interpretation and design of population surveys and with assessment of the efficacy of no-take MPAs to provide adequate levels of protection for all life history stages. In the present study, acoustic telemetry was used to determine long-term patterns in site fidelity, home range, alongshore movements, depth use and diel activity of harlequin fish on a high-relief temperate reef ecosystem. Behavioural information will inform not only survey monitoring methodologies and MPA design and evaluation, but also allow broader comparisons with temperate and tropical reef fishes from across the Australasian region and the world.
Materials and methods
Study site
The study site was located to the east of the township of Penneshaw in Backstairs Passage off Kangaroo Island, SA (). The site is characterised by a relatively narrow strip of high-relief coastal reef (<50 m width) that slopes steeply from cliffs into a relatively flat area at c. 12–14 m depth. The flatter area adjacent to the reef is characterised by a mixture of sparse seagrass, bare sand and patchy low-relief reef with sponges and other filter-feeding invertebrates. Harlequin fish inhabit the strip of high-relief reef (S. Bryars, pers. obs.). While the tidal amplitude at the site is only c. 1.5 m, it experiences relatively strong alongshore tidal flows resulting from flow in and out of Gulf St Vincent through the constriction of Backstairs Passage (Bye & Kämpf Citation2008, B).
Figure 1. Map showing: A, The study region within Australia; B, the Ironstone Hill study site on NE Kangaroo Island in relation to Gulf St Vincent and Backstairs Passage; C, the locations of the seven receivers adjacent to Ironstone Hill. The circles around each receiver indicate a 200 m detection radius.
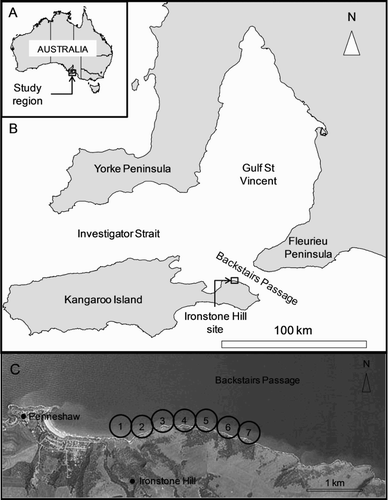
Receiver deployments
A total of seven VR2W acoustic receivers (Vemco Division, Amirix Systems Inc) were deployed at the field site. The Vemco acoustic tracking technology requires the receivers to be moored to the seafloor where they passively detect and log the signals of individual fish that have been tagged with coded transmitters (Heupel et al. Citation2006, www.vemco.com). Receivers are separated by a distance that is a function of the detectable range of the signal from the transmitters. To inform the appropriate distance between the receivers in the present study, results from range testing and fish detections at a comparable site in a previous study were used (Bryars et al. Citation2012). A nominal spacing interval of 400 m between receivers was used at the study site (C).
Deployment of receivers was undertaken on 9 June 2010. Receivers 2 to 7 were located c. 50–100 m offshore from the edge of the coastal reef in 10–19 m depth. Receiver 1 was located farther offshore (c. 200 m) due to a shallow embayment and was set in c. 8 m depth. The seven receivers encompassed a total distance of c. 2.4 km. Receivers were affixed with cable ties to 1.65 m long steel posts that had been hammered into the sand such that receivers were c. 1 m above the seabed and the hydrophone was clear of the top of the post.
Transmitter deployments
A single, individually coded acoustic transmitter (Vemco, V13P-1H, 13 mm diameter, 45 mm length, 12 g weight in air, 6 g weight in water) was surgically implanted into the stomach cavities of 10 harlequin fish (O. dentex). Transmitters were set with a minimum and maximum delay of 110 and 250 seconds, respectively, with a nominal delay of 180 seconds. All transmitters were fitted with pressure sensors set for a depth range of 0–50 m, which covered the range of depths found at the study site. Transmitters had an estimated battery life of 511 days.
Harlequin fish were tagged between 7 June 2010 and 11 June 2010 (). Fish were captured in the middle part of the receiver array (between receivers 3 and 5) by scuba divers using a handheld dab net at depths of <12 m from along the high-relief reef. Fish were slowly brought to the surface to reduce the chance of barotrauma. Surgical procedures were as described in Bryars et al. (Citation2012); variations from this were an anaesthetic concentration of 40 ppm during induction that was reduced to 20 ppm during operation, a 1/0 size needle, no venting occurred, and the recovery period was 20–60 min. Total body length was measured to the nearest 1 cm, weight was estimated to the nearest 0.5 kg and (where possible) sex was able to be determined post-study after French et al. (Citation2014) using total length data and external coloration from photographs taken at the time of capture. Once fully recovered, fish were released underwater by scuba divers at their capture location.
Table 1. Summary of the 10 harlequin fish tagged within the receiver array.
As the detection range and diel pattern of detections can vary depending upon ambient conditions (Payne et al. Citation2010), a single ‘control’ transmitter (Vemco, V13-1H, 13 mm diameter, 45 mm length) was deployed for the duration of the study to aid interpretation of fish transmitter data. The control transmitter was located approximately midway between receivers 3 and 4 () where it was attached by monofilament line to an anchor and a subsurface float such that it was situated c. 1 m above the seabed. The control transmitter was set with a minimum and maximum delay of 300 and 900 seconds, respectively, with a nominal delay of 600 seconds.
Recovery of acoustic detection data
Acoustic detection data were downloaded from receivers on 19 October 2010, 24 January 2011 and 6 October 2011, when the study was terminated after 16 months. Receiver 7 could not be located at the final download and so data are missing from the eastern extreme of the array for the second half of the study.
Analyses utilised data from the 482 full days of data collection commencing on 11 June 2010 when all receivers were in place and all fish had been tagged, and finishing on 5 October 2011 when the final 24-h period of data collection occurred. While data from receiver 7 were missing for the last 255 days of the study, results from the previous 227 days indicated that it was unlikely that the receiver would have logged much detection. Therefore, the partial absence of receiver 7 was ignored when conducting analyses involving the full receiver array and study period. Data from the control transmitter were used to inform interpretations about site fidelity, any possible alongshore movements, and diel activity patterns. Detection efficiency of the control transmitter was calculated as the total number of detections made at each receiver divided by the total number of transmissions possible across the 482 days using the nominal delay of 10 minutes for the transmitter (i.e. 69,408 transmissions).
Site fidelity
Site fidelity or residence time for each fish was calculated by dividing the total number of days with ≥1 detection in the receiver array by the total number of days available for detection (482 days). Although some studies utilise a cut-off of ≥2 detections per day (e.g. Green & Starr Citation2011) and Vemco recommends caution when interpreting single detections, based upon the cryptic habit of the harlequin fish and the high level of site fidelity observed, it was assumed that single detections were real, i.e. it was highly likely that a fish was present within the array but was not particularly active and/or at the detection range limits of the receivers.
Home range
Home range can be defined as the area used by an individual during normal activities and is commonly defined as the area in which an individual spends 95% of its time (Tolimieri et al. Citation2009). Due to the linearity of the receiver array, traditional home range techniques such as kernel density estimates could not provide effective area-based home range estimates. Instead, home range area and alongshore length were calculated after Bryars et al. (Citation2012) in which home range lengths are calculated as the sum of distances (plus 200 m) between the receivers that account for 95% of all detections. Calculations commence with the receiver with the greatest number of detections and then progress to the adjacent receiver with the next highest total, and so on. The additional 200 m is added to account for the distance (i.e. 100 m) at which detection frequency begins to decline significantly (Bryars et al. Citation2012). Thus, in the case of a single receiver that accounts for the entire home range (i.e. 100% of detections), the range is 200 m. Home range areas are then calculated by multiplying the range lengths by the width of reef utilised. In the present study, reef width utilised by each fish was calculated using the depth range that accounted for 95% of all depth detections, a mean reef width of 50 m in the middle part of the array (between receivers 3 to 5) and a mean depth of 12 m at the reef edge. As this calculation technique can generate considerable over-estimates of home range size (see Bryars et al. Citation2012), calculations were limited to the five fish with the largest number of detections and values are presented as indicative maximum values only.
Alongshore movements
Analysis of alongshore movements was conducted by plotting individual receiver detections against time. Alongshore movements were considered to be real when: (1) a detection(s) was made at >1 receiver distance away from the ‘home base’ receiver where the majority of detections had been made prior to the new detection; and (2) the detection(s) was not simultaneous with a detection at the home base receiver. This rule was applied because: (1) while in some cases fish appeared to have their home range close to a single receiver (and all detections occurred at this receiver), other fish appeared to be based in between two receivers and in these cases ‘normal’ detection patterns involved two adjacent receivers; and (2) detection distance varied with environmental conditions, as evidenced by the control transmitter which was occasionally simultaneously detected at much greater distances than usual (up to 800 m).
Depth use
To examine general patterns of depth utilisation for each fish, depth data were binned into 1 m depth classes and plotted as the percentage of total detections versus depth class. To allow for a diel influence on activity (see ‘Diel activity patterns’), depth data were separated into two 7-h periods of night (2100–0400 h) and day (0900–1600 h) which eliminated crepuscular hours around dawn and dusk (see Green & Starr Citation2011) and also allowed for the influence of daily variation in the times of dawn and dusk across a year. To test for a statistical difference in depth utilisation between day and night, coefficients of variation (representing a standardised measure of dispersion) for each fish were utilised in a paired t-test.
Diel activity patterns
Payne et al. (Citation2010) demonstrated that caution must be exercised when interpreting diel activity patterns from acoustic detection data, and their approach was adopted in analysing the detection data for possible diel patterns. Detections for each fish were summed for each hour of each day throughout the entire array, and mean detection frequency per hourly bin was calculated based upon the total number of days each fish was detected within the array (). For each fish, these detection frequencies were divided by the grand mean detection frequency as calculated from the 24 hourly bins to generate standardised detection frequencies (SDFs) that correct for the variable magnitude of detection patterns among individuals. Using data from the single fixed-location control transmitter, the approach of Payne et al. (Citation2010) was employed to correct for environmental changes in detection frequency for each fish by using the control SDFs per hourly bin. Data from the entire 482 detection days of the control transmitter were used in the correction. To test for a statistical difference in activity between day and night, control-corrected SDFs from each fish for midnight (0000–0100 h bin) and midday (1200–1300 h bin) were utilised in a paired t-test.
Results
Fish capture and size
In June 2010, 10 harlequin fish were captured, tagged by surgical insertion of an acoustic transmitter and released during daylight hours between receivers 3 and 5 (). Tagged fish ranged in size from 330–620 mm TL and weighed 0.5–3 kg (). Based upon spot coloration and length, Fish 6 and 7 with blue spots and both >560 mm TL were sexually mature males, while Fish 3, 4, 8 and 9 with yellow spots and all >450 mm TL were likely sexually mature females (size at maturity: Male upper LT95 = 428 mm, Female upper LT95 = 433 mm, French et al. Citation2014). Sex in the remaining four fish could not be predicted.
Temporal patterns of detection
A total of c. 280,000 detections were recorded from the 10 fish during the 16-month study period (, ). On the final full day available for analyses (5 October 2011), seven of the tagged fish (3 to 9) were detected within the array (, ). Fish 3, 5, 7, 8 and 9 were detected almost every day over the 16-month study period (, ). In contrast, Fish 10 was detected for just 5 days (, ). Fish 2 was detected on most days up until 10 July 2010 but was absent for 174 days until a single detection was recorded on 31 December 2010; giving a total of just 26 days detected (5.4%, , ). Fish 1 was detected regularly up until 26 July 2011 when detections ceased at 411 days after the first detection, for a total of 257 (53.3%) days detected (, ). Even for fish that were still being detected at the end of the study, there was considerable variation in the total number of detections (); Fish 3, 5, 7, 8 and 9 had by far the greatest number of detections (>34,000 each), whereas Fish 4 and 6 each recorded <8000 detections (). The number of detections was not related to fish length for the seven fish still being detected at the completion of the study (linear regression: F1,6 = 2.57, P = 0.169).
Control transmitter patterns of detection
The control transmitter was detected at least once every day of the study period. Around 60,000 detections were recorded with 69% logged at receiver 3, 30% at receiver 4 and the small remainder (c. 1%) at receivers 1 and 2 (). Detection efficiency at receivers 3 and 4 (which were c. 200 m from the control transmitter) was 60% and 26%, respectively. These results indicate that at >200 m from a receiver the chance of a detection in the habitat type within the study area was substantially lowered and is consistent with previous range testing which showed that detection efficiency begins to decline significantly at >100 m distance (S. Bryars, unpubl. data).
Site fidelity
Fish 3, 5, 7, 8 and 9 (50% of the total number tagged) had a high degree of site fidelity with residence times of >97% (). Fish 4 and 6 had slightly lower residence times of 87% and 85%, respectively, but were still being detected within the array at the completion of the 16 month study (). Fish 1 had a moderate residence time of 53% (, ); if residence time is calculated using the total number of days between first and last detections then it was present for 63% of the monitored time. Fish 2 and 10 had low residence times (≤5%, ) due to their disappearance from the array after a short period (); however, prior to their disappearance they were present for 87% and 100% of that time, respectively (in this particular case ignoring the single detection for Fish 2 on 31 December 2010). Most individuals had a high degree of fidelity to a single receiver during the time they were detected; eight of the fish had >90% of all detections at a single receiver (Fish 1 and 2 at receiver 3; Fish 3, 4, 5, 7 and 9 at receiver 4; Fish 8 at receiver 6).
Home range
Estimates of home range were made for Fish 3, 5, 7, 8, and 9 (mean TL = 514 mm) that had the highest detections (>34,000, with next highest at c. 7400 for Fish 4) and that remained within the receiver array for almost the entire 16-month study period. Each of these fish had >95% of detections at one receiver, and the alongshore home range length of these fish was a maximum of 200 m and the home range area a maximum of 6000 m2.
Alongshore movements
While fish generally had a high degree of site fidelity, closer examination of presence and depth data over time indicated that: (1) Fish 10 may have been predated upon early in the study; (2) during July 2010, Fish 2, 3, 6, 7 and 8 undertook a short-term but relatively long-distance movement along the coast and into deeper water; and (3) at this same time Fish 3, 6 and 7 returned to their home range, Fish 8 relocated its home range and Fish 2 left the study area.
Fish 10 was detected for just 5 days during which it displayed a pattern of alongshore movement and depth use that were atypical of the other nine individuals. For the first few days, it was detected mainly near receiver 4 across a range of depths from c. 4–15 m. However, around midnight on 14 June 2010 it was detected in very shallow water (2 m) near receiver 4. The next detection was at 16 m depth just 17 min later at receiver 3. Over the next 2.5 h there was a rapid westward movement indicated by a series of detections on receivers 3, 2 and 1, which were accompanied by a series of rapid depth movements ranging between 0 and 16 m depth. No other fish was detected at 0 m depth. The final detection for Fish 10 was at 0300 h in 7 m depth at receiver 1 in the western extreme of the array.
On the morning of 10 July 2010, seven fish displayed distinct changes in their pattern of alongshore movements and/or depth use (, 4). After previously only being detected at just one or two receivers (3 and 4, or 4 and 5) within the middle of the study area for about 1 month, Fish 2, 3, 6, 7 and 8 were suddenly detected at receivers 5, 6 and/or 7 to the east. For each of these fish, this alongshore movement was accompanied by forays down the reef slope to depths of c. 15–18 m (deeper than any previously recorded, ). The alongshore pattern of detections by these fish was not replicated by the control transmitter, which was only detected at receivers 1, 3 and 4 during this time period () and was therefore concluded to be a real pattern of detections rather than a series of false detections. Fish 3, 6 and 7 returned to their original home bases after a period of apparent searching (, 4). Fish 8 after a period of apparent searching permanently relocated its home base from near receiver 4 to 6 (, 4). Fish 2 appeared to leave the array permanently (, 3, 4). Patterns of detections for Fish 1, 4, 5 and 9 during the period 10–15 July 2010 were also unusual with lowered detection frequency for some individuals and a deepwater excursion by Fish 1 (, 4). It is possible that some of these fish also left the middle part of the array during this time.
Diel activity and patterns of depth use
Diel patterns of activity were similar among individuals, with the relative frequency of detections increasing at dawn and decreasing quickly at dusk (). Some individual variability in diel activity was displayed (e.g. Fish 5 and 8, see ). Mean control-corrected SDF was significantly greater at midday than midnight (paired two-tailed t-test: t = –9.38, d.f. = 8, P < 0.001).
Figure 5. Diel activity pattern for all fish combined: mean (±SE) control-corrected standardised detection frequency per hourly bin for harlequin Fish 1 to 9.
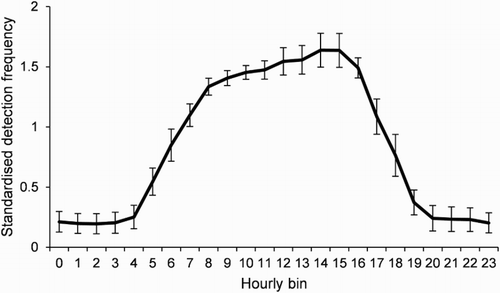
Figure 6. Depth utilisation by harlequin Fish 3, 5, 7, 8 and 9 during the day and night. Data are percentages of the total number of depth detections across 1 m depth classes for day (0900–1600 h) and night (2100–0400 h). Depth classes are 0–1 m (=1), 1–2 m (=2), etc. Note that the y axis range varies between fish. The black bar on the day graphs indicates the night modal depth.
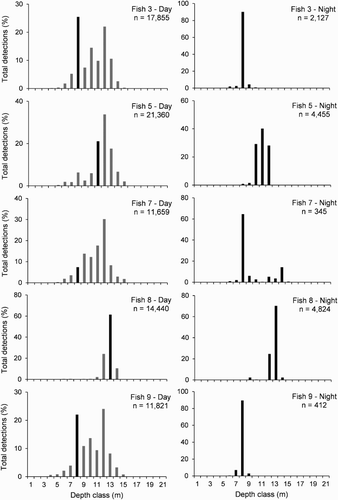
Individual fish utilised a range of depths during the daytime but depth use was non-uniform (). Some fish had a unimodal distribution of detections (Fish 5, 7 and 8), while others had a bimodal distribution (3 and 9; ). All fish (including Fish 1, 2, 4 and 6 that are not shown in ) had relatively little activity at depths <6 m and at depths >13 m, which is at or near the reef edge. For those fish that had a moderate number of night detections (viz. 3, 5, 7, 8 and 9), patterns of depth utilisation varied noticeably between day and night (). Except in Fish 7 and 8, during the day a wide range of depths across at least 10 m of the reef slope were used while at night a more restricted range of depths was detected. Fish 8 was detected across a small range of 4–6 m at both day and night (). For Fish 7 in which there were a few detections at deeper depths of 12–14 m during the night (), these were all during the July 2010 period when the fish underwent an unusual alongshore movement into deeper waters and were thus not representative of the rest of the study period. Using the coefficient of variation for Fish 3, 5, 9 and 7 (minus the July 2010 period), the apparent difference between day and night depth distribution was found to be statistically significant (paired two-tailed t-test: t = 6.60, d.f. = 3, P < 0.01). For each of those fish (Fish 3, 5, 7 and 9), the night modal depth did not correspond with the day modal depth and was shallower than the day modal depth (). This suggests that during the daytime these fish were moving from their night home base down the reef slope into deeper water and then returning at night. Indeed, examination of fine-scale temporal data showed that most fish (Fish 1, 3–7 and 9) displayed movements up and down the reef slope during the daytime with some excursions away from the reef edge which lies in c. 12–14 m depth. The temporal patterns of depth detections also indicated that fish were remaining within narrow depth ranges for periods of up to several hours during the day (data not presented).
Discussion
This study provides strong evidence that the harlequin fish is site-attached and has a relatively small home range. These traits are typical of many serranids around the world (DeLoach & Humann Citation1999; Afonso et al. Citation2016). Other southern Australian reef fishes, such as the western blue groper (Achoerodus gouldii), bluethroat wrasse (Notolabrus tetricus) and senator wrasse (Pictilabrus laticlavius), also show a high degree of site fidelity and occupy a small home range (Barrett Citation1995; Edgar et al. Citation2004a; Shepherd et al. Citation2009; Bryars et al. Citation2012). While it was not possible to provide accurate estimates of home range for the harlequin fish, it is likely that their approximate home range was <200 m in alongshore length and <6000 m2 in area. In comparison with other resident temperate reef teleosts from Australia, New Zealand and North America, the harlequin fish appears to be at the lower end of the home range area spectrum (see Bryars et al. Citation2012).
The high site fidelity and relatively small home range of the harlequin fish (combined with high longevity) makes individuals vulnerable to fishing effort concentrated over their preferred habitat, and therefore meta-populations are likely to be afforded at least partial protection within relatively small no-take MPAs. Nonetheless, an adequate no-take MPA needs to allow for sporadic alongshore/offshore movements outside of the home range and home range relocations of individual fish (see Kramer & Chapman Citation1999). Thus a precautionary approach that incorporates buffer areas would suggest that an MPA of several km in alongshore length and width is required to fully encompass a population of harlequin fish at locations such as the study site. Significantly, a relatively large no-take MPA (or ‘sanctuary zone’) has now been proclaimed to the east of the study site, extending about 9 km alongshore and up to 10 km offshore in parts (DEWNR Citation2012); this no-take MPA, and a number of others proclaimed around South Australia, should have tangible conservation and fisheries management benefits for the harlequin fish.
Results of this study indicate that the harlequin fish is a diurnal predator. Other evidence that harlequin fish are diurnal comes from the BRUVS-based work of Harvey et al. (Citation2012) in which, despite night sampling, harlequin fish were only recorded during the daytime. Many other serranids are diurnal (DeLoach & Humann Citation1999; Afonso et al. Citation2016), as are some other sympatric reef fishes such as the western blue groper (Bryars et al. Citation2012). As harlequin fish do not move more than a few metres above their reef habitat into the water column (i.e. they are demersal), the depth data can be interpreted as vertical distance from the water surface to the reef. Thus it is apparent that during the daytime individual fish actively moved up and down the reef slope. This behaviour was punctuated by sedentary periods where depth remained constant. Such a pattern is consistent with field observations in which fish regularly rest on the reef. It is also likely that these fish were spending some of this time at ambush and cleaning stations as has been observed in the field and documented in previous studies (Shepherd et al. Citation2005; S. Bryars, pers. obs. and unpubl. data). In addition, multiple resights of the same individual using photo identification across many months have occurred at the same reef ledge in another study location (S. Bryars, unpubl. data). In the present study, most fish had one or more distinct depth modes of activity () and it is possible that these were related to utilisation of prey ambush/cleaning stations and/or home bases.
Diel depth data indicated that following the daytime period of activity, individuals were returning to a home base with a set depth each night and that movements away from this depth at night were limited. Observations of fish in the field show that they utilise caves or ledges when confronted by divers (S. Bryars, pers. obs.) and it is likely that they utilise these same habitats at night for resting and predator avoidance. The reduced frequency of detections by receivers at night could also be partly explained by such behaviour as solid structures may partially impede acoustic signals. The pattern is consistent with a species that has a small home range for diurnal activities and a nocturnal home base. Such a pattern has been seen in some other temperate reef fish, such as the California sheephead (Semicossyphus pulcher) (Topping et al. Citation2005). Further investigation to confirm the fine-scale movements of harlequin fish could be undertaken using a multi-receiver positioning system (Espinoza et al. Citation2011). Nonetheless, it appears that the long-term movement patterns of the harlequin fish are quite different to the coral trout (Plectropomus leopardus), a similarly sized, but tropical serranid. The coral trout appears to range over several kilometres of reefs as a mobile, opportunistic predator, but also maintains home sites for access to shelter and cleaning stations (Samoilys Citation1997a). In contrast, the harlequin fish in the present study utilised much smaller spatial scales, which were more similar to the northern hemisphere temperate serranids, the dusky grouper (Epinephelus marginatus) and the blacktail comber (Serranus atricauda) (Afonso et al. Citation2016).
Given the high rugosity and complexity of the reef system and the demersal, sedentary behaviour of harlequin fish, the high detection frequencies recorded for some tagged individuals was somewhat unexpected. In contrast, some individuals had a much lower number of detections. Explanations for this could be related to variation in activity levels and/or differences in the fine-scale area of occupancy of individual fish within the reef complex (and thus differences in distance and ‘line-of-sound’ to the closest receiver). Nonetheless, results of this study show that the type of technology used can be employed successfully on sedentary species such as the harlequin fish in high-relief reef habitats to investigate long-term site fidelity. Study results also demonstrate the usefulness of long-term acoustic data for site-attached reef fish, as other methods such as visual tagging and tracking (e.g. Barrett Citation1995; Edgar et al. Citation2004a) may have missed the alongshore movement that were detected during July 2010, and photographic ID does not provide suitable metrics for resolving unobserved variations in animal behaviours and is reliant on physical characteristics to be temporally stable over multiple seasons.
Although many species of serranids, including the coral trout which aggregates around new moon periods (Samoilys Citation1997b), move outside their home range to participate in spawning activity (DeLoach & Humann Citation1999), the onset of the movement of harlequin fish was not correlated with a new or full moon and it occurred in midwinter which is outside of the usual spawning period (French et al. Citation2014). The temporary alongshore movement of harlequin fish was found to be correlated with an unusual extreme storm event. Maximum wind speed recorded on 10 July 2010 was a direct onshore northerly of 126 km h−1 which was the strongest wind speed recorded from any direction throughout 2010 and 2011 at nearby Cape Willoughby. The maximum speed was far greater than the mean wind speed of 27 km h−1 recorded for Cape Willoughby during the study period (Bureau of Meteorology data). As the wind was directly from the north, the fetch over which waves could be generated was close to the maximum possible for the study site at c. 145 km (see ). Strong northerly winds can cause substantial wave energy along the northern coastline of Kangaroo Island and this event correlated with movement of harlequin fish from shallower inshore waters to deeper offshore waters further along the coast. All fish that moved alongshore initially moved eastwards; water depths immediately offshore from the coastal reef are substantially greater in the eastern part of the study array (>20 m depth) than to the west (<10 m depth). Storm activity has been correlated with the onset of coordinated movement in other site-attached reef fish such as the black rockfish (Sebastes melanops) (Green & Starr Citation2011).
Of interest during this study was that, while some fish were able to return to their home base following the storm event, one individual apparently relocated outside of the study area, and another fish relocated its home base within the area. Other site-attached fishes are able to return to their home bases when displaced to a different location (e.g. Lowry & Suthers Citation1998; Jadot et al. Citation2006). However, it appears that both the distance of displacement and weather conditions can influence the ability to successfully return (Lowry & Suthers Citation1998; Jadot et al. Citation2006). In the present study, harlequin fish displayed homing ability because, following their alongshore movement, they then actively moved up and down the coast searching for their home base and in some cases took several days to return. However, it appears that two of the fish were not able to find their original home base and relocated elsewhere. This behaviour reinforces the need for consideration of seasonal and species-specific factors when planning the size of no-take MPAs (see Kramer & Chapman Citation1999).
Behavioural results of this study have implications for the design and interpretation of fish surveys targeting harlequin fish, which are usually relatively rare in survey data sets (e.g. Shepherd & Baker Citation2008). As harlequin fish are diurnal, UVC and BRUVS should be conducted during the daytime. With UVC, in which transect lines are traditionally laid parallel to the shoreline at set depths (e.g. Barrett et al. Citation2007), it is apparent that at the Ironstone Hill study site there would be a much greater chance of encountering harlequin fish along certain depth strata as tagged fish rarely ventured into depths <6 m or deeper than the reef edge. Clearly, surveys using snorkel at the surface would be ineffective for this species. The complete avoidance of very shallow depths (<2 m) by large harlequin fish (>30 cm TL) appears to be a general habit as: (1) divers failed to locate any fish in <2 m depth during the tagging phase of the study; (2) searches at multiple sites around SA have failed to find fish in <2 m depth (S. Bryars, pers. obs.); and (3) the depth range is reported as 2–30 m (Edgar Citation2008). UVC is known to incorporate biases (Edgar et al. Citation2004b) and information from the current study could potentially assist with the interpretation of UVC data on harlequin fish. However, the distribution and habit of small fish (<30 cm TL) is currently unknown, which has implications for surveys and adequate protection of populations inside no-take MPAs. It is possible that juvenile harlequin fish display cryptic behaviour as seen in some other Australian reef fishes (Fairclough Citation2016) and this would make them difficult to detect with UVC.
One of the harlequin fish (Fish 10) displayed a particularly unusual pattern of depth use and alongshore movement before it disappeared just 5 days post-tagging. As Fish 10 moved right to the surface at one stage and moved out of the acoustic array at an extremely rapid rate, it is possible that it was captured by a fast-moving marine mammal. Fish 10 was the smallest of the fish tagged at 33 cm TL and c. 0.5 kg weight. While the size of the transmitter was 1.2% of its body weight and this did not break the 2% ‘tag-to-body mass rule’ (Childs et al. Citation2011), Fish 10 may have suffered complications post-surgery and been in poor condition or dead at the time of its apparent capture and disappearance from the study site.
In summary, the acoustic tracking results indicate that the harlequin fish is a site-attached, diurnal predator, with a relatively small home range. These characteristics, which had not been demonstrated prior to this study, make populations vulnerable to localised depletions from fishing and other impacts. However, harlequin fish are amenable to protection inside adequately sized and appropriately located no-take MPAs and can also be detected during the daytime with appropriate monitoring techniques. The inclusion of critical habitats that include high-relief reef with caves and ledges in areas where harlequin fish are found is crucial for protection of this particular species. The diurnal predatory habit of harlequin fish (which is the largest serranid in southern Australia) suggests that it may play an important ecological role in the functioning of reef ecosystems of southwestern Australia.
Acknowledgements
Thanks to the following DEWNR staff who assisted with the project: D Miller, D Colella, H Rutherford, J Brook, P Copley, B McDonald, C Thomas, R Morcom and A Wright. Thanks also to C Huveneers (SARDI and Flinders University of SA) and N Payne (University of New South Wales) for technical assistance. The project had Animal Ethics approval from the SA Wildlife Ethics Committee (No. 35-2008-M4). B Page, P von Baumgarten, R Paxinos and A Burnell (all from DEWNR) provided constructive comments on a draft version of the manuscript. The manuscript was improved by feedback from two anonymous reviewers.
Associate Editor: Professor Kendall Clements.
Disclosure statement
No potential conflict of interest was reported by the authors.
Additional information
Funding
References
- Afonso P, Abecasis D, Serrao Santos R, Fontes J. 2016. Contrasting movements and residency of two serranids in a small Micronesian MPA. Fish Res. 177:59–70. doi: 10.1016/j.fishres.2015.12.014
- Barrett NS. 1995. Short- and long-term movement patterns of six temperate reef fishes (Families Labridae and Monacanthidae). Mar Freshwater Res. 46:853–860. doi: 10.1071/MF9950853
- Barrett NS, Edgar GJ, Buxton CD, Haddon M. 2007. Changes in fish assemblages following 10 years of protection in Tasmanian marine protected areas. J Exp Mar Biol Ecol. 345:141–157. doi: 10.1016/j.jembe.2007.02.007
- Bryars S, Rogers P, Huveneers C, Payne N, Smith I, McDonald B. 2012. Small home range in southern Australia’s largest resident reef fish, the western blue groper (Achoerodus gouldii): implications for adequacy of no-take marine protected areas. Mar Freshwater Res. 63:552–563. doi: 10.1071/MF12016
- Bryars S, Shepherd SA. 2013. Ambush predators and night stalkers. In: Shepherd SA, Edgar GJ, editors. Ecology of Australian temperate reefs: the unique south. Collingwood: CSIRO Publishing; p. 399–400.
- Bye JAT, Kämpf J. 2008. Physical oceanography. In: Shepherd SA, Bryars S, Kirkegaard I, Harbison P, Jennings JT, editor. Natural history of Gulf St Vincent. Adelaide: Royal Society of South Australia; p. 56–70.
- Childs AR, Næsje TF, Cowley PD. 2011. Long-term effects of different-sized implanted acoustic transmitters on the sciaenid Arygyrosomus japonicus: breaking the 2% tag-to-body mass rule. Mar Freshwater Res. 62:432–438. doi: 10.1071/MF10219
- DeLoach N, Humann P. 1999. Reef fish behaviour: Florida, Caribbean, Bahamas. Jacksonville, FL: New World Publications. 359 p.
- Department of Environment, Water and Natural Resources. 2012. Encounter marine park management plan 2012 [Internet]; [cited 2015 Nov 24]. Available from: http://www.environment.sa.gov.au/marineparks/home
- Edgar GJ. 2008. Australian marine life. The plants and animals of temperate waters. Sydney: Reed New Holland. 624 p.
- Edgar GJ, Barrett NS, Morton AJ. 2004a. Patterns of fish movement on eastern Tasmanian rocky reefs. Environ Biol Fish. 70:273–284. doi: 10.1023/B:EBFI.0000033342.89719.39
- Edgar GJ, Barrett NS, Morton AJ. 2004b. Biases associated with the use of underwater visual census techniques to quantify the density and size-structure of fish populations. J Exp Mar Biol Ecol. 308:269–290. doi: 10.1016/j.jembe.2004.03.004
- Espinoza M, Farrugia T, Webber D, Smith F, Lowe CG. 2011. Testing a new acoustic telemetry technique to quantify long-term, fine-scale movements of aquatic animals. Fish Res. 108:364–371. doi: 10.1016/j.fishres.2011.01.011
- Fairclough DV. 2016. Similar cryptic behaviour during the early juvenile phase of two unrelated reef fishes: Epinephelides armatus and Bodianus frenchii. Mar Freshw Behav Phy. 49:109–117. doi: 10.1080/10236244.2015.1125122
- Fowler AJ, Lloyd M, Schmarr D. 2009. A preliminary consideration of by-catch in the marine scalefish fishery of South Australia. South Australian Research and Development Institute (Aquatic Sciences), Adelaide, F2009/000097-1. SARDI Research Report Series No 365. 89 p.
- French B, Potter IC, Hesp SA, Coulson PG, Hall NG. 2014. Biology of the harlequin fish Othos dentex (Serranidae), with particular emphasis on sexual pattern and other reproductive characteristics. J Fish Biol. 84:106–132. doi: 10.1111/jfb.12258
- Gomon M. 2001. Documenting change. In: Rasmussen C, editor. A museum for the people: a history of Museum Victoria and its predecessor institutions, 1854–2000. Museum Victoria: Scribe Publications; p. 251.
- Green KM, Starr RM. 2011. Movements of small adult black rockfish: implications for the design of MPAs. Mar Ecol-Prog Ser. 14:219–230. doi:10.3354/meps09263
- Harvey ES, Butler JJ, McLean DL, Shand J. 2012. Contrasting habitat use of diurnal and nocturnal fish assemblages in temperate Western Australia. J Exp Mar Biol Ecol. 426–427:78–86. doi: 10.1016/j.jembe.2012.05.019
- Heupel MR, Semmens JM, Hobday AJ. 2006. Automated acoustic tracking of aquatic animals: scales, design and deployment of listening station arrays. Mar Freshwater Res. 57:1–13. doi: 10.1071/MF05091
- Jadot C, Donnay A, Acolas ML, Cornet Y, Bégout Anras ML. 2006. Activity patterns, home-range size, and habitat utilization of Sarpa salpa (Teleostei: Sparidae) in the Mediterranean Sea. ICES J Mar Sci. 63:128–139. doi: 10.1016/j.icesjms.2005.06.010
- Kramer DL, Chapman MR. 1999. Implications of fish home range size and relocation for marine reserve function. Environ Biol Fish. 55:65–79. doi: 10.1023/A:1007481206399
- Kuiter RH. 1999. Guide to sea fishes of Australia: a comprehensive reference for divers and fishermen. Sydney: New Holland Publishers (Australia) Pty Ltd. 434 p.
- Lowry MB, Suthers IM. 1998. Home range, activity and distribution patterns of a temperate rocky-reef fish, Cheilodactylus fuscus. Mar Biol. 132:569–578. doi: 10.1007/s002270050422
- Moore SE, Hesp SA, Hall NG, Potter IC. 2007. Age and size compositions, growth and reproductive biology of the breaksea cod Epinephelides armatus, a gonochoristic serranid. J Fish Biol. 71:1407–1429. doi: 10.1111/j.1095-8649.2007.01614.x
- Payne NL, Gillanders BM, Webber DM, Semmens JM. 2010. Interpreting diel activity patterns from acoustic telemetry: the need for controls. Mar Ecol-Prog Ser. 419:295–301. doi: 10.3354/meps08864
- Roberts CD, Gomon MF. 2008. Family Serranidae: sea basses, gropers, seaperches, cods. In: Gomon M, Bray D, Kuiter R, editors. Fishes of Australia’s southern coast. Sydney: Reed New Holland; p. 535–548.
- Samoilys MA. 1997a. Movement in a large predatory fish: coral trout, Plectropomus leopardus (Pisces: Serranidae), on Heron Reef, Australia. Coral Reefs. 16:151–158. doi: 10.1007/s003380050069
- Samoilys MA. 1997b. Periodicity of spawning aggregations of coral trout Plectropomus leopardus (Pisces: Serranidae) on the northern Great Barrier Reef. Mar Ecol-Prog Ser. 160:149–159. doi: 10.3354/meps160149
- Saunders RJ, Bryars SR, Fowler AJ. 2010. Preliminary consideration of the biology of several of South Australia’s marine fish species that have conservation or management interest. Report to the Department for Environment and Heritage. South Australian Research and Development Institute (Aquatic Sciences), Adelaide, SARDI Publication Number F2009/000693-1. SARDI Research Report Series No. 426. 41 p.
- Shepherd SA, Baker JL. 2008. Reef fishes of lower Gulf St Vincent. In: Shepherd SA, Bryars S, Kirkegaard I, Harbison P, Jennings JT, editors. Natural history of Gulf St Vincent. Adelaide: Royal Society of South Australia; p. 297–316.
- Shepherd SA, Brook JB, Xiao Y. 2009. Environmental and fishing effects on the abundance, size and sex ratio of the blue-throated wrasse, Notolabrus tetricus, on South Australian coastal reefs. Fisheries Manag Ecol. 2009:1–12.
- Shepherd SA, Teale J, Muirhead D. 2005. Cleaning symbiosis among inshore fishes at Althorpe Island, South Australia and elsewhere. T Roy Soc South Aust. 129:193–201.
- Tolimieri N, Andrews K, Williams G, Katz S, Levin PS. 2009. Home range size and patterns of space use by lingcod, copper rockfish and quillback rockfish in relation to diel and tidal cycles. Mar Ecol-Prog Ser. 380:229–243. doi: 10.3354/meps07930
- Topping DT, Lowe CG, Caselle JE. 2005. Home range and habitat utilization of adult California sheephead, Semicossyphus pulcher (Labridae), in a temperate no-take marine reserve. Mar Biol. 147:301–311. doi: 10.1007/s00227-005-1573-1