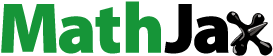
ABSTRACT
Recruiting King George whiting Sillaginodes punctatus were studied to assess the potential for food competition with permanent resident fish species in a nursery habitat. Marine migrant post larval S. punctatus (<60 mm TL) consumed primarily harpacticoid copepods and had high (>0.6) diet overlap with permanent resident fish species Favonigobius leteralis and Stigmatopora nigra. Food electivity index indicated that S. punctatus juveniles preferred harpacticoid copepods and amphipods, while juvenile Heteroclinus adelaide and Gymnapistes marmoratus migrating to the nursery habitat targeted larger prey such as amphipods. Preference for larger prey by H. adelaide and G. marmoratus species coupled with differences in prey composition in the stomach was due to mouth size and feeding habits, resulting in different food preferences to S. punctatus. The whiting showed an ontogenetic shift in diet with early settlers (>60 mm TL) consuming less copepods and more amphipods, while previous year recruits (>120 mm TL) consumed polychaete worms. This study indicates that competition for food resources between the new recruiting S. punctatus juveniles and permanent resident juveniles is reduced through differences in temporal and spatial feeding behaviours, mouth morphology, and ontogenetic shift in prey consumption.
Introduction
Seagrass habitats in estuaries form a highly productive ecosystem and are important nursery sites for many fish species (Able, Citation2005; Akin and Winemiller Citation2006). In southern Australia, seagrass beds can hold 40 times more benthic invertebrates than surrounding bare sand habitats (Bologna and Heck Citation2002). However, these habitats face considerable stress from climate change and anthropogenic activities such as urban development, eutrophication, pollution and recreational use (Bologna and Heck Citation2002; Bintz et al. Citation2003; Oviatt Citation2004; Davies et al. Citation2010; Hemraj et al. Citation2017). Loss of seagrass beds impact both food availability for fish species associated with this habitat, and biodiversity in the coastal environment (Heck et al. Citation2003). Understanding environmental factors and their influence on fish distribution and abundance is important to effectively manage fisheries and protect aquatic resources (Moyle and Cech Citation2004; Dinh et al. Citation2015).
Competitive interactions between teleosts can play an important role in habitat selection, foraging behaviour, growth, and survival and exerts influence on successful recruiting of juvenile fish to spawning stock (Munday et al. Citation2001; Mookerji et al. Citation2004; Polivka Citation2005). Food competition among fish species is generally greatest during early life stages and declines as fish grow (Ross, Citation1986; Dinh et al. Citation2017). The increased competition for resources is due to the influx of marine migrants and marine stragglers competing with permanent residents before migrant fish grow and move away from nursery areas (Najjar et al. Citation2000). It is well accepted that the success of one species can displace or outcompete another species if they cannot successfully partition resources (McDonald et al. Citation2001; Marks et al. Citation2011; Hossain, Ye, et al. Citation2017). In an inshore or estuarine environment, the success of permanent residents has the potential to impact on the success of arriving migrants via direct competition, whereas conversely, the successful recruitment of a migrant may place pressure on resources for permanent residents. Loss of suitable habitat may exacerbate competition among species as marine migrants compete for resources with permanent residents (Hobday et al., Citation1999). In addition, low juvenile recruitment of a species caused by the overfishing of breeding stocks can allow for an increase in abundance of less targeted fish species that share a similar diet. This could result in less available habitats and food resources being available for migrating juveniles (Hobday et al., Citation1999).
The Barker Inlet is the largest estuary in South Australia lying within the Gulf St Vincent. It is an inverse estuary due to high evaporation and low freshwater inflow. The Barker Inlet serves as a nursery ground for both post larvae and juveniles of the commercially important King George whiting (Sillaginodes punctatus). This nursery system provides seagrass beds of Zostera sp. and Heterozostera sp. that are vital for the successful recruitment of S. punctatus post larvae (Jones Citation1984; Fowler et al. Citation2002). However, susceptibility to global warming and anthropogenic activities such as storm water runoff and treated effluent has resulted in considerable changes to habitat structured and distribution in this region (Petrusevics Citation1993; Hossain, Hemraj, et al. Citation2017). In South Australia, S. punctatus spawn in the deep offshore waters off Kangaroo Island where ocean currents transport larvae northwards into the shallow waters of Gulf St Vincent (Fowler et al. Citation2002). Throughout winter and spring, S. punctatus larvae recruit into the shallow seagrass beds in protected bays (Fowler and Short Citation1996). The juvenile fish develop in these nursery areas for 1–2 years where they primarily feed on copepods, amphipods and polychaete worms (Connolly Citation1995) before moving out into the open waters of the gulfs. At approximately three years of age, S. punctatus begin their migration back towards the spawning grounds (Fowler et al. Citation2002). The recruitment success of S. punctatus post larvae that settle into seagrass beds likely drives the population dynamics of adult fish (Myers and Cadigan Citation1993). Despite competition links to survival of early life stages, no study on S. punctatus has evaluated the potential for trophic interactions with other seagrass dwelling fishes, or how S. punctatus recruitment might be impacted by habitat loss and competition for resources.
Within a nursery, there is a dynamic use of prey resources from different age classes and species, leading to complex trophic interactions. The objective of this study was to use juvenile S. punctatus of various ages throughout their temporary residency as a representative species to assess the potential food competition between recruiting fish and permanent resident fish over the period of ontogenetic diet shift. This study tests the hypothesis that permanent resident fishes compete for food with new settlers. The results would provide insight into the understanding of possible exclusion of recruiting fish by permanent dwelling fishes in the same nursery ground within a seagrass habitat.
Materials and methods
Water quality parameters and fish collection
Monthly sampling for post larvae of Sillaginodes punctatus and other resident fish was undertaken for a period of six months between June and November 2007. The sampling area was at King’s Beach, located at the northern end of the Barker Inlet (), which historically has provided the largest catches of juvenile S. punctatus (Jackson and Jones Citation1999). Sampling was conducted using a 5-m beach seine net with a 1-mm mesh. Three replicate hauls were conducted each month one hour either side of low tide between 1000 and 1200 h. For each haul, the net was towed perpendicular to the beach between two people over a patchy 20-m stretch of Zostera sp. and Heterozostera sp. seagrass beds where juvenile whiting were likely to reside. Net hauls were separated by 10–15 m. After completing each haul, the net was redirected towards the beach, pursed and lifted onto the beach. All fish were removed from the net and placed into a lethal dose of benzocaine prior to preservation in 10% buffered formalin. In order to compare diets of post larvae with larger-sized S. punctatus, a 60-m seine net with 2-m mesh wings and a bag mesh of 0.5 cm was deployed in January 2008 at the same location to capture early settlers (61-120 mm) and previous year recruits (>120 mm) for dietary comparisons. The sizes of S. punctatus used in this study were well below the size at maturity (30 cm) around 3 years old (Fowler et al. Citation2002). Temperature and salinity were measured in triplicate upon arrival at the study site in each month prior to fish and zooplankton sampling ().
Figure 1. Location of study site (King’s Beach, Barker Inlet) in relation to South Australian coastline (adapted from Cann et al. Citation2009).
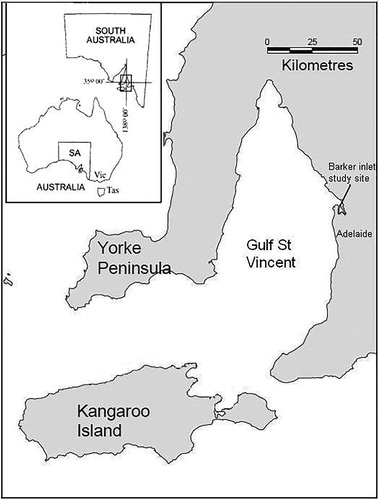
Table 1. Monthly variations of water temperature, salinity and fish abundance with the same fishing effort (60 m trawling distance) at King’s Beach, Barker Inlet, South Australia.
Collection and treatment of pelagic and benthic food sources
Pelagic zooplankton samples were collected monthly by towing a plankton net (30-cm open diameter, 100-µm mesh) fitted with a flow meter over the seagrass where fish were collected. As the water level was different at each sampling month, the zooplankton net was towed just above the level of the seagrass on all occasions. Each tow covered a length of 60 m and was replicated three times. The zooplankton samples were then transferred to storage containers and preserved in 3–5% neutrally buffered formalin. An exact volume (2.5% of the total) was subsampled in triplicate after thoroughly mixing the sample and then pipetting each subsample into a Sedgewick-Rafter counting cell under a microscope at 40–100× magnification.
To collect sediment samples of benthic fauna (e.g. copepod, amphipod, decapod, polychaete, nematode and molluscan), two replicated core samples were taken monthly at each of the three net haul sites, yielding a total of six cores per month. To collect samples, a 10.5 cm diameter piece of PVC pipe with a 0.25-mm mesh covering the top was pushed 5 mm into the sediment. A steel plate was then forced under the pipe and the corer was inverted to capture the sediment as described in Jenkins and Hamer (Citation2001). Most of the water was drained through the mesh and the sediment was placed into containers for processing in the laboratory. To facilitate sorting organisms from the sediment, all sediment samples were sieved through four mesh sizes: 2, 1, 0.5, 0.25 mm and the infauna and epifauna from each core were collected. Samples were placed into a counting wheel, which held 5–10 mL samples in a rotating circular trough. Infauna and epifauna were counted and identified on a dissecting microscope at 40× magnification. For the small mesh sizes where sediment remained, the samples were preserved in a formalin sucrose solution and stained with rose Bengal during counting and identification. Infauna and epifauna were taxonomically identified to the level of order.
Fish dietary analysis
In the laboratory, all fish captured in the net tows were identified and measured to the nearest millimetre using standard length (tip of the snout to caudal peduncle). Fish were dissected by removing the outer flesh and ribcage from one side of the fish and the stomach and intestine were excised. Only prey items within the oesophagus and stomach were analysed by removing the intestine from the posterior of the stomach with a scalpel. The stomachs were then placed in a drop of water and an incision was made so that the contents could be flushed out into the water. The water containing prey items was then drawn through a pipette and placed onto a Sedgewick counting chamber for identification and enumeration. The only exception to this was for pipefish which have no distinct stomach and consequently, the entire digestive tract was used. Depending on the total number of fish species collected, up to 20 stomachs of each species were analysed for diet comparison.
Percentage of prey occurrence in stomachs was calculated as the percent of a prey found in all stomachs of a particular species. Mouth gape for each fish was recorded by placing the fish under a microscope fitted with a digital camera (Moticam 2500), extending the mouth with a pair of forceps and taking a digital image. The Motic image program (Motic image plus 2.0) was then used to measure the mouth gape to the nearest 0.1 mm using a subsample of 10 fish per species.
Prey types identified in the fishes’ diets were used in calculating dietary characteristics. These prey types included copepods (cyclopoid, calanoid, harpacticoid porcelid harpacticoid, and nauplii), amphipods (gammarids and caprellids), tanaids, mysid shrimp, cumaceans, penaeid shrimp, polychaete worms, barnacle nauplii and plant material. Porcelid harpacticoids and caprellid amphipods were placed separately to other harpacticoids and gammarus amphipods using body morphology and structure (Karanovic Citation2008; Hayward and Ryland Citation2017).
Food preference
The Manly/Chesson index (Chesson Citation1983) was used to determine prey preference of the different fish species.where αi is the preference for prey type i ranging from 0 to 1, ni is the proportion of prey type i in the environment, ri is the proportion of i in the fish stomach, and m is the number of prey types in the environment.
To obtain a better comparison, dietary preference was further calculated as an electivity index (ε):where the αi is the preference value and m is the number of prey types. The values (εi) range from −1 to +1, where −1 indicates the absence of prey in stomachs and therefore suggests prey avoidance. Conversely, positive values suggest active selection of prey types. Results of zero indicate that there is no or little selection and that feeding is at random.
Dietary overlap
Diet overlap was calculated using Schoener’s overlap index (Schoener Citation1970).The index determines overlap (α), where Pij = the proportion of the ith resource (prey category) used by species j, and Pik = the proportion of the ith resource used by species k. Overlap index values range from 0 (no overlap) to 1.0 (complete overlap), Values 0–0.29 indicate low overlap, 0.3–0.59 indicate moderate, and ≥0.6 indicate high dietary overlap between the two fish species being compared (Langton Citation1982).
Feeding strategy
To determine if fish fed as specialists or generalists, Levins index (Krebs Citation1999) niche breadth was utilised:where Pi is the proportion of the ith prey in the diet of a fish species and the n is the number of prey groups. The average percentage of prey numbers (n) was used as the proportion (n%). Low values (B) indicate specialists and high values indicate generalists (Fjosne and Gjosaeter Citation1996). A standardised measure of this niche breadth BA is calculated as:
where the value of BA ranged from 0 (specialist) to 1 (generalist).
Statistical analysis and calculation
Due to data availability, the abundance of selected fish species (i.e. S. punctatus <60 mm, Stigmatopora nigra, F. lateralis, Gymnapistes marmoratus, and Heteroclinus adelaide) and prey types (i.e. harpacticoid copepods and amphipods) were used in correlation analysis. Spearman’s rank correlation analyses were used to determine the relationship between prey abundance and fish abundance using SPSS (version 18, IBM Corporation).
Results
During the study period from a cold and wet season (June) to hot and dry season (November), water temperature varied from 11.1°C to 20.8°C. A small increase in salinity was recorded from 38 to 40.5 ppt during the sampling period (). There was no significant correlation between total number of fish collected and temperature or salinity (r = 0.43, P = 0.40; r = 0.57, p = 0.23, respectively).
In benthic samples, prey species were dominated by harpacticoid copepods followed by gammarid amphipods and errant polychaete worms (). Over the sampling period, total abundance of harpacticoid copepods peaked in July and was lowest in October (). In comparison, the maximal abundance of gammarid amphipod occurred in September, but its lowest abundance was found in November. Errant polychaete abundance showed two peak periods in June and August.
Table 2. The abundance of benthic infauna and epifauna (mean ± SD, n = 6 per month) included in a sediment core area (86.6 cm2) at King’s Beach, Barker Inlet, South Australia (2007).
In pelagic samples, harpacticoid copepods and copepod nauplii were the most common pelagic prey available to fish living in the seagrass beds (). Cyclopoid copepods and polychaete larvae were the next abundant although polychaete larvae did not appear in the stomach contents of any fish species. Harpacticoid copepods and copepod nauplii recorded two peaks in abundance in September and November. Polychaete larvae showed two peaks with the first one in August and the second occurring in November. Mollusc veliger larvae were only found in October.
Table 3. Abundances of pelagic zooplankton (N m−3, mean ± SD) collected with a zooplankton trawl net at King’s Beach, Barker Inlet, South Australia (2007).
There was a negative trend between total benthic prey density (Arthropoda, Annelida, Mollusca), and total fish population (r = 0.83, p = 0.04, n = 6), although comparisons for all individual fish with total benthic prey density had no significant correlations except A. microstoma (r = 0.98, p < 0.001). No significant correlations were recorded between fish species and total pelagic prey density (r = 0.25, p = 0.62, n = 6). No significant correlations were observed between benthic or pelagic prey density and water temperature or salinity (p > 0.21). There was a negative but non-significant correlation between benthic prey abundance and temperature (r = −0.54) and salinity (r = −0.57) while there was a positive correlation between pelagic prey and temperature (r = 0.60) and salinity (r = 0.40).
Fish species composition
Throughout the study, 13 fish families were sampled from the seagrass beds (). The family of greatest abundance was Gobiidae (62%), with Favonigobius lateralis, accounting for 57% of all fish caught. The second most common family was Syngnathidae, with three species providing 15% of the total catch, of which Stigmatopora nigra was the most common at 14%. Both F. lateralis and S. nigra were the only species observed in all months and were the main permanent residents in this system. The greatest influx of fish to the region occurred in October and November with the third (Clinidae, Heteroclinus adelaide 8.4%), fourth (Sillaginidae S. punctatus 4.1%) and fifth (Scorpaenidae Gymnapistes marmoratus 2%) most abundant families recruiting throughout this period ().
Table 4. Monthly catch summary of fish species collected at King’s Beach, Barker Inlet, South Australia from June to November 2007.
Diet composition
Diet varied between S. punctatus of different size classes with the 20–60 mm class consuming primarily harpacticoid copepods (86%) and small gammarus amphipods (9%, ). Despite the low quantity of gammarus amphipods consumed, this prey item was consumed regularly with 80% of S. punctatus stomachs making it a very important food source (). The remainder of the diet consisted of calanoid and cyclopoid copepods, and the occasional worm or crustacean (mysid shrimp). The S. punctatus in the 61–120 mm class consumed primarily amphipods (84%) with worms and other crustaceans making up the remainder at low frequencies (). Stomachs of the largest S. punctatus size class (>120 mm) contained only polychaete worms (). The stomachs of F. lateralis contained a large proportion of harpacticoid copepods (88%) with a small percentage of the diets made up of amphipods (10%) and polychaete worms (2%, ). Amphipods were a frequently consumed prey item being present in 40% of stomachs (). The juvenile G. marmoratus and H. adelaide had similar diets which consisted mostly of crustaceans including amphipods (83%), tanaids, penaeid and mysid shrimp (<10%). A small percentage of their diets consisted of worms (<2% in both species) and copepods (7% in G. marmoratus and 11% in H. adelaide, ), but only occurred in a few of the stomachs (). The S. nigra primarily consumed copepods (59% cyclopoids, 35% harpacticoids, and 4% calanoids) with some incidents of small crustacean nauplii (0.7%, ) although the frequency of most prey items consumed was greater than 50% suggesting broad feeding habits (). The P. octolineatus contained a mix of copepods (15%) and amphipods (9%) but stomach contents mainly consisted of plant material (73%, ). The A. microstoma diets also consisted of a mix of calanoid, cyclopoid and harpacticoid copepods (93%) which were the most frequently consumed item ().
Figure 2. Individual prey types (%N) in the diet of the most common fish species captured in a seagrass bed in the Barker inlet from June 2007 to January 2008. *Other crustaceans include cumacean, tanaids and penaeid prawns grouped together.
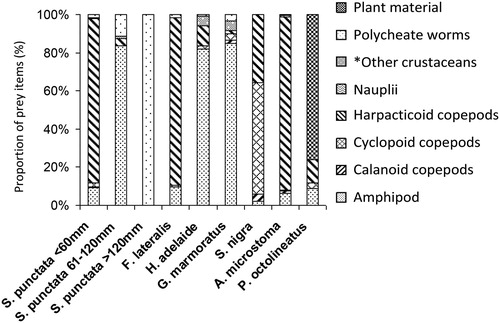
Table 5. The occurrence (%) of each prey in the stomach of fishes collected at King’s Beach, Barker Inlet, South Australia from June 2007 to January 2008.
Niche breadth, prey preference and diet overlap
According to the preference index, S. punctatus less than 60 mm and F. lateralis shared similar feeding behaviour showing active preference for harpacticoid copepods (εi = 0.5) and neutral selection for amphipods (εi = 0.05, −0.24, respectively). Food preference of both G. marmoratus and H. adelaide was positive for amphipods (εi > 0.5). With the exception of S. nigra, all fish species showed active avoidance of calanoid and cyclopoid copepods. All fish species exhibited selection against polychaete worms and large crustaceans (with the exception of S. punctatus >120 mm TL). G. marmoratus and H. adelaide were the only two fish species that selected against harpacticoid copepods (). Niche breadth was quite low for all species with the highest value observed in S. nigra (BA = 0.09, ). The G. marmoratus and H. adelaide with their similar diets had a slightly lower niche breadth (BA = 0.04) while S. punctatus >120 mm showed no dietary breadth consuming only one prey type (). Juvenile S. punctatus (<60 mm) had high dietary overlap (α ≥ 0.6) with F. lateralis and S. nigra while moderate (α = 0.3–0.59) overlap was observed with P. octolineatus (). Whiting <60 mm exhibited low (α < 0.029) overlap with H. adelaide, G. marmoratus, A. microstoma and also with the larger size of S. punctatus (61–120 mm) and high dietary overlap with H. adelaide and G. marmoratus, moderate overlap with A. microstoma and low overlap with all other species and S. punctatus size classes. The S. punctatus >120 mm had very little overlap with other species or other size classes of S. punctatus ().
Table 6. The range of fish length and mouth gape, mean stomach fullness rank and electivity index of fish caught between June 2007 and January 2008.
Table 7. Dietary overlap of King George whiting (Sillaginodes punctatus) at three size classes with other commonly occurring fishes in Barker inlet from June 2007 to January 2008.
Discussion
Fish species composition in Barker Inlet is similar to that reported in other studies of seagrass areas in temperate Australia (Crinall and Hindell Citation2004; Smith, Jenkins, et al. Citation2008). In the Barker Inlet habitat, fish abundance was relatively low compared to previous studies in the same area (Fowler and Short Citation1996; Jackson and Jones Citation1999). Habitat quality is often related to habitat selection, fish growth and survival (Nemerson and Able Citation2004). The seagrass beds at the sample site were covered by a thick coating of sediment which probably devalued the habitat quality and may have been responsible for the overall low fish abundance and low recruitment of S. punctatus into this historically productive nursery.
In a 10-year study, Jackson and Jones (Citation1999) provided a thorough historical analysis of fish assemblages in the Port River and specifically at King’s Beach where this study was conducted. King’s Beach previously had a large population of S. punctatus and was the most abundant species at this location, approximately 2.8 times higher than the next most abundant family (Mugilidae). In this study, S. punctatus was ranked fifth behind Gobiidae, Syngnathidae, Clinidae and Atherinidae. Fish assemblages appear to have changed considerably between the two studies especially in regard to the number of S. punctatus.
The timing of benthic settlement can be important where resources are limited because exploitative competition may lead to individuals depleting a food supply before the arrival of a competitor (Ward et al. Citation2006). Differences in recruitment timing can also be important by increasing niche separation between species (Golani, Citation1994). In this study, we found that S. punctatus were recruiting to the seagrass beds in small numbers from June to November. The arrival of juveniles of H. adelaide, G. marmoratus and F. lateralis occurred later in the sampling period from August to November generally peaking in abundance in November. The data of dietary composition in the stomach suggest that S. punctatus arriving to the seagrass earlier in the year may be advantageous to recruitment success as competition for resources is primarily with permanent residents and not the increased number of conspecifics and migrants arriving later in the year in the seagrass nursery ground.
Post larval fish in the 20–60 mm size class often consume copepod and amphipod prey items (Livingston Citation1982; Whitfield Citation1985; Schlacher and Wooldridge Citation1996). While there are similarities in the size of fish species, mouth morphology appears to be important in separating diet selection as is seen in other species occupying the same habitat (Hyndes et al. Citation1997; Linke et al. Citation2001). Fish species within the study site were of similar body size. S. punctatus and F. lateralis had a small mouth gape and selected for small prey such as copepods compared to H. adelaide and G. marmoratus which had relatively larger mouth gapes and actively selected for the larger amphipod prey. This morphological characteristic separated the diet of post larval S. punctatus <60 mm from other species and potentially reducing food competition.
The F. lateralis shared a diet consisting mainly of harpacticoid copepods with S. punctatus <60 mm, as indicated by the high overlap index close to 1 (i.e. complete diet overlap). Smith, Hindell, et al. (Citation2008) observed that most fish favoured the seaward side of the seagrass, whereas gobies Nesogobius sp. favoured the shoreward side of seagrass beds, suggesting spatial segregation with other seagrass-associated species. In this study, S. punctatus were located primarily over seagrass beds whereas the gobies, F. lateralis, could easily be observed being captured in the net on the shoreward side of the seagrass beds in shallower water. Therefore, despite the high food overlap, it is likely that these species are spatially separated to some extent. The macroalgae, Ulva australis, is often present at King’s Beach (Jackson and Jones Citation1999) and we found that F. lateralis often used this weed as shelter on the sand between the shore and the seagrass beds, whereas most other fish species were located in the seagrass beds. The difference in habitat selection along with preference for the most abundant prey species (harpacticoid copepods) may diminish food competition between recruiting S. punctatus <60 mm and permanent resident F. lateralis despite the high overlap recorded (Cabral et al. Citation2007). The increased shelter of Ulva australis for F. lateralis may explain the high abundance of this species despite the poor state of the seagrass beds. The preference for Ulva australis by F. lateralis may become advantageous allowing them to thrive in a sub-optimal seagrass habitat. Given the similarity in diets between F. lateralis and S. punctatus, loss of seagrass beds will likely reduce the habitat separation as S. punctatus seek other forms of shelter potentially contributing to an increase in competition for food resources. Generally, there are positive relationships observed between seagrass biomass and abundance of prey items such as harpacticoids and amphipods (Bologna and Heck Citation2002; Murphy et al. Citation2010). Loss of shelter may result in a loss of amphipods and force H. adelaide and G. marmoratus to exploit a less favoured prey items increasing the competition between seagrass associated fish.
The stomachs of S. nigra usually contain small amphipods and harpacticoid copepods (Howard and Koehn Citation1985). The S. nigra exhibited neutral selection for all families of copepods suggesting a diverse and generalised feeding habit. These data agree with Howard and Koehn (Citation1985) who observed that S. nigra feed on pelagic or epibenthic prey on the eelgrass. In contrast, the lack of pelagic prey in the diets of S. punctatus suggests that its feeding area is close to the benthic region, although in shallow environments such as the Barker Inlet study site, feeding on pelagic prey items can be done without much change to the fish’s position in the water column. Mobility is a factor that can potentially reduce competition within an ecosystem (Gibson and Ezzi Citation1987). The S. punctatus are not confined to seagrass beds often feeding over bare sand (Jenkins and Wheatley Citation1998), providing evidence that feeding interactions may also be avoided between recruiting S. punctatus and resident S. nigra via spatial differentiation.
Ontogenetic changes in the diet of S. punctatus also aided in dietary separation. The S. punctatus >60 mm consumed a greater proportion of amphipods and fewer copepods. In contrast, S. punctatus >120 mm had a diet consisting almost entirely of polychaete worms which were not consumed in large amounts by any other species or S. punctatus of different sizes. Changes in ontogenetic food preference are important in creating greater niche partitioning and reducing competition (Schellekens et al. Citation2010). The relatively large mouth gape of juvenile H. adelaide and G. marmoratus indicates that they target larger prey than newly settled S. punctatus and this would make them more competitive with early recruited than newly settled S. punctatus. The ontogenetic shift away from small prey items as S. punctatus grow also limits the time spend competing for food with small permanent resident species.
Resource partitioning of fish can also be enhanced by temporal differences in the period of peak feeding (Piet and Guruge Citation1997). In this study, the stomach contents of both G. marmoratus and H. adelaide were almost fully digested. Scorpaenids feed at both day and night (Hobson Citation1974), while weedfish Cristiceps australis move to areas of higher food abundance at night (Smith, Hindell, et al. Citation2008). In our study, sampling was conducted in the late morning, therefore the highly digested food in the stomachs of G. marmoratus and H. adelaide were probably ingested during the night. Juvenile S. punctatus are reported to only feed during the day (Connolly Citation1995), therefore competition with G. marmoratus or H. adelaide might be reduced through feeding at different times.
The low abundance of different prey species may contribute to a high degree of dietary overlap between fish species (Moyle and Cech Citation2004). In this study, niche breadth was low due to the relatively small amount of prey items available at high concentrations. Benthic prey was dominated by harpacticoid copepods and amphipods with only very small amounts of other prey items being recorded. The lack of prey items is reflected in the niche breadth index, suggesting that most of these fish species feed as specialists.
Potential competitors rarely reach the population densities that exceed resource supplies (Moyle and Cech Citation2004). Despite the significant correlation observed between total fish population and prey benthic prey density, predation on harpacticoid copepods is not thought to be the principal cause of variations in seasonal abundance of copepod prey (Gee Citation1989). Likewise, predation on meiofauna, in general, does not significantly reduce prey density due to the rapid reproductive rates of meiofauna (Coull Citation1999). Therefore, it is unlikely that this nursery is resource limited in terms of food, despite the observed correlation between fish and benthic prey density and overlap being observed between a few fish species. The overall low numbers of fish and the abundance of harpacticoid copepods and amphipods suggest that there should be an adequate supply of food in the system and that competitive exclusion by depletion of food resources is not at a level likely to have significant influence on post larval recruitment or permanent resident populations.
In conclusion, S. punctatus of different size classes show distinct ontogenetic shifts in diet preference reducing the period of competition for food between age classes of S. punctatus, and small permanent resident fish. This study supports the hypothesis that species living in close proximity show dietary overlap and food competition, but the main prey types targeted by all fish are abundant and competition for scarce resources is unlikely to affect the recruitment success of recruiting fish in this system. Competition is also currently reduced by differences in mouth morphology between species and by discrepancy in temporal and spatial uses of prey items, allowing a number of species to coexist without reducing resources to a limiting level. However, loss of seagrass habitats is likely to exacerbate the competitive interactions of fish within this nursery through decreased prey abundance and reduced spatial segregation.
Acknowledgements
Gratitude is extended to Anthony Fowler, Liang Song and Sophie Leterme, for their contribution to site selection, field sampling and discussion on this project. Animal ethics approval was obtained through Flinders University approval number E318.
Disclosure statement
No potential conflict of interest was reported by the authors.
Additional information
Funding
References
- Able KW. 2005. A re-examination of fish estuarine dependence: evidence for connectivity between estuarine and ocean habitats. Estuarine Coastal and Shelf Science. 64:5–17. doi: 10.1016/j.ecss.2005.02.002
- Akin S, Winemiller KO. 2006. Seasonal variation in food web composition and structure in a temperate tidal estuary. Estuaries and Coasts. 29:552–567. doi: 10.1007/BF02784282
- Bintz JC, Nixon SW, Buckley BA, Granger SL. 2003. Impacts of temperature and nutrients on coastal lagoon plant communities. Estuaries. 26:765–776. doi: 10.1007/BF02711987
- Bologna PAX, Heck KL. 2002. Impact of habitat edges on density and secondary production of seagrass-associated fauna. Estuaries. 25:1033–1044. doi: 10.1007/BF02691350
- Cabral HN, Vasconcelos R, Vinagre C, Franca S, Fonseca V, Maia A, Reis-Santos P, Lopes M, Ruano M, Campos J, et al. 2007. Relative importance of estuarine flatfish nurseries along the Portuguese coast. Journal of Sea Research. 57:209–217. doi: 10.1016/j.seares.2006.08.007
- Cann JH, Scardigno MF, Jago JB. 2009. Mangroves as an agent of rapid coastal change in a tidal-dominated environment, Gulf St Vincent, South Australia: implications for coastal management. Australian Journal of Earth Sciences. 56:927–938. doi: 10.1080/08120090903005386
- Chesson J. 1983. The estimation and analysis of preference and its relationship to foraging models. Ecology. 64:1297–1304. doi: 10.2307/1937838
- Connolly RM. 1995. Diet of juvenile King George whiting Sillaginodes punctata (Pisces: Sillaginidae) in the Barker inlet – Port River estuary, South Australia. Transactions of the Royal Society of South Australia. 119:191–198.
- Coull BC. 1999. Role of meiofauna in estuarine soft-bottom habitats. Australian Journal of Ecology. 24:327–343. doi: 10.1046/j.1442-9993.1999.00979.x
- Crinall SM, Hindell JS. 2004. Assessing the use of saltmarsh flats by fish in a temperate Australian embayment. Estuaries. 27:728–739. doi: 10.1007/BF02907656
- Davies PJ, Wright IA, Findlay SJ, Jonasson OJ, Burgin S. 2010. Impact of urban development on aquatic macroinvertebrates in south eastern Australia: degradation of in-stream habitats and comparison with non-urban streams. Aquatic Ecology. 44:685–700. doi: 10.1007/s10452-009-9307-y
- Dinh QM, Qin JG, Dittmann S, Dinh DT. 2017. Seasonal variation of food and feeding in burrowing goby Parapocryptes serperaster (Gobiidae) at different body sizes. Ichthyological Research. 64:179–189. doi: 10.1007/s10228-016-0553-4
- Dinh QM, Qin JG, Tran DD. 2015. Population and age structure of the goby Parapocryptes serperaster (Richardson, 1864; Gobiidae: Oxudercinae) in the Mekong Delta. Turkish Journal of Fisheries and Aquatic Sciences. 15:345–357. doi: 10.4194/1303-2712-v15_2_17
- Fjosne K, Gjosaeter J. 1996. Dietary composition and the potential of food competition between 0-group cod (Gadus morhua L) and some other fish species in the littoral zone. Ices Journal of Marine Science. 53:757–770. doi: 10.1006/jmsc.1996.0097
- Fowler AJ, Jones GK, McGarvey R. 2002. Characteristics and consequences of movement patterns of King George whiting (Perciformes: Sillaginodes punctata) in South Australia. Marine and Freshwater Research. 53:1055–1069. doi: 10.1071/MF02023
- Fowler AJ, Short DA. 1996. Temporal variation in the early life-history characteristics of the King George whiting (Sillaginodes punctata) from analysis of otilith microstructure. Marine and Freshwater Research. 47:809–818. doi: 10.1071/MF9960809
- Gee JM. 1989. An ecological and economic review of meiofauna as food for fish. Zoological Journal of Linnean Society. 96:243–261. doi: 10.1111/j.1096-3642.1989.tb01830.x
- Gibson RN, Ezzi IA. 1987. Feeding relationships of a demersal fish assemblage on the west coast of Scotland. Journal of Fish Biology. 31:55–69. doi: 10.1111/j.1095-8649.1987.tb05214.x
- Golani D. 1994. Niche separation between colonizing and indigenous Goatfish (Mullidae) along the Mediterranean coast of Israel. Journal of Fish Biology. 45:503–513. doi: 10.1111/j.1095-8649.1994.tb01332.x
- Hayward PJ, Ryland JS. 2017. Handbook of the marine fauna of North-West Europe. 2nd ed. Oxford: Oxford University Press.
- Heck KL, Hays G, Orth RJ. 2003. Critical evaluation of the nursery role hypothesis for seagrass meadows. Marine Ecology-Progress Series. 253:123–136. doi: 10.3354/meps253123
- Hemraj DA, Hossain A, Ye QF, Qin JG, Leterme SC. 2017. Anthropogenic shift of planktonic food web structure in a coastal lagoon by freshwater flow regulation. Scientific Reports. 7:44441. doi: 10.1038/srep44441
- Hobday DK, Officer RA, Parry GD. 1999. Changes to demersal fish communities in Port Phillip Bay, Australia, over two decades, 1970–91. Marine and Freshwater Research. 50:397–407. doi: 10.1071/MF97088
- Hobson ES. 1974. Feeding relationships of teleostean fishes on coral reefs in Kona, Hawaii. Fisheries Bulletin. 72:915–1031.
- Hossain MA, Hemraj DA, Ye QF, Qin JG. 2017. Diet overlap and resource partitioning among three forage fish species in Coorong, the largest inverse estuary in Australia. Environmental Biology of Fishes. 100:639–654. doi: 10.1007/s10641-017-0592-3
- Hossain MA, Ye QF, Leterme SC, Qin JG. 2017. Spatial and temporal changes of three prey fish assemblage structure in a hypersaline lagoon: the Coorong, South Australia. Marine and Freshwater Research. 68:282–292. doi: 10.1071/MF15212
- Howard RK, Koehn JD. 1985. Population dynamics and feeding ecology of pipefish (Sygnathidae) associated with eelgreass beds of Western Port, Victoria. Australian Journal of Marine and Freshwater Research. 36:361–370. doi: 10.1071/MF9850361
- Hyndes GA, Platell ME, Potter IC. 1997. Relationships between diet and body size, mouth morphology, habitat and movements of six sillaginid species in coastal waters: implications for resource partitioning. Marine Biology. 128:585–598. doi: 10.1007/s002270050125
- Jackson G, Jones GK. 1999. Spatial and temporal variation in nearshore fish and macroinvertebrate assemblages from a temperate Australian estuary over a decade. Marine Ecology-Progress Series. 182:253–268. doi: 10.3354/meps182253
- Jenkins GP, Hamer PA. 2001. Spatial variation in the use of seagrass and unvegetated habitats by post-settlement King George whiting (Percoidei: Sillaginidae) in relation to meiofaunal distribution and macrophyte structure. Marine Ecology-Progress Series. 224:219–229. doi: 10.3354/meps224219
- Jenkins GP, Wheatley MJ. 1998. The influence of habitat structure on nearshore fish assemblages in a southern Australian embayment: comparison of shallow seagrass, reef-algal and unvegetated sand habitats, with emphasis on their importance to recruitment. Journal of Experimental Marine Biology and Ecology. 221:147–172. doi: 10.1016/S0022-0981(97)00121-4
- Jones GK. 1984. The importance of Barker Inlet as an aquatic reserve: with special reference to fish species. Safic Magazine. 8(6):8–13.
- Karanovic T. 2008. Marine interstitial poecilostomatoida and cyclopoida (Copepoda) of Australia. Leiden: Brill.
- Krebs CJ. 1999. Ecological methodology. 2nd ed. Menlo Park (CA): Benjamin Cummings Press; 620 pp.
- Langton RW. 1982. Diet overlap between Atlantic cod, Gadus morhua, silver hake, Merluccius bilinearis, and fifteen other northwest Atlantic finfish. Fisheries Bulletin. 80:745–759.
- Linke TE, Platell ME, Potter IC. 2001. Factors influencing the partitioning of food resources among six fish species in a large embayment with juxtaposing bare sand and seagrass habitats. Journal of Experimental Marine Biology and Ecology. 266:193–217. doi: 10.1016/S0022-0981(01)00356-2
- Livingston RJ. 1982. Trophic organisation of fishes in a coastal seagrass system. Marine Ecology Progress Series. 7:1–12. doi: 10.3354/meps007001
- Marks JC, Williamson C, Hendrickson DA. 2011. Coupling stable isotope studies with food web manipulations to predict the effects of exotic fish: lessons from Cuatro Cienegas, Mexico. Aquatic Conservation-Marine and Freshwater Ecosystems. 21:317–323. doi: 10.1002/aqc.1199
- McDonald PS, Jensen GC, Armstrong DA. 2001. The competitive and predatory impacts of the nonindigenous crab Carcinus maenas (L.) on early benthic phase Dungeness crab Cancer magister Dana. Journal of Experimental Marine Biology and Ecology. 258:39–54. doi: 10.1016/S0022-0981(00)00344-0
- Mookerji N, Weng Z, Mazumder A. 2004. Food partitioning between coexisting Atlantic salmon and brook trout in the Sainte-Marguerite River ecosystem, Quebec. Journal of Fish Biology. 64:680–694. doi: 10.1111/j.1095-8649.2004.00333.x
- Moyle PB, Cech JJ. 2004. Fishes: an introduction to ichthyology. 5th ed. Upper Saddle River: Prentice Hall.
- Munday PL, Jones GP, Caley MJ. 2001. Interspecific competition and coexistence in a guild of coral-dwelling fishes. Ecology. 82:2177–2189. doi: 10.1890/0012-9658(2001)082[2177:ICACIA]2.0.CO;2
- Murphy HM, Jenkins GP, Hindell JS, Connolly RM. 2010. Response of fauna in seagrass to habitat edges, patch attributes and hydrodynamics. Austral Ecology. 35:535–543. doi: 10.1111/j.1442-9993.2009.02062.x
- Myers RA, Cadigan NG. 1993. Density dependent juvenile mortality in marine demersal fish. Canadian Journal of Fisheries and Aquatic Sciences. 50:1576–1590. doi: 10.1139/f93-179
- Najjar RG, Walker HA, Anderson PJ, Barron EJ, Bord RJ, Gibson JR, Kennedy VS, Knight CG, Megonigal JP, O'Connor R E, et al. 2000. The potential impacts of climate change on the mid-Atlantic coastal region. Climate Research. 14:219–233. doi: 10.3354/cr014219
- Nemerson DM, Able KW. 2004. Spatial patterns in diet and distribution of juveniles of four fish species in Delaware Bay marsh creeks: factors influencing fish abundance. Marine Ecology-Progress Series. 276:249–262. doi: 10.3354/meps276249
- Oviatt CA. 2004. The changing ecology of temperate coastal waters during a warming trend. Estuaries. 27:895–904. doi: 10.1007/BF02803416
- Petrusevics PM. 1993. SST fronts in inverse estuaries, South Australia-indicators of reduced gulf-shelf exchange. Australian Journal of Marine and Freshwater Research. 44:305–323. doi: 10.1071/MF9930305
- Piet GJ, Guruge W. 1997. Diel variation in feeding and vertical distribution of ten co-occurring fish species: consequences for resource partitioning. Environmental Biology of Fishes. 50:293–307. doi: 10.1023/A:1007390516552
- Polivka KM. 2005. Resource matching across habitats is limited by competition at patch scales in an estuarine-opportunist fish. Canadian Journal of Fisheries and Aquatic Sciences. 62:913–924. doi: 10.1139/f04-235
- Ross ST. 1986. Resource partitioning in fish assemblages – a review of field studies. Copeia. 1986:352–388. doi: 10.2307/1444996
- Schellekens T, de Roos AM, Persson L. 2010. Ontogenetic diet shifts result in niche partitioning between two consumer species irrespective of competitive abilities. American Naturalist. 176:625–637. doi: 10.1086/656488
- Schlacher TA, Wooldridge TH. 1996. Patterns of selective predation by juvenile, benthivorous fish on estuarine macrofauna. Marine Biology. 125:241–247. doi: 10.1007/BF00346304
- Schoener TW. 1970. Non-synchronous spatial overlap of lizards in patchy habitats. Ecology. 51:408–418. doi: 10.2307/1935376
- Smith TM, Hindell JS, Jenkins GP, Connolly RM. 2008. Edge effects on fish associated with seagrass and sand patches. Marine Ecology-Progress Series. 359:203–213. doi: 10.3354/meps07348
- Smith T, Jenkins G, Kemp J. 2008. Baywide monitoring key fishery species in seagrass beds sub-program. Fisheries Victoria; p. 17. Technical Report Series No. 7.
- Ward AJW, Webster MM, Hart PJB. 2006. Intraspecific food competition in fishes. Fish and Fisheries. 7:231–261. doi: 10.1111/j.1467-2979.2006.00224.x
- Whitfield AK. 1985. The role of zooplankton in the feeding ecology of fish from some southern African estuaries. South African Journal of Zoology. 20:166–171. doi: 10.1080/02541858.1985.11447930