ABSTRACT
Body mass and temperature are primary determinants of metabolic rate in ectothermic animals. Oxygen consumption of post-larval Galaxias maculatus was measured in respirometry trials under different temperatures (5–21 °C) and varying body masses (0.1–>1.5 g) spanning a relevant range of thermal conditions and sizes. Specific respiration rates (R in g O2 g−1 d−1) declined as a power function of body mass and increased exponentially with temperature and was expressed as: R = 0.0007 * W −0.31 * e 0.13 * T. The ability of this model to predict specific respiration rate was evaluated by comparing observed values with those predicted by the model. Our findings suggest that the respiration rate of G. maculatus is the result of multiple interactive processes (intrinsic and extrinsic factors) that modulate each other in ‘meta-mechanistic’ ways; this would help to explain the species’ ability to undergo the complex ontogenetic habitat shifts observed in the lakes of the Andean Patagonic range.
Introduction
Bioenergetic models offer an effective framework for examining processes that regulate trophic interactions and growth of fish populations, allowing both quantitative and predictive analysis of factors influencing the structure and dynamics of predator and prey populations (Brandt & Hartman Citation1993; Ney Citation1993). In Argentinian Patagonia, bioenergetic models have been used to analyse ecological impacts of exotic salmonids in lakes (Vigliano et al. Citation2009; Juncos et al. Citation2011, Citation2013, Citation2015). Fully parameterised bioenergetic models for native Patagonian fishes do not currently exist, but would be extremely useful tools to address many of the emerging issues facing these species and their communities such as response to climate change, estimation of carrying capacity, and bioenergetic consequences of alternative life history strategies.
Galaxias maculatus (Jenyns, 1842) is a species with a wide circumpolar distribution, exhibiting diadromous and landlocked life history patterns (Cussac et al. Citation2004). The species plays an important role in trophic coupling across freshwater–estuarine–marine, pelagic–benthic and lotic systems in temperate regions of the southern hemisphere (McDowall et al. Citation1994; Cussac et al. Citation2004). In South America, this species expresses both life history strategies, constituting an important component of native aquatic communities (Pascual et al. Citation2002; Cussac et al. Citation2004). Landlocked populations of G. maculatus in Argentinian Patagonia show ontogenetic movements as well as diel horizontal and vertical movements within lakes (Cussac et al. Citation1992; Rechencq et al. Citation2011, Citation2014; Milano et al. Citation2013). Ontogenetic habitat shifts occur as individuals achieve a threshold size of approximately 28 mm total length, metamorphosing and shifting from pelagic to littoral habitats (Barriga et al. Citation2002) and gradually shifting from a planktonic–littoral to a littoral–benthic diet (Cervellini et al. Citation1993). In addition, diel movements to avoid predators and improve feeding (Milano et al. Citation2013) expose fish to varying water temperatures. Both types of movements are considered key processes in habitat coupling of Patagonian Andean lakes (Milano et al. Citation2013), where this species plays an important role in linking lower and upper trophic levels (Modenutti et al. Citation1993; Macchi et al. Citation1999). Larval galaxiids are the primary link between plankton and piscivorous fishes, and the post-larval ontogenetic habitat shift links the pelagic and benthic habitats (Vigliano et al. Citation2009; Juncos et al. Citation2011, Citation2013, Citation2015; Reissig et al. Citation2015). Consequently, for many Andean lakes in Patagonia, G. maculatus serves as a keystone prey species responsible for maintaining food web stability in the face of salmonid introductions (Juncos et al. Citation2013).
Due to the importance of G. maculatus in Patagonia, developing a bioenergetic modelling approach would enhance understanding of how changing thermal conditions and body sizes affect feeding, growth, distribution and habitat use by G. maculatus and thus influence their ontogenetic shifts in energy–matter transfers between trophic levels and habitats. Given the important effects of body mass (Randall et al. Citation2002) and environmental temperature on metabolic rate (Jobling Citation1995) and potential interactions between these factors, a unified approach that accounts for the combined effects of both factors is needed to understand how ontogeny and environmental variability affect growth and trophic interactions of this key species. Therefore, the objective for this paper was to parameterise a respiration function corresponding to the Wisconsin-type bioenergetics modelling framework (Kitchell et al. Citation1977; Hanson et al. Citation1997) by estimating mass-specific rates of respiration (R) of G. maculatus as functions of body mass and water temperature.
Materials and methods
Galaxias maculatus were collected using baited traps from Moreno Lake (41°80′50″S, 71°83′20″W; near San Carlos de Bariloche city, Nahuel Huapi National Park, Río Negro Province, Argentina). Post-larval fish were collected at different times of the year at different sizes (37–79 mm, 0.12–2.88 g; ) as needed for the experiments, avoiding individuals in reproductive status. Collected fish were transported immediately to the laboratory in aerated freshwater tanks. In the laboratory, fish were gradually acclimatised to experimental temperatures by 1 °C per day for up to 1 week, depending on the difference between the temperature at capture (9.5–17.9 °C) and the target experimental temperatures (5–21 °C; ), after which fish were maintained at a constant target temperature and photoperiod (13 h light/11 h dark) until used in the trials. Fish were fed maintenance rations of live food (i.e. mosquito larvae; cladocerans; copepods).
Table 1. Body mass (g, mean ± SD) , specific metabolic rate (g O2 g–1 d–1, mean ± SD) and fish number of Galaxias maculatus at different test temperatures and body masses.
For respirometry trials, we used an acrylic closed chamber (diameter 9 cm, length 18 cm, volume 1.1 L) in a controlled temperature incubator. These respirometers have been used successfully for this species in previous studies (Milano et al. Citation2010, Citation2013). Each trial was conducted with an individual G. maculatus deprived of food for 24 h prior to the test; each fish was used only once. The chamber was completely filled with water from the same source and under identical temperature conditions as used in the acclimation aquarium. Oxygen concentrations and temperatures were recorded continuously during the experiments at 1 s intervals using a digital oxygen meter (YSI Model 5000) connected to the respirometry chamber. Data were transferred to a computer via HyperTerminal (RS232 serial port). The oxygen-measuring probe (Pro-BOD Probe) mixed the water with a stirring paddle. No water was exchanged during the experiment. To reduce the effect of handling stress from these trials, oxygen concentration was measured for 25 h, eliminating the first hour of data from the analysis (Milano et al. Citation2013). Dissolved oxygen concentrations were not allowed to drop below 5 mg L–1 during the trials. The respiration trials were conducted as a factorial combination of five temperatures: 5, 9, 13, 17 and 21 °C and body masses (range: 0.12–2.88 g) binned into size classes of 0.1 g, 0.5 g, 1.0 g and >1.5 g ().
Specific rates of respiration (R) were estimated from the slope of dissolved oxygen versus time multiplied by the volume of water in the chamber and divided by the body mass of the fish, expressed as g (O2) g (fish)−1 d−1. Using the naming convention for parameters in the Wisconsin Bioenergetics Model (Hanson et al. Citation1997), the effect of body mass on R at a given water temperature was described by the allometric function: R = RA WRB. The effect of body mass and temperature on R was modelled using the basic form of the respiration function R = RA WRB e (RQ T) (equation 1 in Hanson et al. Citation1997; Stewart et al. Citation1983), where R is the specific rate of respiration (g O2 g−1 d−1), RA is the intercept of the allometric mass function (g O2 g−1 d−1), W is body mass (g), RB is the slope of the allometric mass function, RQ is the exponential coefficient for the temperature dependence function, and T is water temperature (°C).
Simple linear regressions were used to estimate RA and RB coefficients for each temperature separately using the log-transformed data of R and W. In order to account for the effect of T, a multiple linear regression analysis was used to estimate the corresponding RA, RB and RQ coefficients. An analysis of covariance (ANCOVA) was used to test homogeneity of the slope of the linear relationship among R and T (with W as a covariate). This equation was tested through the absence of significant interaction between T and W, after checking that the assumptions of linearity and independence between W and T were met.
The model was further evaluated by comparing the observed experimental values with predicted values by the model. Following Mayer and Buttler (Citation1993) we evaluated the model through: the mean absolute percent error (E% = 100 [Σ(|yo − yp| /|yo|)]/n, where yo represented observed values, yp predicted values and n the number of pairs); the modelling efficiency (EF = 1 − Σ(yo − yp)2/ Σ(yo − ym)2, where yo and yp were the same as described previously, and ym was the mean of observed values); the coefficient (r2) of the linear regression between observed and predicted values; and through testing of the deviation of the slope (b) from 1 by the F-test (Harrison Citation1990) and of the intercept (a) of the regression line from 0 by the t-test.
Results
The specific respiration rate of G. maculatus declined with body mass at most temperatures, but this allometric relationship weakened at the highest temperature (; ). For a particular T, the exponent RB (in the equation R = RA WRB) was −0.32, −0.32, −0.34 and −0.25 at 5, 9, 13 and 17 °C, respectively (). However, both the value and fit of RB declined rapidly at 21 °C (RB = −0.10) and no statistically significant relationship with W was observed at 21 °C (). Given this lack of linearity, we excluded the 21 °C data from the analysis. There were no significant differences among the RB values at different T (i.e. 5–17°C), as demonstrated by the lack of significant interaction between T and W (ANCOVA, F3,46 = 0.175; P = 0.913). This shows that the relationship of specific respiration rate of G. maculatus with W is uniform between 5 and 17 °C. The specific respiration rate increased significantly as a function of temperature and decreased as a function of body mass (). Estimation of the RA, RB, and RQ through multiple linear regression yielded the following specific respiration rate equation (R2 = 0.87; n = 54; P < 0.0001; ):
Figure 1. Relationships between the log-specific respiration rate and log-body mass (W, g) of Galaxias maculatus at different temperatures (T, °C). Plotted lines represent best-fit regressions for each temperature.
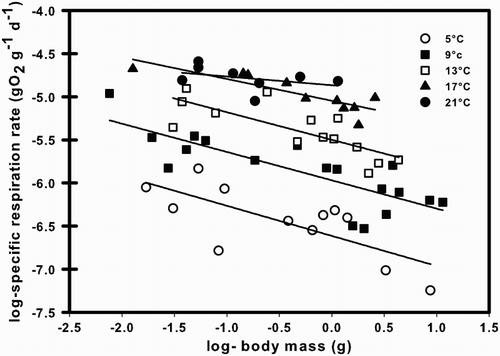
Figure 2. Relationship between the specific respiration rate and temperature (T, °C) of Galaxias maculatus at different body masses (W, g). Plotted lines represent best-fit regressions for each body mass.
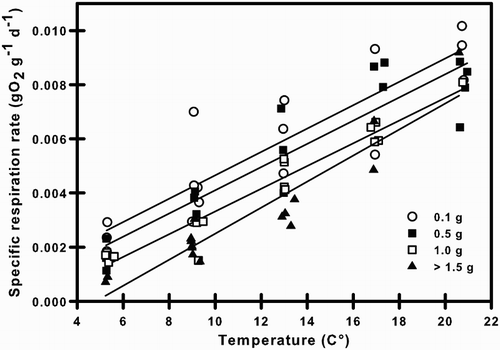
Figure 3. Specific respiration rates under the combined effects of body mass and temperature of Galaxias maculatus. model for fitted curves at varying temperatures. Plotted lines represent best-fit regressions for each temperature.
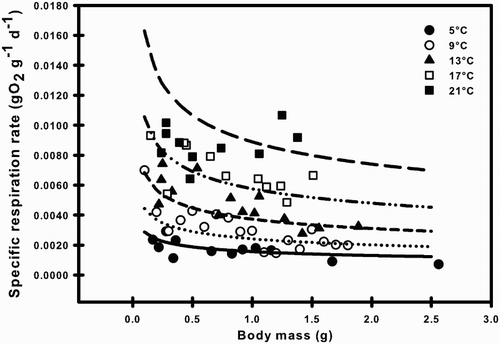
Table 2. Predictive models of specific respiration rate (R, g O2 g–1 d–1) of Galaxias maculatus using fish body mass (W, g) and water temperature (T, °C) as explicative variables (coefficient estimation ± SD).
The ability of the model to predict specific respiration rate was evaluated by comparing the observed experimental values with those predicted by the model (between 5 and 17°C). The comparison between observed and predicted specific respiration rates under experimental conditions resulted in the mean absolute percentage errors of (E%) = 18.01%, a modelling efficiency (EF) = 0.85 and a R2 = 0.86 (). The intercept a = 0.0004 (±0.0002) of the linear regression did not differ significantly from 0 (t-test; P = 0.061) and slope b = 0.95 (±0.052) did not differ significantly from 1 (F-test; P > 0.05).
Discussion
This is the first study in which the specific respiration rate of G. maculatus has been tested under the combined effects of body mass and temperature, across a relevant size range of juveniles and adults (0.1 to >1.5 g) and a broad temperature range (5–21 °C). The specific respiration rate of G. maculatus followed the expected declining power function with body mass and exponential increase with temperature. The model fit the experimental data well for all but the warmest temperature (21 °C). Although the model performed well when evaluated for model efficiency, slope and intercept (Harrison Citation1990; Mayer & Buttler Citation1993), a true corroboration of the model against independent data has not been conducted due to uncertainties associated with limitations or comparability of other independent data sets.
The specific respiration rates of G. maculatus obtained in this study ranged from 0.0017–0.0087 g O2 g−1 d−1 and were in agreement with values produced over more limited ranges of temperatures or body masses (Encina-Montoya et al. Citation2011; Milano et al. Citation2013; Urbina & Glover Citation2013). In previous studies, the respiration rate for G. maculatus had been estimated for fixed temperatures and varying masses. Ríos (Citation1979) determined the allometric relationship between oxygen consumption and body size (on the order on 0.1–2 g) at 15 °C for lake and river populations of G. maculatus as R (mL O2 h−1) = 0.25 W 0.61 (lake) and R = 0.269 W 0.60 (river), finding no significant differences between the slopes for both populations. More recently, Encina-Montoya et al. (Citation2011) reported b = 0.612 for G. maculatus at 12 °C (0.02–1.5 g), whereas Urbina & Glover (Citation2013) reported b = 0.82 at 14 °C (0.14–11.28 g). Our estimate of RB was −0.31 when fit simultaneously to body mass and temperature. After converting RB in this study from a specific rate (g O2 g−1 d−1) to an absolute rate by adding 1.0, a value of 0.69 (g O2 d−1) was produced in units comparable to the other studies. The allometric slope from our study, while accounting for a broad range of temperatures and an ecologically relevant range of body masses, was similar to two of the three studies (Ríos Citation1979; Encina-Montoya et al. Citation2011) conducted at single temperatures, but deviated more from the study that included a much broader range of body masses, including extremely large individuals (Urbina & Glover Citation2013). While this potential shift in the allometry of respiration associated with larger sizes deserves further attention, most G. maculatus landlocked populations are predominantly composed of sizes approximately 80 mm (2–3 g) or smaller. Therefore, we believe our model can reasonably represent the generalised respiration relationships for the ecologically significant fraction of post-larval G. maculatus.
As a mobile consumer with distinct ontogenetic shifts in diet and habitat use, G. maculatus provides crucial links among production pathways of littoral, benthic and pelagic zones (Cussac et al. Citation1992; Barriga et al. Citation2002; Rechencq et al. Citation2011, Citation2014; Milano et al. Citation2013). The ability to quantify their metabolic and trophic responses to changing environmental conditions will advance our understanding of habitat coupling processes in Patagonian oligotrophic lakes. Accurate parameterisation of mass- and temperature-dependent effects on metabolic rates as presented here can be combined with mass- and temperature-dependent functions for maximum consumption and waste losses functions to construct a full bioenergetics model for the species. Such a model will facilitate a clearer understanding of the role of G. maculatus as both a mobile consumer and prey in varying habitats and seasonally fluctuating temperatures. This capability will be critically important for addressing local and regional management issues related both to short- and long-term human impacts such as urban development and global warming.
Acknowledgements
We want to thank M Beveraggi for allowing access to the lake through her property. Any use of trade, firm or product names is for descriptive purposes only and does not imply endorsement by the US Government.
Associate Editor: Dr Joanne Clapcot.
Disclosure statement
No potential conflict of interest was reported by the authors.
Additional information
Funding
References
- Barriga JP, Battini MA, Macchi PJ, Milano D, Cussac VE. 2002. Spatial and temporal distribution of landlocked Galaxias maculatus and Galaxias platei (Pisces, Galaxiidae) in a lake in the South American Andes. New Zeal J Mar Fresh. 36:349–363. doi: 10.1080/00288330.2002.9517092
- Brandt SB, Hartman KJ. 1993. Innovative approaches with bioenergetics models: future applications to fish ecology and management. Trans Am Fish Soc. 122:731–735. doi: 10.1577/1548-8659(1993)122<0731:IAWBMF>2.3.CO;2
- Cervellini PM, Battini MA, Cussac VE. 1993. Ontogenetic shift in the diet of Galaxias maculatus (Galaxiidae) and Odontesthes microlepidotus (Atheridae). Environ Biol Fish. 36:283–290. doi: 10.1007/BF00001724
- Cussac VE, Cervellini PM, Battini MA. 1992. Intralacustrine movements of Galaxias maculatus (Galaxiidae) and Odontesthes microlepidotus (Atherinidae) during their early life history. Environ Biol Fish. 35:141–148. doi: 10.1007/BF00002189
- Cussac VE, Ortubay S, Iglesias G, Milano D, Lattuca ME, Barriga JP, Battini MA, Gross M. 2004. The distribution of South America galaxiid fishes: the role of biological traits and post-glacial history. J Biogeogr. 31:103–121. doi: 10.1046/j.0305-0270.2003.01000.x
- Encina-Montoya F, Vega-Aguayo R, Mardones-Lazcano A, Rueda T, Tello A. 2011. Characterization of whitebait (Galaxias maculatus) respiratory rates to optimize intensive culture carrying capacities. Aquacult Res. 42:835–843. doi: 10.1111/j.1365-2109.2010.02692.x
- Hanson PC, Johnson TB, Schindler DE, Kitchell JF. 1997. Fish bioenergetics 3.0. University of Wisconsin, Sea Grant Institute, Madison, WI, No. WISCU-T-97-001.
- Harrison SR. 1990. Regression of a model on real-system output: an invalid test of model validity. Agric Syst. 34:183–190. doi: 10.1016/0308-521X(90)90083-3
- Jobling M. 1995. Fish bioenergetic. London: Chapman & Hall.
- Juncos R, Beauchamp DA, Vigliano PH. 2013. Modelling prey consumption by native and nonnative piscivorous fishes: implications for competition and impacts on shared prey in an ultraoligotrophic lake in Patagonia. Trans Am Fish Soc. 142:268–281. doi: 10.1080/00028487.2012.730109
- Juncos R, Milano D, Macchi PJ, Alonso M, Vigliano PH. 2011. Response of rainbow trout (Oncorhynchus mykiss) to different food web structures in Northern Patagonia: implications on growth, bioenergetics and invasiveness. Trans Am Fish Soc. 140:415–428. doi: 10.1080/00028487.2011.572000
- Juncos R, Milano D, Macchi PJ, Vigliano PH. 2015. Niche segregation facilitates coexistence between native and introduced fishes in a deep Patagonian lake. Hydrobiologia. 747:53–67. doi: 10.1007/s10750-014-2122-z
- Kitchell JF, Stewart DJ, Weininger D. 1977. Applications of a bioenergetics model to Yellow Perch (Perca flavescens) and Walleye (Stizostedion vitreum vitreum). J Fish Res. Board Can. 34:1910–1921. doi: 10.1139/f77-257
- Macchi PJ, Cussac VE, Alonso MF, Denegri MA. 1999. Predation relationships between introduced salmonids and the native fish fauna in lakes and reservoirs in Northern Patagonia. Ecol Freshw Fish. 8:227–236. doi: 10.1111/j.1600-0633.1999.tb00074.x
- Mayer DG, Butler DG. 1993. Statistical validation. Ecol Model. 68:21–32. doi: 10.1016/0304-3800(93)90105-2
- McDowall RM, Mitchell CP, Brothers EB. 1994. Age at migration from the sea of juvenile “Galaxias” in New Zealand (Pisces: Galaxiidae). Bull Mar Sci. 54:385–402.
- Milano D, Aigo JC, Macchi PJ. 2013. Diel patterns in space use, food and metabolic activity of Galaxias maculatus (Pisces: Galaxiidae) in the littoral zone of a shallow Patagonian lake. Aquat Ecol. 47:277–290. doi: 10.1007/s10452-013-9443-2
- Milano D, Lozada M, Zagarese HE. 2010. Predator-induced reaction patterns of landlocked Galaxias maculatus to visual and chemical cues. Aquat Ecol. 44:741–748. doi: 10.1007/s10452-010-9312-1
- Modenutti BE, Balseiro EG, Cervellini PM. 1993. Effect of the selective feeding of Galaxias maculatus (Salmoniformes Galaxiidae) on zooplankton of a South Andes lake. Aquat Sci. 55:65–75. doi: 10.1007/BF00877259
- Ney JJ. 1993. Bioenergetics modeling today: growing, pains on the cutting edge. Trans Am Fish Soc. 122:736–748. doi: 10.1577/1548-8659(1993)122<0736:BMTGPO>2.3.CO;2
- Pascual MA, Macchi PJ, Urbanski J, Marcos F, Riva Rossi C, Novara M, Dell'Arciprete P. 2002. Evaluating potencial effects of exotic freshwater fish from incomplete species presence-absence data. Biol Invasions. 4:101–113. doi: 10.1023/A:1020513525528
- Randall DJ, Burggren WW, French K, Eckert R. 2002. Animal physiology: mechanisms and adaptations. New York (NY): W.H. Freeman and Co.
- Rechencq M, Sosnovsky A, Macchi PJ, Alvear PA, Vigliano PH. 2011. Extensive diel fish migrations in a deep ultraoligotrophic lake of Patagonia Argentina. Hydrobiologia. 658:147–161. doi: 10.1007/s10750-010-0458-6
- Rechencq M, Vigliano PH, Macchi PJ, Lippolt GE. 2014. Fish distribution patterns and habitat availability in lakes Moreno Este and Moreno Oeste, Patagonia, Argentina. Limnologica. 49:73–83. doi: 10.1016/j.limno.2014.09.003
- Reissig M, Queimaliños C, Modenutti B, Balseiro E. 2015. Prey C:P ratio and phosphorus recycling by a planktivorous fish: advantages of fish selection towards pelagic cladocerans. Ecol Freshw Fish. 24:214–224. doi: 10.1111/eff.12136
- Ríos CF. 1979. Balance energético en poblaciones de Galaxias maculatus Jenyns (Salmoniformes: Galaxiidae). Medio Ambiente. 4:24–39.
- Stewart DJ, Weininger D, Rottiers DV, Edsall TA. 1983. An energetics model for Lake Trout, Salvelinus namaycush: application to the Lake Michigan population. Can J Fish Aquat Sci. 40:681–698. doi: 10.1139/f83-091
- Urbina MA, Glover CN. 2013. Relationship between fish size and metabolic rate in the oxyconforming inanga Galaxias maculatus reveals size-dependent strategies to withstand hypoxia. Physiol Biochem Zool. 86:740–749. doi: 10.1086/673727
- Vigliano PH, Beauchamp DA, Milano D, Macchi PJ, Alonso MF, García Asorey M, Denegri MA, Ciancio JE, Lippolt G, Rechencq M, Barriga JP. 2009. Quantifying predation on galaxiids and other native organisms by introduced rainbow trout in an ultraoligotrophic lake in northern Patagonia, Argentina: a bioenergetics modeling approach. Trans Am Fish Soc. 138:1405–1419. doi: 10.1577/T08-067.1