ABSTRACT
Genes encoded in nuclear ribosomal DNA (nrDNA), which comprise a multi-family of genes with tandem repeat units, play an important role in nucleolus formation. In the present study, we sequenced and analysed the complete rDNA sequence of an economically important shellfish in Australia, Haliotis rubra. The single rDNA unit measured 9881 base pairs (bp) in length and included the following elements in order: 18S rRNA (1858 bp), internal transcribed spacers (ITS, 756 bp), 28S rRNA (3413 bp) and an intergenic spacer (IGS, 3854 bp). The phylogenetic tree was constructed using abalone ITS sequences; here, the relationship revealed that abalone species within the same region and with same chromosome number tended to cluster within a clade. The positions of putative transcription termination and RNA transcription initiation sites were predicted in the IGS region. A comparative sequence complexity analysis and dot plots revealed a high level of variability in the IGS.
Introduction
The blacklip abalone Haliotis rubra (Gastropoda, Mollusca) is a highly valuable marine shellfish species endemic to Australia (Baranski et al. Citation2008). The production of this most abundant wild fishery abalone species has exceeded 3500 tons per year (i.e. 82% of abalone production in Australia), with a net value of US$79 million (Mayfield et al. Citation2012; Miller et al. Citation2014; Miller et al. Citation2016). However, the wild H. rubra population has recently suffered a major decline attributed to overfishing (Valentine et al. Citation2010; Liu et al. Citation2015), disease (Hooper et al. Citation2007; Dang et al. Citation2011; Corbeil et al. Citation2016) and predators (Ling Citation2008; Pederson et al. Citation2008). Three strategies have been proposed to restock these depleted wild stocks: control of the yield at a reduced level, reductions in fishing efforts, and an increased size at first capture (Sanders and Beinssen Citation1998; Mayfield et al. Citation2012). Meanwhile, the field of abalone aquaculture has developed and expanded rapidly in Australia over the last decade (Goodsell et al. Citation2006). To further improve the productivity and sustainability of the abalone industry, genetic improvement techniques such as triploidy (Liu et al. Citation2006, Citation2009) and hybridisation (Botwright Citation2015; Alter et al. Citation2017) have been evaluated, and the subsequent application of the latter might lead to germplasm confusion and contamination of the H. rubra population. Accordingly, the development of reasonable H. rubra germplasm conservation and management strategies is extremely urgent and requires a deeper understanding of molecular genetics. Previous molecular studies of H. rubra have focused on the population structure (Conod et al. Citation2002; Li et al. Citation2006; Appleyard et al. Citation2009; Miller et al. Citation2016), linkage map construction (Baranski et al. Citation2006), QTL (quantitative trait locus) growth rate (Baranski et al. Citation2008) and mitochondrial analysis (Maynard et al. Citation2005).
Nuclear ribosomal DNA (nrDNA) is the genomic region in which the RNA components of ribosomes are encoded (Ki et al. Citation2009). Eukaryotic nrDNA comprises a multigene family including transcribable rRNA genes (18S rRNA, 28S rRNA and 5.8S rRNA) separated by internal transcribed spacers (ITS1 and ITS2) and an intergenic spacer (IGS) that are located downstream of the 18S rRNA gene and upstream of 28S rRNA gene (). These genes cluster in large tandems located on certain chromosomes to form nucleolus organising regions (NORs) (Dyomin et al. Citation2016). Although the rDNA present concerted evolution, each region of rDNA units evolves at a different rate. During evolution, the coding regions (18S and 28S rRNA) have remained more conserved than the non-coding regions (ITS and IGS), and nrDNA regions have always been used as molecular markers in phylogenetic analyses (Li et al. Citation2016). Complete nrDNA sequences provide us with various options, depending on the molecular variability. To date, complete nrDNA research has been conducted in the black-footed abalone (Guo et al. Citation2017), pacific abalone Haliotis discus hannai (Guo et al. Citation2017), moon jelly Aurelia sp.1 (Ki et al. Citation2009), Chinese mitten crab Eriocheir sinensis (Yu et al. Citation2010), Paramphistomum cervi (Zheng et al. Citation2014), Beta (Santoni and Bervillé Citation1992), alga Pyropia yezoensis (Li et al. Citation2016), Bangia (Xu et al. Citation2016) and Pyropia haitanensis (He et al. Citation2017). However, neither the complete structure nor each region of nrDNA has been reported before except ITS1-5.8S region (GenBank accession number: AF296867) conducted by Coleman and Vacquier (Citation2002).
The present study aimed to conduct the first amplification and analysis of the complete nrDNA sequence of H. rubra, to characterise the features of each region, and to explore the phylogenetic relationship between H. rubra and other abalone species. The findings are expected to provide additional data for the classification, identification, phylogenic analysis, germplasm management and conservation of Haliotis species.
Materials and methods
Sampling
One blacklip abalone collected from Port Lincoln, South Australia (34°73′ S, 135°85′ E) was used for the nrDNA sequence analysis. The foot muscle of the organism was extracted, transferred to 75% alcohol, and stored at −20°C for subsequent DNA isolation.
DNA extraction, PCR amplification and sequencing
Genomic DNA was isolated using the TIANamp Marine Animals DNA Kit (TianGen Biotech Co., Ltd, Beijing, China) according to the manufacturer’s instructions. Each nrDNA region was amplified using the primers described by Guo et al. (Citation2017). The primer sequences are listed in . The PCR reaction volume was 25 μl, including 2 μl of DNA template, 0.5 μl of each primer (20 μmol/l), 12.5 μl of 2 × Tran Taq High Fidelity (HiFi) PCR SuperMix (TransGen Biotech Co., Ltd) and 9.5 μl of ddH2O. The PCR amplification conditions were as follows: initial denaturation at 95°C for 5 min, and 35 cycles of denaturation at 95°C for 1 min, annealing at 52–58°C for 30–60 s, and extension 72°C for 1–5 min (). The PCR products were visualised as single bands via electrophoresis on 1% agarose gels and subsequently cloned using the specific method reported by Guo et al. (Citation2017). Six positive recombinant colonies per amplification product were picked and sequenced by Sangon Biotech Co., Ltd. (Shanghai, China).
Table 1. Primers and polymerase chain reaction protocols used in this study.
Sequence analysis
The sequences of complete nrDNA and each region were assembled into contigs using DNAMAN software (Feng et al. Citation2014). The boundaries of each region were confirmed by comparison with data from the NCBI GenBank database using BLAST software. The sequence alignment was performed using T-Coffee software (Notredame et al. Citation2000), and manually adjusted using BioEdit (Li et al. Citation2009). The general molecular features of H. rubra rDNA were calculated by MEGA 6.0 (Tamura et al. Citation2013). The nucleic acid distribution, sequence complexity and entropy across all H. rubra nrDNA nucleotides were calculated using the BioAnnotator in Vector NTI Advance 11.5.1 (Ki et al. Citation2009). A dot plot analysis was performed using MegAlign 5.01 software (DNAstar) (Jang et al. Citation2014). The NCBI GenBank was searched for the 18S, ITS, 28S and IGS sequence information of other abalone species, and the details were shown in . The pairwise distances of 18S, 28S rRNA and IGS sequences in other abalone species were calculated using MEGA 6.0 according to the Kimura 2-parameter model. A phylogenetic tree based on the ITS sequences was constructed using the maximum likelihood (ML) method and PHYML software (Guindon et al. Citation2010). The phylogenetic analysis was tested using the bootstrap method with 1000 bootstrap replications, and jmodeltest 0.1 software (Posada Citation2009) was used to confirm the best substitution model as ‘GTR + G’. Repeat fragments of the IGS region were analysed using Tandem Repeats Finder (Benson Citation1999).
Table 2. Collected information and GenBank accession numbers for abalone species used in this study.
Results
General features of the complete rDNA unit
Each region of nrDNA was successfully amplified and sequenced, and the boundaries of ribosomal genes were confirmed after assembling and blasting the sequences in the NCBI GenBank database. The complete nrDNA sequence had a length of 9881 base pairs (bp) and exhibited the following typical eukaryotic rDNA organisation: 18S, 1858 bp – ITS, 756 bp (comprising ITS1, 327 bp – 5.8S, 132 bp – ITS2, 297 bp)–28S, 3413 bp–IGS, 3854 bp. The nucleotide contents of each region are listed in . The complete nrDNA contained the following nucleotide distribution: A, 23.9%; T, 25.3%; C, 22.7% and G, 28.0%. The GC content of the IGS, at 46.5%, was lower than that of other genes (50.5–58.7%). The GenBank accession numbers of the newly acquired sequences are shown in .
Table 3. Nucleotide composition, length, and GenBank accession number of each rRNA region from H. rubra.
18S and 28S rRNA
The lengths of H. rubra 18S and 28S rRNA were confirmed through a comparison with the sequences of H. discus hannai and H. iris. The GenBank includes 18S and 28S rRNA records for 14 and 8 abalone species, respectively. Among the existing records for abalones, close pairwise distances were observed for 18S (0.000–0.010), whereas more sequence divergence was observed in the 28S region (0.005–0.042) as shown in . H. rubra and H. discus exhibited the maximum genetic distance (0.007) in 18S whereas H. rubra and H. diversicolor exhibited the maximum genetic distance (0.029) in 28S, which were both <0.1.
Table 4. Pairwise distances of 18S sequences (lower left matrix) and 28S rDNA sequences (upper right matrix).
ITS
The 5.8S gene is short and conserved and is therefore rarely used by itself. Instead, it is combined with the ITS1 and ITS2 genes to compose the ITS region. ITS sequences have been recorded for 24 abalone species. A ML phylogenetic tree was constructed based on these ITS sequences to reveal the evolutionary relationships among Haliotidae (). This tree diverged into two main clades, in which the Australian species H. rubra, H. scalaris, H. roei and H. cyclobates clustered together with a bootstrap value of 85%.
IGS
The length of the IGS region was confirmed through comparison with 3′ of 28S and 5′ of 18S, which yielded a length of 3854 bp (). The IGS sequence included three regions: the non-repetitive segment N1 (bp 1–899); the repetitive midsection R (bp 900–1294 bp); and the non-repetitive segment N2, located downstream of the repeat arrays and 5′ of the 18S rRNA coding region (bp 1295–3854). The tandem arrays in the R-region were further dissected into two repeat types: type-I, a 40-bp length containing seven tandem copies (bp 900–1179) which are imperfect tandem copies, and type-II, a 30-bp length containing 4.8 tandem copies (bp 1151–1294 bp) which are perfect tandem copies, in which positions 1151–1179 belong to two repeat types. The IGS region comprises three components, 3′ ETS, NTS and 5′ ETS, arranged in that order. A poly(T) tract (TTTCTTTTTTTTT) was detected at position 466–478, and was considered a putative transcription termination site. Therefore, the position of the 3′ ETS sequence was located at bp 1–478 of the 5′ IGS. Next, we predicted the RNA polymerase I transcription promoter using the Promoter 2.0 Prediction Server (Knudsen Citation1999), and identified a single sequence (GGGCGGG) at bp 3142–3148 of IGS. According to Maggini et al. (Citation2008), ‘GGGNGGG’ is the common zone, and the +1 position of the transcription initiation site is the A nucleotide that preceding maybe the G residues at position 3138. Accordingly, the putative position of the 5′ ETS was located at bp 3138–3854. The remainder of the IGS comprised the NTS (bp 479–3137). The lengths of the 3′ ETS, NTS and 5′ ETS regions were 478, 2659 and 717 bp, respectively. Currently, complete IGS sequences are available for three abalone species (H. discus hannai, H. iris and H. rubra), and the genetic distances between H. rubra and H. discus hannai, between H. rubra and H. iris, and between H. discus hannai and H. iris were calculated as 0.275, 0.282 and 0.217, respectively.
Figure 3. The entire nucleotide sequence of the H. rubra rDNA IGS region. The repeated sequences are underlined as follows: single line for repeat type A and double line for repeat type B. A putative termination signal (poly(T) tract) is in the solid box, and the RNA polymerase I transcription promoter is in the dashed box.
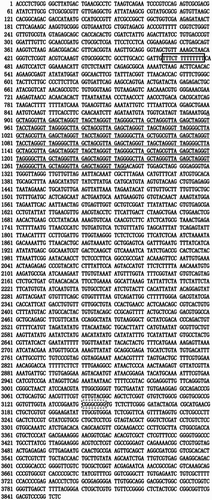
Nucleic acid distribution, sequence complexity, and dot-matrix analysis of H. rubra
We constructed a map of the H. rubra nrDNA organisation and molecular characteristics, including the GC content (%), nucleic acid (T) distribution (%), and sequence complexity, using sliding windows of 80 nucleotides (). Although the GC content distribution varied around 50% across the complete rDNA sequence, the IGS region, and especially the zones of bp 1330–2097 and 2652–2974, had low GC contents and higher T contents relative to other regions. The sequence complexity values fluctuated slightly, with no relatively large differences among rDNA regions.
Figure 4. The GC content (%), nucleic acid distribution (% T), and sequence complexity determined using 80-bp windows across the entire rDNA nucleotide sequence of H. rubra.
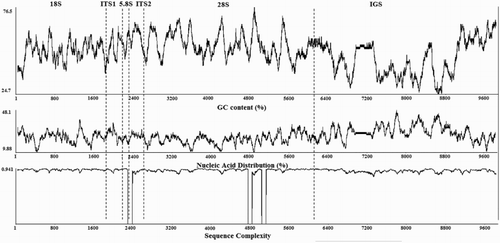
We finally compared the reported complete rDNA sequences for H. discus hannai and H. iris to that of H. rubra through a dot-matrix analysis. The dots and lines in represent the regions of homology between sequence pairs. We observed high levels of similarity among the complete rDNA sequences at the 18S, ITS and 28S regions, whereas the main differences were observed in the IGS region.
Discussion
In the present study, the sequencing of the complete nrDNA of H. rubra for the first time revealed that the order of genes is 18S–ITS1–5.8S–ITS2–28S–IGS, which is identical to the sequences of typical eukaryote rDNA. The complete H. rubra rDNA was 9881 bp in length, which is shorter than that of H. discus hannai (Guo et al. Citation2017), chicken (Dyomin et al. Citation2016) or Bangia (Xu et al. Citation2016) but longer than that of H. iris (Guo et al. Citation2017) or Aurelia (Ki et al. Citation2009). The main differences among species were observed in the IGS gene length.
The degree of conservation of each rDNA domain varied due to different selective pressures on the domains. The highly conserved coding regions are always used in phylogenetic studies of orders and families, whereas the moderately variable ITS region is used mainly for the identification of species and varieties. The IGS domain, which exhibited the most rapid evolution, is always applied to analyses of genetic diversity within species (Li et al. Citation2014). As shown in , the pairwise distances of the 18S and 28S regions among the abalone species were all <0.03, whereas in the IGS region, values were exceeded 0.2 and could be used to distinguish species. These results also support the features of rDNA evolution.
A comparison of our findings with the ITS1 sequence studied by Coleman and Vacquier (Citation2002) (AF296867) revealed 99.39% sequence identity, with a difference of only two bases. The ML phylogenetic tree constructed based on the ITS database was similar to that reported by Guo et al. (Citation2017). Specifically, the tree had three main subclades, and H. rubra clustered within the Australian group. While, the tree present two types of H. tuberculata ITS sequences, H. tuberculata tuberculata, H. tuberculata coccinea and H. marmorata are both H. tuberculata subspecies or sister species, which clustered within a group, but have little similarity with H. tuberculata (GenBank accession number: AF296860.1), the result was in accordance with Van Wormhoudt et al. (Citation2011). According to a report by Guo and Hou (Citation2016), the Pacific group and Australian groups exhibited a diploid complement of 2n = 36 chromosomes, in which H. midae and H. diversicolor comprised 36 and 32 chromosomes, respectively. H. tuberculata and H. lamellosa (Europe group) had 28 chromosomes. Furthermore, abalone with the same chromosome number and distributed in the same region tended to cluster within the same clade, thus supporting the Tethys model of evolution originally proposed by Geiger and Groves (Citation1999) and corroborating the latest model predicted by Estes et al. (Citation2005) in which the earliest haliotids had a Tethyan origin. Although these early species eventually became extinct in the New World, the lineages remained detectable in the current-day Mediterranean and eastern tropical seas. These early tropical abalone gave rise to the Southern Hemisphere fauna that led to extant abalone populations in South Africa, Australia and New Zealand and the abalone of the tropical western Pacific. Another lineage subsequently spread north-eastward to occupy the New World in the eastern Pacific. These northeastern Pacific lineages developed from their early ancestors, and New World tropical taxa reappeared before a subsequent division by the Pliocene closure of the Panamic Portal. The temperate North Pacific was then occupied and subsequently divided into western and eastern groups (Estes et al. Citation2005).
The IGS region varies widely in terms of length and sequence between and within species, and even among individuals (Parvaresh et al. Citation2014), as demonstrated in H. discus hannai, H. iris (Guo et al. Citation2017) and yellow perch (Kakou et al. Citation2015). In H. rubra, the IGS region length was 3854 bp, which was shorter than that of H. discus hannai (4624–4654 bp) but longer than that of H. iris (3560–3662 bp). The IGS structure is important, as it regulates the rRNA gene transcription levels via the transcription stop and start signals contained within its the rDNA units (Fernández et al. Citation2000). A poly(T) tract (TTTCTTTTTTTTT) at the beginning of the 5′ of the IGS region was considered a rDNA transcript termination similar, similar to that of Aurelia (Ki et al. Citation2009). We used Promoter 2.0 Prediction Server software to predict the putative RNA polymerase I transcription promoter because of the lack of research on this element and initiation sites in molluscs. The common ‘GGGNGGG’ sequence in the RNA pol I transcription promoter was also identified in Punica granatum (Parvaresh et al. Citation2014) and Olea europaea (Maggini et al. Citation2008). Although we predicted this putative RNA transcription initiation site, in the future, investigations are needed to confirm these predictions and test the specific spacer–promoter functionality in the H. rubra IGS.
As shown in and , we detected two continuous repeat types in the NTS region, with highly consistent GC contents (%) and nucleic acid (T) distributions (%). The lengths of the repeat elements were 40 and 30 bp, with an overlap region at 1151–1179 bp. Our findings differed from the observations in yellow perch (Karkou et al. Citation2015), wherein four repeat regions are separated by five non-repeat regions. A blast of the GenBank dataset did not reveal similarities of these repeat sequences with other known sequences. Many studies have established that the IGS repetitive elements serve as rRNA gene transcription regulatory elements, particularly enhancers that increase the rRNA transcription rate (Yu et al. Citation2010; Karkou et al. Citation2015), and that the lengths of repeat elements are species-specificity (Guo et al. Citation2017).
Among Haliotidae, although the repeat fragments of H. discus hannai, H. iris and H. rubra were both located the upstream of IGS region, which harbour different repeat elements. H. discus hannai exhibited several upstream (710–2847 bp) repeat fragments 6, 12,18, 31, 71, 73 and 195 bp lengths, while that of H. iris were insertions covering 2, 4, 6, 12, 14, 20 and 32 bp within the scope of 1078–1833bp. It indicated that the repeat fragments of three abalones have rarely rules and similarities. In the present study, the IGS region was used to conduct the first analysis of pairwise distances among three abalone species. As the values of both comparisons exceeded 0.2, this region can be used as a classification marker for Haliotis species. The IGS genes of Porphyra haitanensis (Li et al. Citation2010), Daphnia pulex (Ambrose and Crease Citation2011) and Auricularia auricula-judae (Li et al. Citation2014) have been used as genetic markers with which to identify species or analyse evolutionary relationships. More abalone species should be sequenced to clarify the phylogenetic relationships among Haliotidae, and the IGS region should be evaluated to determine whether it yields the same results as ITS.
In conclusion, we determined and described the complete sequence of a single rDNA unit from H. rubra for the first time. The structural organisation of H. rubra nrDNA is similar to that of many other eukaryotes, with an assembled ribosomal unit length of 9881 bp. The phylogenetic relationships among Haliotidae have been determined using ITS sequences. Here, repeat regions that diverged from the known regulatory sequences of other species were detected in the IGS region. H. rubra rDNA therefore provides a structural model of nuclear rDNA for molecular comparisons, particularly among Haliotidae species. Our study findings are expected to pave the way for analyses of mollusc population genetics and evolution.
Disclosure statement
No potential conflict of interest was reported by the authors.
Additional information
Funding
References
- Alter K, Andrewartha SJ, Morash AJ, Clark TD, Hellicar AD, León RI, Elliott NG. 2017. Hybrid abalone are more robust to multi-stressor environments than pure parental species. Aquaculture. 478:25–34. doi: 10.1016/j.aquaculture.2017.04.035
- Ambrose CD, Crease TJ. 2011. Evolution of the nuclear ribosomal DNA intergenic spacer in four species of the Daphnia pulex complex. BMC Genetics. 12:13. doi: 10.1186/1471-2156-12-13
- Appleyard SA, Carr NA, Elliott NG. 2009. Molecular analyses indicate homogenous structure of abalone across morphologically different Haliotis rubra collections in South Australia. Journal of Shellfish Research. 28:609–616. doi: 10.2983/035.028.0325
- Baranski M, Loughnan S, Austin CM, Robinson N. 2006. A microsatellite linkage map of the blacklip abalone, Haliotis rubra. Animal Genetics. 37:563–570. doi: 10.1111/j.1365-2052.2006.01531.x
- Baranski M, Rourke M, Loughnan S, Hayes B, Austin C, Robinson N. 2008. Detection of QTL for growth rate in the blacklip abalone (Haliotis rubra Leach) using selective DNA pooling. Animal Genetics. 39:606–614. doi: 10.1111/j.1365-2052.2008.01773.x
- Benson G. 1999. Tandem repeats finder: a program to analyze DNA sequences. Nucleic Acids Research. 27(2):573–580. doi: 10.1093/nar/27.2.573
- Botwright NA. 2015. Karyotype comparison between Haliotis rubra, H. laevigata and the interspecific hybrid. Aquaculture Research. 46:236–241. doi: 10.1111/are.12156
- Coleman AW, Vacquier VD. 2002. Exploring the phylogenetic utility of ITS sequences for animals: a test case for abalone (Haliotis). Journal of Molecular Evolution. 54:246–257. doi: 10.1007/s00239-001-0006-0
- Conod N, Bartlett JP, Evans BS, Elliott NG. 2002. Comparison of mitochondrial and nuclear DNA analyses of population structure in the blacklip abalone Haliotis rubra Leach. Marine and Freshwater Research. 53:711–718. doi: 10.1071/MF01197
- Corbeil S, Williams LM, McColl KA, Crane MS. 2016. Australian abalone (Haliotis laevigata, H. rubra and H. conicopora) are susceptible to infection by multiple abalone herpesvirus genotypes. Diseases of Aquatic Organisms. 119:101–106. doi: 10.3354/dao02989
- Dang VT, Speck P, Doroudi M, Smith B, Benkendorff K. 2011. Variation in the antiviral and antibacterial activity of abalone Haliotis laevigata, H. rubra and their hybrid in South Australia. Aquaculture. 315:242–249. doi: 10.1016/j.aquaculture.2011.03.005
- Dyomin AG, Koshel EI, Kiselev AM, Saifitdinova AF, Galkina SA, Fukagawa T, Kostareva AA, Gaginskaya ER. 2016. Chicken rRNA gene cluster structure. Plos One. 11(6):e0157464. doi: 10.1371/journal.pone.0157464
- Estes JA, Lindberg DR, Wray C. 2005. Evolution of large body size in abalones (Haliotis): patterns and implications. Paleobiology. 31:591–606. doi: 10.1666/04059.1
- Feng S, Powell SM, Wilson R, Bowman JP. 2014. Extensive gene acquisition in the extremely psychrophilic bacterial species Psychroflexus torquis and the link to sea-ice ecosystem specialism. Genome Biology and Evolution. 6:133–148. doi: 10.1093/gbe/evt209
- Fernández M, Polanco C, Ruiz ML, Pérez De La Vega M. 2000. A comparative study of the structure of the rDNA intergenic spacer of Lens culinaris Medik. and other legume species. Genome. 43:597–603. doi: 10.1139/g00-022
- Geiger DL, Groves LT. 1999. Review of fossil abalone (Gastropoda: Vetigastropoda: Haliotidae) with comparison to recent species. Journal of Paleontology. 73:872–885. doi: 10.1017/S0022336000040713
- Goodsell PJ, Underwood AJ, Chapman MG, Heasman MP. 2006. Seeding small numbers of cultured black-lip abalone (Haliotis rubra Leach) to match natural densities of wild populations. Marine and Freshwater Research. 57:747–756. doi: 10.1071/MF06039
- Guindon S, Dufayard JF, Lefort V, Anisimova M, Hordijk W, Gascuel O. 2010. New algorithms and methods to estimate maximum-likelihood phylogenies: assessing the performance of PhyML 3.0. Systematic Biology. 59:307–321. doi: 10.1093/sysbio/syq010
- Guo ZS, Ding Y, Zhang XH, Hou XG. 2017. Complete nuclear ribosomal DNA sequence analysis of Pacific abalone Haliotis discus hannai. Fisheries Science. 83:777–784. doi: 10.1007/s12562-017-1109-x
- Guo ZS, Hou XG. 2016. Research progress on chromosome in family Haliotidae. Fisheries Science. 35:597–602. (In Chinese).
- Guo ZS, Hou XG, Han L. 2017. Complete nuclear ribosomal DNA (nrDNA) sequence analyses of the black-footed abalone Haliotis iris. New Zealand Journal of Marine and Freshwater Research. 1–15. doi:10.1080/00288330.2017.1363790.
- He Y, Shen SD, Shen ZG. 2017. Cloning and application of the complete nuclear ribosomal DNA (nrDNA) cistron sequence of Pyropia haitanensis (Bangiales, Rhodophyta). Botanica Marina. 60:327–337. doi: 10.1515/bot-2016-0079
- Hooper C, Hardy-Smith P, Handlinger J. 2007. Ganglioneuritis causing high mortalities in farmed Australian abalone (Haliotis laevigata and Haliotis rubra). Australian Veterinary Journal. 85:188–193. doi: 10.1111/j.1751-0813.2007.00155.x
- Jang HM, Erf GF, Rowland KC, Kong BW. 2014. Genome resequencing and bioinformatic analysis of SNP containing candidate genes in the autoimmune vitiligo Smyth line chicken model. BMC Genomics. 15:707. doi: 10.1186/1471-2164-15-707
- Kakou B, Angers B, Glémet H. 2015. Extensive length variation in the ribosomal DNA intergenic spacer of yellow perch (Perca flavescens). Genome. 59:149–158. doi: 10.1139/gen-2015-0114
- Ki JS, Kim IC, Lee JS. 2009. Comparative analysis of nuclear ribosomal DNA from the moon jelly Aurelia sp.1 (Cnidaria: Scyphozoa) with characterizations of the 18S, 28S genes, and the intergenic spacer (IGS). Hydrobiologia. 616:229–239. doi: 10.1007/s10750-008-9596-5
- Knudsen S. 1999. Promoter 2.0: for the recognition of Pol II promoter sequences. Bioinformatics. 15:356–361. doi: 10.1093/bioinformatics/15.5.356
- Li ZB, Appleyard SA, Elliott NG. 2006. Population structure of Haliotis rubra from south Australia inferred from nuclear and mtDNA analyses. Acta Oceanologica Sinica. 25:99–112.
- Li YY, Shen SD, He LH, Xu P, Lu S. 2010. Sequence analysis of rDNA intergenic spacer (IGS) of Porphyra haitanensis. Journal of Applied Phycology. 22:187–193. doi: 10.1007/s10811-009-9441-x
- Li YY, Shen SD, He LH, Xu P, Wang GC. 2009. Sequence analysis of the ITS region and 5.8S rDNA of Porphyra haitanensis. Chinese Journal of Oceanology and Limnology. 27(3):493–501. doi: 10.1007/s00343-009-9168-1
- Li XC, Xu JJ, He Y, Shen SS, Zhu JY, Shen ZG. 2016. The complete nuclear ribosomal DNA (nrDNA) cistron sequence of Pyropia yezoensis (Bangiales, Rhodophyta). Journal of Applied Phycology. 28:663–669. doi: 10.1007/s10811-015-0522-8
- Li L, Zhong CH, Bian YB. 2014. The molecular diversity analysis of Auricularia auricula-judae in China by nuclear ribosomal DNA intergenic spacer. Electronic Journal of Biotechnology. 17:27–33. doi: 10.1016/j.ejbt.2013.12.005
- Ling SD. 2008. Range expansion of a habitat-modifying species leads to loss of taxonomic diversity: a new and impoverished reef state. Oecologia. 156:883–894. doi: 10.1007/s00442-008-1043-9
- Liu W, Heasman M, Simpson R. 2009. Growth and reproductive performance of triploid and diploid blacklip abalone, Haliotis rubra (Leach, 1814). Aquaculture Research. 40:188–203. doi: 10.1111/j.1365-2109.2008.02082.x
- Liu W, Heasman M, Simpson R, Dworjanyn S, Pirozzi I. 2006. Growth and feeding in juvenile triploid and diploid blacklip abalone, Haliotis rubra (Leach, 1814), at two temperatures. Aquaculture Nutrition. 12:410–417. doi: 10.1111/j.1365-2095.2006.00442.x
- Liu Y, Xu T, Robinson N, Qin J, Li X. 2015. Cryopreservation of sperm in farmed blacklip abalone (Haliotis rubra Leach, 1814). Aquaculture Research. 46:2628–2636. doi: 10.1111/are.12415
- Maggini F, Gelati MT, Spolverini M, Frediani M. 2008. The intergenic spacer region of the rDNA in Olea europaea L. Tree Genetics & Genomes. 4:293–298. doi: 10.1007/s11295-007-0109-x
- Mayfield S, Mundy C, Gorfine H, Hart AM, Worthington D. 2012. Fifty years of sustained production from the Australian abalone fisheries. Review in Fisheries Science. 20:220–250. doi: 10.1080/10641262.2012.725434
- Maynard BT, Kerr LJ, McKiernan JM, Jansen ES, Hanna PJ. 2005. Mitochondrial DNA sequence and gene organization in the Australian blacklip abalone Haliotis rubra (Leach). Marine Biotechnology. 7:645–658. doi: 10.1007/s10126-005-0013-z
- Miller KJ, Mundy CN, Mayfield S. 2014. Molecular genetics to inform spatial management in benthic invertebrate fisheries: a case study using the Australian Greenlip Abalone. Molecular Ecology. 23:4958–4975. doi: 10.1111/mec.12914
- Miller AD, van Rooyen A, Rašić G, Ierodiaconou DA, Gorfine HK, Day R, Wong C, Hoffmann AA, Weeks AR. 2016. Contrasting patterns of population connectivity between regions in a commercially important mollusc Haliotis rubra: integrating population genetics, genomics and marine LiDAR data. Molecular Ecology. 25:3845–3864. doi: 10.1111/mec.13734
- Notredame C, Higgins DG, Heringa J. 2000. T-Cofee: a novel method for multiple sequence alignments. Journal of Molecular Biology. 302:205–217. doi: 10.1006/jmbi.2000.4042
- Parvaresh M, Talebi M, Sayed-Tabatabaei BE. 2014. Molecular characterization of ribosomal DNA intergenic spacer (IGS) region in pomegranate (Punica granatum L.). Plant Systematics and Evolution. 300:899–908. doi: 10.1007/s00606-013-0928-1
- Pederson HG, Barrett NS, Frusher SD, Buxton CD. 2008. Effect of predator–prey and competitive interactions on size at emergence in the black-lip abalone Haliotis rubra in a Tasmanian MPA. Marine Ecology Progress Series. 366:91–98. doi: 10.3354/meps07521
- Posada D. 2009. Selection of models of DNA evolution with jModelTest. bioinformatics for DNA sequence analysis. Methods in Molecular Biology. 537:93–112. doi: 10.1007/978-1-59745-251-9_5
- Sanders MJ, Beinssen KH. 1998. A comparison of management strategies for the rehabilitation of a fishery: applied to the fishery for blacklip abalone Haliotis rubra in the Western Zone of Victoria (Australia). Fisheries Research. 38:283–301. doi: 10.1016/S0165-7836(98)00158-1
- Santoni S, Bervillé A. 1992. Characterization of the nuclear ribosomal DNA units and phylogeny of Beta L. wild forms and cultivated beets. Theoretical and Applied Genetics. 83(5):533–542. doi: 10.1007/BF00226896
- Tamura K, Stecher G, Peterson D, Filipski A, Kumar S. 2013. MEGA 6: molecular evolutionary genetics analysis version 6.0. Molecular Biology and Evolution. 30(12):2725–2729. doi: 10.1093/molbev/mst197
- Valentine JP, Tarbath DB, Frusher SD, Mundy CN, Buxton CD. 2010. Limited evidence for ecosystem-level change on reefs exposed to Haliotis rubra (‘blacklip abalone’) exploitation. Austral Ecology. 35:806–817. doi: 10.1111/j.1442-9993.2009.02088.x
- Van Wormhoudt A, Gaume B, Le Bras Y, Roussel V, Huchette S. 2011. Two different and functional nuclear rDNA genes in the abalone Haliotis tuberculata: tissue differential expression. Genetica. 139:1217–1227. doi: 10.1007/s10709-011-9623-8
- Xu JJ, Jiang B, Chai SM, He Y, Zhu JY, Shen ZG, Shen SD. 2016. Complete nuclear ribosomal DNA sequence amplification and molecular analyses of Bangia (Bangiales, Rhodophyta) from China. Chinese Journal of Oceanology and Limnology. 34(5):1044–1053. doi: 10.1007/s00343-016-5033-1
- Yu JH, Li HX, Ge XP, Li JL, Tang YK. 2010. Characteristics analysis of complete ribosomal DNA sequence in Chinese mitten crab (Eriocheir sinensis). Journal of Fisheries of China. 34(5):698–703. (In Chinese with English Abstract). doi: 10.3724/SP.J.1231.2010.06659
- Zheng X, Chang QC, Zhang Y, Tian SQ, Lou Y, Duan H, Guo DH, Wang CR, Zhu XQ. 2014. Characterization of the complete nuclear ribosomal DNA sequences of Paramphistomum cervi. Scientific World Journal. 2014:751907.