ABSTRACT
New Zealand benthic and epiphytic dinoflagellate records increased in recent years as harmful algal bloom research increased. This was largely due to risk assessments of micro-algal biotoxins for the seafood industry and concerns regarding ciguatera fish poisoning in humans from toxic finfish. High-throughput sequencing enabled the detection of dinoflagellate species that were previously overlooked by microscopy, particularly where diatoms or sediments obscured visual identification. This checklist includes new species records for New Zealand and species usually considered planktonic, but which have benthic life stages. Thirty-one dinoflagellate genera were recorded from isolations and descriptions of living cells: Alexandrium, Amphidinium, Archaeperidinium, Azadinium, Biecheleria, Blastodinium, Bysmatrum, Coolia, Crypthecodinium, Cryptoperidiniopsis, Durinskia, Ensiculifera, Fukuyoa, Gambierdiscus, Gymnodinium, Gyrodinium, Heterocapsa, Karenia, Kryptoperidinium, Ostreopsis, Pelagodinium, Pentapharsodinium, Pfiesteria, Polarella, Prorocentrum, Protodinium, Protoperidinium, Pseudopfiesteria, Scrippsiella, Togula and Vulcanodinium. A further nine genera were detected by high-throughput sequencing: Cachonina, Dinophysis, Fragilidium, Gonyaulax, Karlodinium, Lepidodinium, Protodinium, Symbiodinium and Woloszynskia.
Introduction
Research on the unicellular eukaryotic dinoflagellates has evolved globally in recent years. In the last decades of the twentieth century, research was driven by the harmful effects of toxic and noxious dinoflagellate blooms on fisheries and aquaculture. The New Zealand shellfish industry and the New Zealand government biotoxin regulators responded to harmful algal blooms (HABs) around New Zealand's coastline by implementing internationally recognised biotoxin and phytoplankton monitoring programmes in 1993 and 1994, respectively (Rhodes et al. Citation2013b). These programmes are on-going and the samples monitored are shellfish flesh, to test for biotoxins, and seawater, to determine the presence and concentration of planktonic HAB species. The micro-algal identification is via light microscopy and is supported by molecular tests. Risk assessments are provided to seafood managers within 24 hours of sample receipt to help reduce impacts on commercial fisheries and public health (Rhodes & Munday Citation2016). Blooms of potentially toxin-producing dinoflagellate genera, such as Alexandrium (MacKenzie Citation2014), Dinophysis (MacKenzie et al. Citation2004), Karenia (de Salas et al. Citation2005; Haywood et al. Citation2004; Smith et al. Citation2007), Protoceratium (Satake et al. Citation1997) and Gymnodinium (for example, the paralytic shellfish toxin (PST) producer, G. catenatum; MacKenzie Citation2014), occur regularly around New Zealand's coastline.
Benthic and epiphytic dinoflagellates, however, occur mainly attached to surfaces, including sediments, coral, macroalgae and seagrasses, and motile forms or re-suspended cells (as may occur following storm events) are only occasionally detected in the sea water samples for phytoplankton monitoring. Even when the epiphytic dinoflagellate Ostreopsis siamensis covers macroalgae in dense concentrations in sub-tropical Northland, during the austral summers (Shears and Ross Citation2009; Rhodes Citation2011), only a few cells are detected in the phytoplankton monitoring samples collected from those areas (Cawthron Institute Microalgae Laboratory, unpublished data).
Benthic and epiphytic harmful algal bloom (BHABs) species produce diverse toxins and cause different issues to planktonic HAB species. For example, ciguatera fish poisoning (CFP) in humans is caused when the toxin producers, epiphytic species in the genus Gambierdiscus, are consumed along with macroalgae and coral by herbivorous fish. The toxins (ciguatoxins) bioaccumulate in fish tissue, which are then consumed by humans. BHAB species therefore need a specialised approach for their detection and quantification (GEOHAB Citation2012). One method which has been used by the authors in Northland, New Zealand, utilises an artificial substrate, which standardises sampling techniques and enables comparisons between locations, including international BHAB data (Tester et al. Citation2014; Berdalet et al. Citation2017; Smith et al. Citation2017a). This approach has also proved particularly useful for obtaining live dinoflagellate isolates free from debris, as well as for determining species presence using molecular techniques (e.g. quantitative PCR, high-throughput sequencing). Parsons et al. (Citation2017), however, have advised caution in using the artificial substrates for determining Gambierdiscus abundance in some habitats as they found a lack of consistent correlation between cell concentrations from their deployments compared with those from the natural macrophyte hosts at the sites they investigated. The method was particularly useful, however, in New Zealand's protected northern embayments (Smith et al. Citation2017b).
The benthic life stages of some mainly planktonic dinoflagellate species may be found in the sediments or attached to macroalgae and eel grasses, but will also be detected in motile form during phytoplankton monitoring. Any study of the meroplankton, i.e. plankton spending at least one life stage in the benthos, must include dormant forms. Ten per cent of dinoflagellates have been estimated to produce resting cysts (Dale Citation1983), and these increase the chances of survival during unfavourable environmental conditions. There are also temporary or ecdysal (non-dormant) cysts which may also be a response to adverse conditions. The cycling between benthic and planktonic stages may be rapid, for example, dinoflagellates that inhabit shallow pools and are vulnerable to tidal cycles (Hoppenrath et al. Citation2014).
Salgado et al. (Citation2018) also discuss the flattened cells of the marine dinoflagellates Protoceratium reticulatum and Ceratocorys mariaovidiorum (Order Gonyaulacales). (The name C. mariaovidiorum is a synonym for Pentaplacodinium saltonense (Mertens et al. Citation2017).) The flattened cells differ morphologically from the planktonic form of each species and, whilst mostly benthic, they may become motile if disturbed. Benthic life stages are also included in this study.
The research focus on biotoxin-producing dinoflagellates continues globally and toxicity testing (e.g. the mouse bioassay) is invaluable as it indicates when a toxic compound is present that has not been detected by current chemical tests (Munday et al. Citation2017). There has been a slight shift in dinoflagellate toxin production research recently, from sampling improvements and the development of new chemical tests, to the production of valuable compounds. For example, the cyclic imine portimine, which was isolated from the non-motile benthic cells of Vulcanodinium rugosum, has potential as an agent of apoptosis (Selwood et al. Citation2013). Portimine has very low acute toxicity to mice but is highly cytotoxic to cultured cells and is one of the most potent naturally derived inducers of apoptosis to be discovered (Cuddihy et al. Citation2016). Compounds are sought from other dinoflagellates for their nutritional value (for example, non-meat proteins) and for potential pharmacological applications (Assunção et al. Citation2017).
There is also a need to determine the microbial biodiversity of New Zealand in view of expected changes in the communities due to climate change. It has recently been discovered through novel metabarcoding approaches that, in the marine environment, dinoflagellates represent up to 50% of the planktonic species richness, representing a major source of primary production and nutrient cycling in the world's oceans. Of the approximately 5000 living microalgae species known (including the dinoflagellates), approximately 6% multiply to form HABs (Kohli et al. Citation2014, Citation2015). Less than 2% of the described species produce potent biotoxins (Hallegraeff Citation2014), which may be directly toxic to shellfish or fish, or toxic to animals or humans consuming contaminated seafood. Dinoflagellate distributions are already expanding, with species from tropical regions being detected in temperate to sub-tropical regions. For example, a previously considered tropical species, Gambierdiscus carpenteri, now blooms regularly in temperate southern New South Wales, Australia (Kohli et al. Citation2014). A combination of factors is probably responsible, including an increase in average coastal seawater temperatures along the south east Australian coastline and associated changes in the direction of the main currents (http://climatechange.environment.nsw.gov.au/About-climate-change-in-NSW/Evidence-of-climate-change/Observed-Australian-climate-change: accessed 5 March 2018). There is also the added complicating factor of adaptation of tropical species to cooler temperatures. Increases in average annual coastal seawater temperatures are also occurring in northern New Zealand waters (Ministry for the Environment Citation2016) and while the risk of CFP occurring due to local Gambierdiscus blooms is currently low, there is a potential risk of CFP occurring in New Zealand in the future (Rhodes et al. Citation2017a).
The identification of benthic dinoflagellates, historically based on light microscope morphological descriptions of motile cells and their resting cysts, now often requires electron microscope examination for a confident identification and DNA sequence data can be critical in deciding on the differentiation of morphologically similar species (for example, Gambierdiscus species, Smith et al. Citation2017a, Citation2017b). Differences in pigments and toxin production strengthen the characterisation of a species. For example, Gambierdiscus species have been observed in high concentrations in samples collected from macroalgae in Rangitāhua/Kermadec Islands (a New Zealand territory approximately 1000 km north-east of the mainland; Rhodes et al. Citation2017b). The genus is readily recognised under the light microscope, but identifications to species level in environmental samples have required molecular approaches, for example quantitative Polymerase Chain Reaction (qPCR) assays or high-throughput sequencing metabarcoding (Smith et al. Citation2017a). Genera that commonly co-occur with Gambierdiscus in New Zealand waters are Amphidinium, Coolia, Ostreopsis and Prorocentrum. These are readily recognised at the genus level, but may also require molecular approaches for species determination (Smith et al. Citation2017b).
Benthic dinoflagellate species from sub-tropical New Zealand
Sub-tropical New Zealand includes the Rangitāhua/Kermadec Islands as well as the northern coastal waters of New Zealand ( and ). For this study, the subtropics refers to geographic and climate zones located approximately between the tropics at latitude 23.5° and temperate zones (normally referring to latitudes 35°–66.5°) to the south of the Equator. Average annual sea surface temperatures (SSTs) at Raoul Island ranges from approximately 17°C–23°C (a 6°C range; Sutton et al. Citation2012, Trnski & de Lange Citation2015).
Figure 1. Map of Rangitāhua/Kermadec Islands highlighting the sampling sites where epiphytic dinoflagellates referred to in this study were collected from macroalgae.
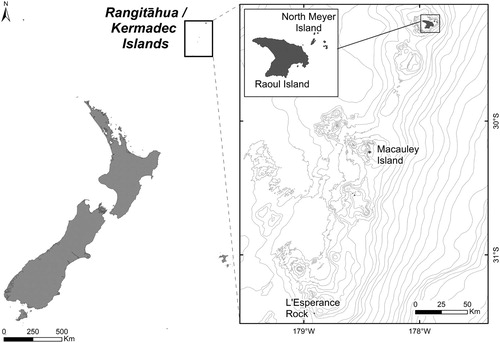
Figure 2. Map of New Zealand highlighting sites where benthic and epiphytic dinoflagellates referred to in this study were collected.
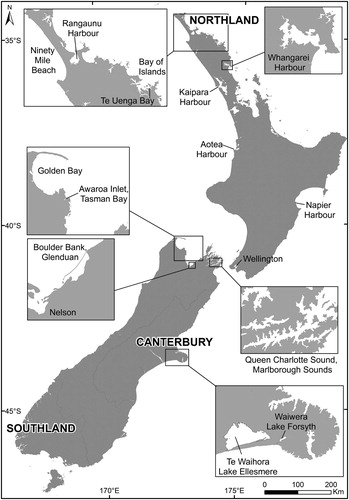
For Northland, New Zealand, average annual SSTs range from approximately 13°C–23°C (a 10°C range; https://terra.nasa.gov/data/modis-data). Sampling for dinoflagellates in the Kermadec Islands has been carried out during four Auckland War Memorial Museum expeditions to determine the biodiversity of the region (Rhodes et al. Citation2014a, Citation2015, Citation2017b, Citation2017d; Smith et al. Citation2017a). Sampling of surface sediments in Northland has also been carried out on numerous occasions (Rhodes et al. Citation2010a, Citation2010b, Citation2013a).
Rangitāhua / Kermadec Islands
The Kermadec Islands are at the north-eastern limit of New Zealand's territories (), approximately 1000 km from mainland New Zealand. They currently include a 747,000-hectare marine reserve with the only human habitation being Raoul Island Station.
Collection of samples for the isolation of epiphytic dinoflagellates from macroalgae was carried out at Rangitāhua/Raoul Island in November 2013 (Rhodes et al. Citation2014a, Citation2014b), North Meyer Island in November 2015 (Rhodes et al. Citation2015) and at Macauley Island in 2016 and 2017 (Rhodes et al. Citation2017b). Samples were transported back to Cawthron Institute, Nelson, New Zealand, for dinoflagellate identification. Isolates of Gambierdiscus (Family Ostreopsidaceae), the causative organism of CFP, were of interest as CFP afflicts many people in the Pacific region, including Australia (Kohli et al. Citation2017), following consumption of contaminated fish, even causing deaths (Rongo & van Woesik Citation2012). The genus Gambierdiscus is common throughout tropical and sub-tropical regions (Litaker et al. Citation2010; Parsons et al. Citation2012) and species identified from the Kermadec expeditions were G. australes (the dominant species in samples, from all sites and a producer of maitotoxins), G. honu (a previously undescribed species; Rhodes et al. Citation2017c) and G. polynesiensis, a known ciguatoxin (CTX) producer, from Macauley Island (Rhodes et al. Citation2017b). Two G. polynesiensis isolates from Macauley Island were cultured and one produced trace levels of CTX-3 as well as isomers of CTX-3B/C and -4A/B (isolate CAWD254), but the second isolate produced no known CTXs (CAWD259).
Associated dinoflagellates from the same family included Ostreopsis sp. 3 (Sato et al. Citation2011), O. ovata and Coolia malayensis (Rhodes et al. Citation2014b). Isolates from the family Gymnodiniaceae included Amphidinium massartii and A. carterae and from the family Prorocentraceae included Prorocentrum emarginatum, P. hoffmannianum and P. lima. All were identified by analysis of DNA sequencing data (large subunit ribosomal RNA gene region) of clonal cultures as well as by their morphology (Rhodes et al. Citation2014a, Citation2014b, Citation2015, Citation2017a, Citation2017b, Citation2017d).
Northland (Rangaunu and Whangaroa Harbours and the Bay of Islands)
The far north of New Zealand has a sub-tropical climate and the coastline varies from muddy bottomed mangrove (Avicennia marina) dominated harbours surrounded by eel grass (Zostera muelleri) to rocky tidal pools (providing habitat for macroalgae) and sandy beaches (). Comprehensive field studies have been carried out over the last two decades in Rangaunu Harbour, where Pacific oyster (Crassostrea gigas) farmers suffered economic losses through regulatory closures of their leases. The closures were for extended periods due to positive toxin results for the oysters using the mouse bioassay. The biotoxins proved to be pinnatoxins, produced by the dinoflagellate Vulcanodinium rugosum (Family Peridiniales incertae sedis; Rhodes et al. Citation2011), which formed dense mats of non-motile cells on the surface sediments near the edge of mangrove and eel grass habitat around the harbour. At times, the cells become motile and are taken up by the farmed oysters grown on racks further out in the harbour. A morphologically similar and genetically related species, Bysmatrum sp. (Dr Lincoln MacKenzie, Cawthron Institute, unpublished data), were also been found in the same habitat as V. rugosum.
Another dinoflagellate found as mats on surface sediments in Rangaunu Harbour and on oyster farm infrastructure was Prorocentrum lima. Cells were also detected in sea water samples when the mats were disturbed by storm events (Rhodes & Syhre Citation1995). Okadaic acid (OA), the cause of diarrhetic shellfish poisoning, and OA-diol esters were found at approximately equal levels in P. lima strains from Rangaunu Harbour. Some strains produced the diarrhetic shellfish toxin DTX1 at low concentrations and a novel nitrogen-containing polyether compound was also detected (Rhodes et al. Citation2006a). Other Prorocentrum species isolated from Rangaunu Harbour surface sediments included P. maculosum and P. hoffmanianum, and from coralline turfs at Te Uenga Bay in the Bay of Islands, P. rhathymum, P. thermaeum and P. triestinum (the latter usually planktonic). The related species P. balticum, P. compressum and P. gracile have only been found as planktonic species.
Amphidinium species were found in the same sediment and macroalgae samples as P. lima. They included A. boggayum, A. carterae, A. massartii, A. mootonorum and A. thermaeum. The type specimen of A. trulla was isolated from seawater in Rangaunu Harbour, but the cells have been described as benthic in Australia (Murray et al. Citation2004). Many antifungal and haemolytic amphidinol analogues have been isolated from an A. carterae isolate from New Zealand and their structures characterised (Houdai et al. Citation2001; Echigoya et al. Citation2005).
Temporary and resting dinoflagellate cysts were found associated with beached macroalgae and sediments from Ninety Mile Beach, which is situated in north-west New Zealand adjacent to Rangaunu Harbour (). At times, the beach wrack is covered with GreenshellTM mussel spat (Perna canaliculus), which is desirable for on-growing on marine farms in more southern, temperate waters. Monitoring of the macroalgae bearing the spat, prior to transport by truck and ferry to the Marlborough Sounds and Stewart Island aquaculture regions, was carried out to prevent transference of toxin dinoflagellates to other parts of New Zealand (Rhodes et al. Citation1994). Temporary cysts of Karenia mikimotoi (Family Brachidiniaceae) bearing a mucilaginous outer coat and resting cysts of Alexandrium ostenfeldii (Family Ostreopsidaceae), a spirolide producer, were found during monitoring of the macroalgae. Cysts of Gymnodinium catenatum were also found in sediments collected from Kaipara and Aotea Harbours (north-west Northland) as well as in Whangarei Harbour to the north-east (Mackenzie et al. Citation1996; MacKenzie Citation2009; Taylor and MacKenzie Citation2001).
In the Bay of Islands, Fukuyoa paulensis, previously classified as Gambierdiscus cf. yasumotoi (Rhodes et al. Citation2013a), was discovered at Te Uenga Bay in 2013. This raised the concern that, with increasing coastal seawater temperatures, toxic Gambierdiscus populations could become established in Northland, leading to a risk of CFP from New Zealand fish populations. Gambierdiscus was reported in Northland, New Zealand, nearly two decades ago as G. toxicus (Chang Citation1996). The morphological plasticity of the species in the genus made microscopic identification difficult in the past and the classification of G. toxicus before 1999 is uncertain, but molecular markers now allow rapid determination to species level (Parsons et al. Citation2012).
The producer of palytoxin (PLTX)-like compounds, Ostreopsis siamensis, has been a bloom former down the eastern coastline of Northland for many years (Shears and Ross Citation2009; Rhodes Citation2011) and occurred with F. paulensis in the Bay of Islands. Despite dense blooms covering macroalgae in Northland during 2014, and the fact that cultured isolates were positive for PLTXs (0.2 pg PLTX equiv./cell), no PLTX was found in herbivorous fish or other marine grazers at that time (Rhodes et al. Citation2015).
A study carried out in the Bay of Islands in 2014 resulted in new taxonomic records for New Zealand based on high-throughput sequencing metabarcoding analyses. Many of the species reported were not detected by microscopic investigation of surface sediment or macro-algal samples, which highlighted the strength of this high-throughput sequencing technique (Smith et al. Citation2017b; ).
Table 1. Sub-tropical benthic/epiphytic dinoflagellates, including species with benthic life stages, isolated and identified from New Zealand and its territories.
Table 2. Temperate benthic/epiphytic dinoflagellates, including species with benthic life stages, isolated and identified from New Zealand and its territories.
Table 3. List of epiphytic and benthic families and genera in the Class Dinophyceae F.E. Fritsch reported in New Zealand, including records from metabarcoding analyses (Smith et al. Citation2017a, Citation2017b).
Benthic dinoflagellate species from temperate New Zealand
The temperate epiphytic/benthic dinoflagellate microflora of New Zealand differs from the sub-tropical microflora, particularly from those species found in Rangitāhua/Kermadec Islands. The annual average range in SSTs for the temperate region is approximately 9°C–20°C (cooler in Canterbury where the range is 8°C–12°C; https://terra.nasa.gov/data/modis-data).). There are several species, however, that have been found in both the sub-tropical northern and the cooler southern waters of New Zealand's mainland (). Coolia malayensis, for example, was found in unlined hatchery pond sediments at Glenhaven, Nelson (Rhodes et al. Citation2014b). Ostreopsis siamensis has been reported in low cell concentrations from as far south as temperate Wellington coastal waters (New Zealand's National Marine Biotoxin monitoring programme records; Rhodes Citation2011). The chain-forming dinoflagellate G. catenatum, found in cyst form in northern sediments, was probably introduced to southern waters during transfer of drift macroalgae bearing mussel spat collected by the truckload from Ninety Mile Beach. This species has been detected in its motile form in phytoplankton samples collected from the outer Marlborough Sounds (New Zealand's National Marine Biotoxin monitoring programme records). One species found throughout both sub-tropical and temperate regions, in water samples, surface sediments and associated with macroalgae, is P. lima.
The success of New Zealand's phytoplankton monitoring programmes is exemplified by the excellent management of blooms of the PST producing HAB species G. catenatum, A. pacificum (previously A. catenella), A. minutum and A. ostenfeldii in recent years (MacKenzie Citation2014). However, benthic cyst forms of these species are not included in micro-algal monitoring programmes and are only known to be a vital component of the microflora because of on-going research efforts (Knight and MacKenzie Citation2015). In fact, resting cysts (hypnozygotes) of A. ostenfeldii have been reported in sediments collected from all around the New Zealand coastline (MacKenzie et al. Citation1996). Vegetative cell cultures were established by hatching cysts and the toxicity of these cultures was ascertained using high-performance liquid chromatography, with considerable differences detected in the toxin profiles of strains isolated from different sites (MacKenzie et al. Citation1996). Cysts of A. pacificum have been found in sediments following bloom events in the temperate waters of Queen Charlotte Sound, Marlborough Sounds, with a heavily impacted area being Opua Bay. QPCR assays have been developed to rapidly quantify the cysts to determine the future risk to GreenshellTM mussel farms in the vicinity.
The family Gymnodiniaceae includes the genera Togula as well as Amphidinium and both have been found in temperate New Zealand waters associated with surface sediments and on marine structures. The species T. jolla was found on sand ripples in Awaroa Inlet, Tasman Bay, in the north-east of the South Island of New Zealand. The inlet is known for its golden sands and the golden-brown Togula blended with its surroundings. The same species has also been isolated from surface sediments near Napier in the east of the North Island. Amphidinium spp. are common in sub-tropical habitats associated with macroalgae and with sediments (especially in the non-motile form), but A. carterae is also regularly reported in sea water monitoring samples collected from temperate waters, often associated with A. operculatum.
The heterotrophic dinoflagellate family Thoracosphaeraceae includes Pfiesteria piscicida, caused major fish kills along the eastern USA seaboard in the northern summer of 1997 (Burkholder and Glasgow Citation2001). Such fish kills had previously been associated with neurological health symptoms in local fishers (Burkholder et al. Citation1992). The economic ramifications were considerable as consumers panicked and sales of seafood went down with a calculated loss of US$43M for the state. The ‘halo effect’ also led to the avoidance of Maryland for boat charters with the recreational fishing industry also losing several million dollars. An overall economic loss of US$50M was estimated for just a four-month period (CENR Citation2000). Since then, Pfiesteria species have been reported from at least 15 countries and the genus is considered to have a global distribution (Rublee et al. Citation2004; Citation2005). In 2000, Pseudopfiesteria shumwayae (Litaker et al. Citation2005) (synonym: Pfiesteria shumwayae; Guiry & Guiry Citation2017) was reported in surface sediments from estuaries in the Kaipara Harbour in the North Island of New Zealand. In the South Island, it was detected, using molecular methods, in sediment samples from Tasman Bay, Golden Bay, Marlborough and Southland. It was also detected in Canterbury in Wairewa/Lake Forsyth and Te Waihora/Lake Ellesmere (Rhodes et al. Citation2002). PCR-based assays also determined the presence of P. piscicida DNA in Tasman Bay and the Canterbury Lakes (Rhodes et al. Citation2006b). A research study in Tasmania, Australia, showed cross-reactivity of the related species, Cryptoperidiniopsis brodyi, with the PCR assay for P. piscicida and, while there has been scanning electron microscope confirmation of the presence of P. piscicida in New Zealand, there may have been an over-estimation of occurrence in the earliest studies (Park Citation2006; Park et al. Citation2007). Using a specifically designed PCR assay, C. brodyi was detected in 2007 in Wairewa/Lake Forsyth (Rhodes and Smith, unpublished data).
The azaspiracid-producing dinoflagellate genus, Azadinium (Family Amphidomataceae), has been detected in cyst form in surface sediments from the Marlborough Sounds (Rhodes and Smith, unpublished data). The Irish shellfish aquaculture industry, with a value of >US$65M per annum, suffered economic losses from the closure of export markets due to azaspiracids in 1995. The organism responsible for toxin production was not identified until more than a decade later. It was classified as Azadinium spinosum (Tillmann et al. Citation2009). Many other Azadinium species, both toxic and non-toxic, have been identified since 2007 (Tillmann et al. Citation2014). Motile cells of Azadinium poporum were isolated from seawater in the Marlborough Sounds, but the isolate proved non-toxic (Smith et al. Citation2016) and Azadinium dalianense was detected in benthic dinoflagellate communities from Northland, New Zealand (Smith et al. Citation2017b).
Metabarcoding results
Nineteen new species records of dinoflagellate species for New Zealand were detected in samples from Northland, collected from macroalgae and on artificial substrates by high-throughput sequencing, using metabarcoding techniques, and the results were published in 2017 (Smith et al. Citation2017b, refer Table 4 in that publication). Metabarcoding has also been used to characterise benthic dinoflagellates communities in the Kermadec Islands. The analyses demonstrated a dominance by Gambierdiscus species and the first confirmed identification of G. polynesiensis, a known CTX producer, in New Zealand waters. Other known toxin-producing genera were also detected, including Alexandrium, Amphidinium, Azadinium, Dinophysis, Ostreopsis and Prorocentrum. It is expected that these novel next-generation sequencing methods, including metabarcoding, will reveal many more cryptic species in New Zealand's epiphytic and benthic marine habitats.
Conclusions
The epiphytic and benthic dinoflagellates of New Zealand have been the subject of research in recent years, but despite that the group have been barely touched on. Further studies are planned, particularly in shellfish growing areas where there are perceived health risks from dinoflagellate cyst ‘seed’ beds. In northern New Zealand, where a potential risk of CFP has been noted, a continuation of monitoring using high-throughput molecular tools is planned. It is likely that in just a few years this checklist will be increased substantially as sites are reinvestigated and new sites explored. In the interim, this study provides a baseline for future work with 30 genera reported.
Acknowledgements
Samples for identification of dinoflagellates from Rangitāhua/Kermadec Islands were collected by Tom Trnski and colleagues during expeditions carried out by the Auckland War Memorial Museum. Thanks also for technical help to Janet Adamson, Laura Biessy and Kendall Morman, including obtaining DNA sequence data. Thanks to Krystyna Ponikla and Sarah Challenger for maintaining the unique Cawthron Institute Culture Collection of Microalgae.
Disclosure statement
No potential conflict of interest was reported by the authors.
Additional information
Funding
References
- Assunção J, Guedes A, Malcata FX. 2017. Biotechnological and pharmacological applications of biotoxins and other bioactive molecules from dinoflagellates. Marine Drugs. 15:393. doi: 10.3390/md15120393
- Berdalet E, Tester PA, Chinain M, Fraga S, Lemée R, Litaker W, Penna A, Usup G, Vila M, Zingone A. 2017. Harmful algal blooms in benthic systems: recent progress and future research. Oceanography. 30:36–45. doi: 10.5670/oceanog.2017.108
- Burkholder JM, Glasgow HB. 2001. History of toxic pfiesteria in North Carolina Estuaries from 1991 to the present: many toxic pfiesteria outbreaks have plagued the Albemarle-Pamlico Estuarine System, including events both before and after the 1997 outbreaks in Chesapeake Bay. BioScience. 51:827–841. doi: 10.1641/0006-3568(2001)051[0827:HOTPIN]2.0.CO;2
- Burkholder JM, Noga EJ, Hobbs CH, Glasgow Jr HB. 1992. New ‘phantom’ dinoflagellate is the causative agent of major estuarine fish kills. Nature. 358:407–410. doi: 10.1038/358407a0
- CENR. 2000. National assessment of harmful algal blooms in US waters. National Science and Technology Council Committee on Environment and Natural Resources, Washington, DC, 38 pp.
- Chang FH. 1996. Shellfish toxin update. Seafood New Zealand. 26, February 1996.
- Cuddihy SL, Drake S, Harwood DT, Selwood AI, McNabb PS, Hampton MB. 2016. The marine cytotoxin portimine is a potent and selective inducer of apoptosis. Apoptosis. doi:10.1007/s10495-016-1302-x.
- Dale B. 1983. Dinoflagellate resting cysts: “benthic plankton”. In: Fryxell GA, editor. Survival strategies of the algae. New York (NY): Cambridge University Press; p. 69–136.
- de Salas MF, Rhodes LL, Mackenzie LA, Adamson JE, Ponikla K. 2005. The gymnodinoid genera Karenia and Takayama (Dinophyceae) in New Zealand coastal waters. New Zealand Journal of Marine and Freshwater Research. 39:135–139. doi: 10.1080/00288330.2005.9517296
- Echigoya R, Rhodes L, Oshima Y, Satake M. 2005. The structures of five new antifungal and haemolytic amphidinol analogs from Amphidinium carterae collected in New Zealand. Harmful Algae. 4:383–389. doi: 10.1016/j.hal.2004.07.004
- GEOHAB. 2012. Global ecology and oceanography of harmful algal blooms, GEOHAB Core Research Project: HABs in benthic systems. In Berdalet E, Tester P, Zingone A, editors. IOC of UNESCO and SCOR, Paris and Newark, 64 pp.
- Guiry MD in Guiry MD, Guiry GM. 2017. AlgaeBase. World-wide electronic publication, National University of Ireland, Galway. http://www.algaebase.org; searched on 14 August 2017.
- Hallegraeff GM. 2014. Harmful algae and their toxins: progress, paradoxes and paradigm shifts. In: Rossini GP, editor. Toxins and biologically active compounds from microalgae. Enfield: Science Publishers; p. 3–20.
- Haywood AJ, Steidinger KA, Truby EW, Bergquist PR, Bergquist PL, Adamson J, MacKenzie L. 2004. Comparative morphology and molecular phylogenetic analysis of three new species of the genus Karenia (Dinophyceae) from New Zealand. Journal of Phycology. 40:165–179. doi: 10.1111/j.0022-3646.2004.02-149.x
- Hoppenrath M, Murray SA, Chomèrat N, Horiguchi T. 2014. Marine benthic dinoflagellates – unveiling their worldwide biodiversity. Stuttgart (Germany): Schweizerbart; 276 pp.
- Houdai T, Matsuoka S, Murata M, Satake M, Ota S, Oshima Y, Rhodes LL. 2001. Acetate labeling patterns of dinoflagellate polyketides, amphidinols 2, 3 and 4. Tetrahedron 57: 5551-5555. doi: 10.1016/S0040-4020(01)00481-1
- Jørgensen MF, Murray S, Daugbjerg N. 2004. A new genus of athecate interstitial dinoflagellates, Togula gen. nov., previously encompassed within Amphidinium sensu lato: inferred from light and electron microscopy and phylogenetic analyses of partial large subunit ribosomal DNA sequences. Phycological Research. 52:284–299. doi: 10.1111/j.1440-1835.2004.tb00338.x
- Karafas S, Teng ST, Leaw CP, Alves-de-Souza C. 2017. An evaluation of the genus Amphidinium (Dinophyceae) combining evidence from morphology, phylogenetics, and toxin production, with the introduction of six novel species. Harmful Algae. 68:128–151. doi: 10.1016/j.hal.2017.08.001
- Knight B, MacKenzie AL. 2015. Modelling the potential impact of Alexandrium catenella in a marine farming area. In: MacKenzie AL, editor. Marine and Freshwater Harmful Algae. Proceedings of the 16th International Conference on Harmful Algae, Wellington, New Zealand 27th–31st October 2014, p. 155–158.
- Kohli GS, Farrell H, Murray SA. 2015. Gambierdiscus, the cause of ciguatera fish poisoning: an increased human health threat influenced by climate change. In: Botana LM, Louzao C, Vilariño N, editors. Climate change and marine and freshwater toxins. Berlin: De Gruyter. Chapter 9, p. 273–312.
- Kohli GS, Haslauer K, Sarowar C, Kretzschmar AL, Boulter M, Harwood DT, Laczka O, Murray SA. 2017. Qualitative and quantitative assessment of the presence of ciguatoxin, P-CTX-1B, in Spanish Mackerel (Scomberomorus commerson) from waters in New South Wales (Australia). Toxicology Reports. 4:328–334. doi: 10.1016/j.toxrep.2017.06.006
- Kohli GS, Murray SA, Neilan BA, Rhodes LL, Harwood DT, Smith KF, Meyer L, Capper A, Brett S, Hallegraeff GM. 2014. High abundance of the potentially maitotoxic dinoflagellate Gambierdiscus carpenteri in temperate waters of New South Wales, Australia. Harmful Algae. 39:134–145. doi: 10.1016/j.hal.2014.07.007
- Leaw CP, Tan TT, Lim HC, Teng ST, Yong HL, Smith KF, Rhodes L, Wolf M, Holland WC, Vandersea MW, et al. 2016. New scenario for speciation in the benthic dinoflagellate genus Coolia (Dinophyceae). Harmful Algae. 55:137–149. doi: 10.1016/j.hal.2016.02.010
- Litaker RW, Steidinger KA, Mason PL, Landsberg JH, Shields JD, Reece KS, Haas LW, Vogelbein WK, Vandersea MW, Kibler SR, et al. 2005. The reclassification of Pfiesteria shumwayae (Dinophyceae): Pseudopfiesteria, gen nov. Journal of Phycology. 41:643–651. doi: 10.1111/j.1529-8817.2005.00075.x
- Litaker RW, Vandersea MW, Faust MA, Kibler SR, Nau AW, Holland WC, Chinain M, Holmes MJ, Tester PA. 2010. Global distribution of ciguatera causing dinoflagellates in the genus Gambierdiscus. Toxicon. 56:711–730. doi: 10.1016/j.toxicon.2010.05.017
- MacKenzie A. 2014. The risk to New Zealand shellfish aquaculture from paralytic shellfish poisoning (PSP) toxins. New Zeal J Mar Freshwat Res. 48:430–465. doi: 10.1080/00288330.2014.911191
- MacKenzie AL, Selwood AI, McNabb P, Rhodes LL. 2011. Benthic dinoflagellate toxins in two warm-temperate estuaries: Rangaunu and Parengarenga Harbours, Northland, New Zealand. Harmful Algae. 10:559–566. doi: 10.1016/j.hal.2011.02.007
- MacKenzie LA. 2009. Risk evaluation of dredging and the potential for harmful algal bloom initiation in Whangarei Harbour. Prepared for Northland Regional Council. Cawthron Report No. 1584. 8p.
- MacKenzie L, Beuzenburg V, McNabb P, Holland P, Suzuki T, Neil T, Selwood A. 2004. Pectenotoxin and okadaic acid based toxin profiles in Dinophysis acuta and D. acuminata from New Zealand. Harmful Algae. 4:75–85. doi: 10.1016/j.hal.2003.12.001
- MacKenzie L, Holland P, McNabb P, Beuzenberg V, Selwood A, Suzuki T. 2002. Complex toxin profiles in phytoplankton and Greenshell mussels (Perna canaliculus), revealed by LC-MS/MS analysis. Toxicon. 409:1321–1330. doi: 10.1016/S0041-0101(02)00143-5
- MacKenzie L, White D, Oshima Y, Kapa J. 1996. The resting cyst and toxicity of Alexandrium ostenfeldii (Diniphyceae) in New Zealand. Phycologia. 35:148–155. doi: 10.2216/i0031-8884-35-2-148.1
- Mertens KN, Carbonell-Moore MC, Pospelova V, Head MJ, Highfield A, Schroeder D, Gu H, Andree KB, Fernández M, Yamaguchi A, et al. 2017. Pentaplacodinium saltonense gen. et sp. nov. (Dinophyceae) and its relationship to the cyst-defined genus Operculodinium and yessotoxin-producing Protoceratium reticulatum. Harmful Algae. 71:57–77. doi: 10.1016/j.hal.2017.12.003
- Ministry for the Environment. 2016. Climate change projections for New Zealand. Ministry for the Environment. 127 pp.
- Munday R, Murray S, Rhodes L, Larsson M, Harwood DT. 2017. Ciguatoxins and maitotoxins in extracts of 17 Gambierdiscus isolates from the South Pacific and their toxicity to mice by intraperitoneal and oral administration. Marine Drugs, accepted.
- Munday R, Quilliam MA, LeBlanc P, Lewis N, Gallant P, Sperker SA, Ewert SH, McKinnon SL. 2012. Investigations into the toxicology of spirolides, a group of marine phycotoxins. Toxins. 4:1–14. doi: 10.3390/toxins4010001
- Murray S, Daugbjerg N, Flo Jorgensen M, Rhodes L. 2004. Amphidinium revisited: II. Resolving species boundaries in the Amphidinium operculatum species complex (Dinophyceae), including the descriptions of Amphidinium trulla sp. Nov. and Amphidinium gibbosum comb. nov. Journal of Phycology. 40:366–382. doi: 10.1046/j.1529-8817.2004.03132.x
- Park T-G. 2006. Morphotaxonomy, genetic affinities and ecology of Australian and Antarctic populations of the potentially fish killing, heterotrophic dinoflagellates Cryptoperidiniopsis brodyi and Pfiesteria piscicida. PhD thesis, University of Tasmania, Australia.
- Park T-G, de Salas MF, Bolch CJS, Hallegraeff GM. 2007. Development of a real-time PCR probe for quantification of the heterotrophic dinoflagellate Cryptoperidiniopsis brodyi (Dinophyceae) in environmental samples. Applied and Environmental Microbiology. 73:2552–2560. doi: 10.1128/AEM.02389-06
- Parsons M, Aligizaki A, Bottein M-YD, Fraga S, Morton S, Penna A, Rhodes L. 2012. Gambierdiscus and Ostreopsis: reassessment of the state of knowledge of their taxonomy, geography, ecophysiology and toxicology. Harmful Algae. 14:107–129. doi: 10.1016/j.hal.2011.10.017
- Parsons ML, Brandt AL, Ellsworth A, Leynse AK, Rains LK, Anderson DM. 2017. Assessing the use of artificial substrates to monitor Gambierdiscus populations in the Florida Keys. Harmful Algae. 68:52–66. doi: 10.1016/j.hal.2017.07.007
- Rhodes L. 2011. World-wide occurrence of the toxic dinoflagellate genus Ostreopsis Schmidt. Toxicon. 57:400–407. doi: 10.1016/j.toxicon.2010.05.010
- Rhodes L, Gimenez Papiol G, Smith K, Harwood T. 2013a. Gambierdiscus cf. yasumotoi (Dinophyceae) isolated from New Zealand’s sub-tropical northern coastal waters. New Zealand Journal of Marine and Freshwater Research. 48:303–310. doi: 10.1080/00288330.2013.870220
- Rhodes L, Holland PT, McNabb PS, Selwood AI, Adamson JE, Quilliam ME. 2006a. Prorocentrum lima strains from New Zealand: DSP toxins profiles and uptake and conversion in Greenshell mussel. In: Henshilwood K, Deegan B, et al., editors. Molluscan Shellfish Safety. Proceedings of the 5th Int. Conf. on Molluscan Shellfish Safety, Galway, Ireland June 2004. The Marine Institute, Ireland, p. 235–241.
- Rhodes L, MacKenzie L, White D, Smith P. 1994. Movement of mussel spat within New Zealand: the risks of toxic microalgal introductions. Cawthron report No. 473.
- Rhodes L, Munday R. 2016. Harmful algae and their commercial implications. In: Chisti Y, Bux F, editors. Algae biotechnology: products and processes. Switzerland: p. 301–315.
- Rhodes L, Selwood A, McNabb P, Smith K. 2010a. Pinnatoxin in Rangaunu Harbour, Northland, New Zealand. In: Ho K-C, Qi YI, editors. Harmful Algae 2008. Proceedings. 13th International Conference on Harmful Algae, Hong Kong, China, Nov 2008. ISSHA and Environmental Pub. House Hong Kong; p. 151–154.
- Rhodes L, Smith K, Harwood T, Bedford C. 2014a. Novel and toxin-producing epiphytic dinoflagellates isolated from sub-tropical Raoul Island, Kermadec Islands group. New Zealand Journal of Marine and Freshwater Research. 48:594–599. doi: 10.1080/00288330.2014.963127
- Rhodes L, Smith KF, Moisan C. 2013b. Shifts and stasis in marine HAB monitoring in New Zealand. Environmental Science and Pollution Research. 20:6872–6877. doi: 10.1007/s11356-012-0898-9
- Rhodes L, Smith K, Selwood A, McNabb P, van Ginkel R, Holland P, Munday R. 2010b. Production of the cyclic imine, pinnatoxin, by a peridinoid dinoflagellate isolated from Northland, New Zealand. Harmful Algae. 9:384–389. doi: 10.1016/j.hal.2010.01.008
- Rhodes L, Smith K, Selwood A, McNabb P, Munday R, Suda S, Molenaar S, Hallegraeff G. 2011. Dinoflagellate Vulcanodinium rugosum Nézan et Chomérat newly identified as the causative organism of pinnatoxins in Australia, New Zealand and Japan. Phycologia. 50:624–628. doi: 10.2216/11-19.1
- Rhodes L, Suzuki T, Adamson, Mountfort D. 2001. Esterified okadaic acid in New Zealand strains of Prorocentrum lima. In: Hallegraeff GM, Blackburn SI, Bolch CJ, Lewis RJ, editors. Harmful Algal Blooms. IOC of UNESCO. p. 364–366.
- Rhodes LL, Adamson JE, Rublee P, Schaeffer E. 2006b. Geographic distribution of Pfiesteria piscicida and P. shumwayae (Pfiesteriaceae) in Tasman Bay and Canterbury, New Zealand (2002–2003). New Zealand Journal of Marine and Freshwater Research. 40:211–220. doi: 10.1080/00288330.2006.9517414
- Rhodes LL, Burkholder JM, Glasgow HB, Rublee PA, Allen C, Adamson JE. 2002. Pfiesteria shumwayae (Pfiesteriaceae) in New Zealand. New Zealand Journal of Marine and Freshwater Research. 36:621–630. doi: 10.1080/00288330.2002.9517117
- Rhodes LL, Smith KF, Gimenez Papiol G, Adamson JE, Harwood T, Munday R. 2014b. Epiphytic dinoflagellates in sub-tropical New Zealand, in particular the genus Coolia meunier. Harmful Algae. 34:36–41. doi: 10.1016/j.hal.2014.02.004
- Rhodes L, Smith L, Harwood T, Murray S, Biessy L, Argyle P, Munday R. 2017a. Is Gambierdiscus expanding its geographic range in the Pacific region? Harmful Algae News. 56:1–4.
- Rhodes L, Smith K, Harwood T, Selwood A, Argyle P, Bedford C, Munday R. 2015. Gambierdiscus and Ostreopsis from New Zealand, the Kermadec Islands and the Cook Islands and the risk of ciguatera fish poisoning in New Zealand. In: MacKenzie AL, editor. Marine and Freshwater Harmful Algae. Proceedings of the 16th International Conference on Harmful Algae, Wellington, New Zealand 27th–31st October 2014, p. 180–183.
- Rhodes L, Smith KF, Murray S, Harwood DT, Trnski T, Munday R. 2017b. The epiphytic genus Gambierdiscus (Dinophyceae) in the Kermadec Islands and Zealandia regions of the southwestern Pacific and the associated risk of ciguatera fish poisoning. Marine Drugs 15, on-line. doi: 10.3390/md15070219
- Rhodes LL, Smith KF, Verma A, Curley BG, Harwood DT, Kohli GS, Solomona D, Rongo T, Munday R, Murray S. 2017c. A new species of Gambierdiscus (Dinophyceae) from the south-west Pacific: Gambierdiscus honu sp. nov. Harmful Algae. 65:61–70. doi: 10.1016/j.hal.2017.04.010
- Rhodes, L.L., Smith, K.F., Verma, A., Murray, S., Harwood, D.T., Trnski, T. 2017d. The dinoflagellate genera Gambierdiscus and Ostreopsis from sub-tropical Raoul Island and North Meyer Island, Kermadec Islands. New Zealand Journal of Marine and Freshwater Research. doi:10.1080/00288330.2016.1270337.
- Rhodes LL, Syhre M. 1995. Okadaic acid production by New Zealand Prorocentrum lima isolate. New Zealand Journal of Marine and Freshwater Research. 29:367–370. doi: 10.1080/00288330.1995.9516671
- Rongo T, van Woesik R. 2012. Socioeconomic consequences of ciguatera poisoning in Rarotonga, southern Cook Islands. Harmful Algae. 20:927–100. doi: 10.1016/j.hal.2012.08.003
- Rublee P, Allen C, Schaefer E, Rhodes L, Adamson J, Lapworth C, Burkholder J, Glasgow H. 2004. Global distribution of toxic Pfiesteria complex species detected by PCR assay. In: Steidinger KA, Landsberg JH, Tomas CR, Vargo GA, editors. Harmful Algae 2002. Florida Fish and Wildlife Conservation Commission, Florida Institute of Oceanography, and IOC of UNESCO, p. 320–322.
- Rublee PA, Remington DL, Schaefer EF, Marshall MM. 2005. Detection of the dinozoans Pfiesteria piscicida and P. shumwayae: A review of detection methods and geographic distribution. Journal of Eukaryotic Microbiology. 52:83–89. doi: 10.1111/j.1550-7408.2005.05202007.x
- Salgado P, Fraga S, Rodríguez F, Bravo I. 2018. Benthic flattened cells of the phylogenetically related marine dinoflagellates Protoceratium reticulatum and Ceratocorys mariaovidorum (Gonyaulacales): a new type of cyst? Journal of Phycology. 54:138–149. doi: 10.1111/jpy.12611
- Satake M, Mackenzie L, Yasumoto T. 1997. Identification of Prorocentrum reticulatum as the biogenetic origin of Yessotoxin. Natural Toxins. 5:164–167. doi: 10.1002/19970504NT7
- Sato S, Nishimura T, Uehara K, Sakanari H, Tawong W, Hariganeya N, Smith K, Rhodes L, Yasumoto T, Taira Y, et al. 2011. Phylogeography of Ostreopsis along West Pacific Coast, with special reference to a novel clade from Japan. PloS ONE. 6(12):e27983. doi:10.1371/journal.pone.0027983.
- Selwood AI, Wilkins AL, Munday R, Shi F, Rhodes LL, Holland PT. 2013. Portimine: a bioactive metabolite from the benthic dinoflagellate Vulcanodinium rugosum. Tetrahedron Letters. 54:4705–4707. doi: 10.1016/j.tetlet.2013.06.098
- Shears NT, Ross PM. 2009. Blooms of benthic dinoflagellates of the genus Ostreopsis; an increasing and ecologically important phenomenon on temperate reefs in New Zealand and worldwide. Harmful Algae. 8:916–925. doi: 10.1016/j.hal.2009.05.003
- Smith KF, Biessy L, Argyle P, Trnski T, Halafihi T, Rhodes LL. 2017a. Molecular identification of Gambierdiscus and Fukuyoa (Dinophyceae) from environmental samples. Marine Drugs. 15, available on-line.
- Smith KF, Kohli G, Murray SA, Rhodes LL. 2017b. Assessment of the metabarcoding approach for community analysis of benthic-epiphytic dinoflagellates using mock communities. New Zealand Journal of Marine and Freshwater Research, accepted.
- Smith KF, Rhodes L, Harwood DT, Adamson J, Moisan C, Munday R, Tillmann U. 2016. Detection of Azadinium poporum in New Zealand: the use of molecular tools to assist with species isolations. Journal of Applied Phycology. 28:1125–1132. doi: 10.1007/s10811-015-0667-5
- Smith K, Rhodes L, Selwood A, Marfell M, Zeewoldt C. 2007. Massive Karenia mikimotoi bloom in Northland, New Zealand: use of traditional and molecular techniques for rapid identification of HAB species. Harmful Algae News (IOC Newsletter). 34:1–3.
- Sutton P, Chiswell S, Gorman R, Kennan S, Rickard G. 2012. Physical marine environment of the Kermadec Islands region. Science for Conservation 318 Department of Conservation, Wellington. 16 p.
- Taylor MD, MacKenzie LA. 2001. Delimitation survey of the toxic dinoflagellate Gymnodinium catenatum in New Zealand. Cawthron report No. 661, 32 pp.
- Tester PA, Kibler SR, Holland WC, Usup G, Vandersea MW, Leaw CP, Teen LP, Larsen J, Mohammad-Noor N, Faust MA, Litaker RW. 2014. Sampling harmful benthic dinoflagellates: comparison of artificial and natural substrate methods. Harmful Algae. 39:8–25. doi: 10.1016/j.hal.2014.06.009
- Tillmann U, Elbrächter M, Krock B, Uwe J, Cembella A. 2009. Azadinium spinosum gen. et sp. nov. (Dinophyceae) identified as a primary producer of azaspiracid toxins. European Journal of Phycology. 44:63–79. doi: 10.1080/09670260802578534
- Tillmann U, Gottschling M, Nézan E, Krock B, Bilien G. 2014. Morphological and molecular characterisation of three new Azadinium species (Amphidomataceae, Dinophyceae) from the Irminger Sea. Protist. 165:417–444. doi: 10.1016/j.protis.2014.04.004
- Trnski T, de Lange PJ. 2015. Introduction to the Kermadec Biodiscovery Expedition 2011. Bulletin of the Auckland Museum. 20:1–18. http://www.aucklandmuseum.com/research/pub/bulletin/20/1.