ABSTRACT
The tetrasporophytic ‘Trailliella’ stage of the red algal genus Bonnemaisonia is reported for the first time from the Southern Hemisphere. Here we describe a bloom of the tetrasporophyte of B. hamifera from the mouth of the Waikouaiti River, Karitāne, South Island, where it occurred as thousands of free-floating tufts up to 1 cm wide in shallow water, accumulated in windrows on sand banks and beaches, and in the water column at depths of 10–12 m on the adjacent open coast. Gametophytic stages have not yet been found in New Zealand. The material collected to date is strictly vegetative, and reproduction occurs via fragmentation. The identity of the New Zealand samples as B. hamifera was confirmed by both morphology and rbcL sequencing. The abundance and floating dispersal of B. hamifera suggests that it is invasive and will become widely distributed in New Zealand.
Introduction
Here we report for the first time from the Southern Hemisphere the filamentous life-history stage of Bonnemaisonia hamifera Hariot (Citation1891), previously known as Trailliella intricata Batters (Citation1896). Bonnemaisonia has eight currently accepted species (Guiry and Guiry Citation2019) of which six have a native range in the North Pacific Ocean, and two species, B. australis Levring and B. spinescens Womersley, are endemic to Australia (Womersley Citation1996). Bonnemaisonia hamifera, Hariot, a native of Japan, has become widespread in the North Atlantic where it is common on temperate coasts of both Europe and North America (Mathieson and Dawes Citation2017; Guiry and Guiry Citation2019). The gametophytic stage was reported from South Africa (Stegenga et al. Citation1997) based on a single specimen, but the species has not been found subsequently in that country (John Bolton, personal communication). In the North Atlantic, where both gametophytic and sporophytic stages may be abundant, it is typically the tetrasporophyte that is most common and can become invasive (Svensson et al. Citation2013; Garbary et al. Citation2019). With the abundant population described here spanning seasons and habitats, the filamentous stage has invasive potential in New Zealand similar to other introduced species (Nelson et al. Citation2015).
Material and methods
From early March to mid-June 2019, observations and collections were made of a bloom of red algae in the Waikouaiti River estuary, Karitāne (45.64°S, 170.66°E), and on the adjacent outer coast at Te Awa Mokihi (Butterfly Bay), South Island (). Voucher specimens were pressed and deposited in WELT (Thiers Citation2019), with subsamples dried in silica gel. Morphological observations were carried out on fresh material or plants stained with 1% aniline blue acidified with 1% HCl and mounted in 40% Karo syrup (Karo, Englewood Cliffs, New Jersey USA). Photomicrographs were taken with an Olympus BX53 microscope (Olympus Corp., Tokyo, Japan) with an SC100 digital camera (Olympus Corp., Münster, Germany).
Figure 1. Aerial view of the mouth of the Waikouaiti River, Karitāne, north of Dunedin (−45.641023, 170.658784) showing drift accumulations of Bonnemaisonia estuarine shores with map inset of New Zealand.
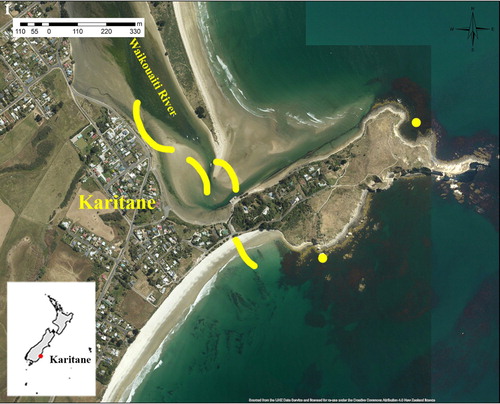
Sub-surface temperature data (December 2015–March 2019) was recorded every 10 min by SeaBird SBE37-SMP-ODO MicroCAT sensor moored at 2 m below mean low water (MLW) in Te Awa Mokihi (Butterfly Bay). Temperature data were used to calculate monthly summary statistics (means, standard deviations, maxima and minima) for surface waters in this area.
DNA was extracted from the subsamples dried in silica gel, with a modified CTAB protocol (Zuccarello and Lokhorst Citation2005). The plastid-encoded large subunit of the ribulose bisphosphate carboxylase/oxygenase gene (rbcL) was amplified using the primer combination F145–R898 and F762–R1442 (Kim et al. Citation2010). PCR products were cleaned using ExoSAP-IT reagent (Affymetrix, Santa Clara, CA, USA) and commercially sequenced (Macrogen Inc., Seoul, Korea). Sequences were aligned with those of other taxa of Bonnemaisoniaceae available in GenBank. New sequence data were deposited in Genbank (Accession Number MN067423).
Results
An aerial image () shows the distribution of patches of Bonnemaisonia stranded on sand bars and beaches in the estuary mouth at low tide. Accumulations were present in windrows and shallows of the estuary, with individual clumps forming fluffy balls up to c. 1 cm diameter (B–E). The clumps were entangled with thalli of Agarophyton chilense (C.J. Bird, McLachlan & E.C. Oliveira) Gurgel, J.N.Norris & Fredericq growing in the estuary. Material collected in June 2019 had associated small fragments of Ceramium spp., Centroceras clavulatum (C.Agardh) Montagne, Rhodophyllis acanthocarpa (Harvey) J.Agardh, Pterosiphonia sp., Antithamnion sp., and Jania sp.
Figure 2. A, Aerial image of the mouth of the Waikouaiti River. B, Drift windrows of Bonnemaisonia – March 2019. C–D, Dense accumulations of Bonnemaisonia in shallow water in estuary – March 2019. E, Bonnemaisonia bloom showing growth as balls/pompoms –1 April 2019.
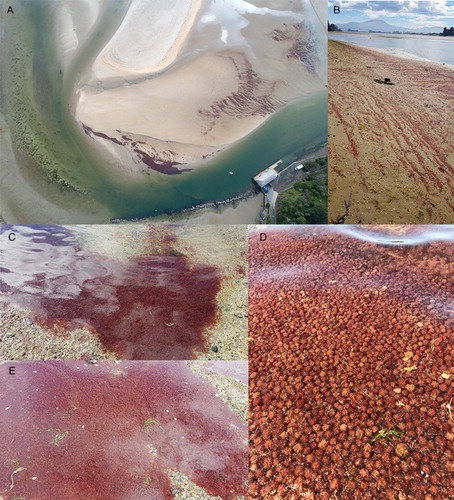
In the subtidal zone of coastal waters at Butterfly Bay () thalli were also abundant attached to benthic turf algae (A), and detached as free-floating clumps in populations of Macrocystis pyrifera (B–C) and Undaria pinnatifida (D). They were common throughout the water column of 10–12 m. Attached clumps of B. hamifera were likely the source of the free-floating material and explain the long duration of the bloom.
Figure 3. Subtidal habitats at Te Awa Mokihi (Butterfly Bay) in June 2019 with Bonnemaisonia hamifera. A, Numerous clumps settled on bottom associated with various turf and larger foliose algae. B, View of water column with numerous clumps of B. hamifera. C, Free-floating clumps of B. hamifera among Macrocystis forest. D, Non-native thallus of Undaria with B. hamifera.
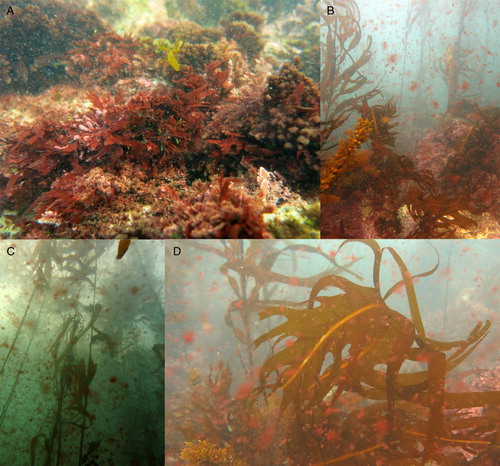
When examined in the laboratory, the often tight balls seen in the field were more irregular (A–B), similar to the free-floating material in the field (B,D). Microscopic examination of clumps that were teased apart had typical morphology of the tetrasporophytic phase of Bonnemaisonia hamifera, consisting of a mass of irregularly to alternately branched filaments (C–E). Filament diameters were uniform (c. 50 µm wide), except near apices that were slightly attenuated. Apical cells were dome-shaped. Primary filament cells were cylindrical and often associated with non-photosynthetic gland cells (F–G), with the typical cone-shape of the species, and these were attached laterally at the end of photosynthetic cells with little to conspicuous bulging of the outer cell wall. Aniline blue staining showed clearly defined pit connections between adjoining cells (arrows, H–I).
Figure 4. Field-collected samples of Bonnemaisonia hamifera. A-F, freshly collected; G-I, dead thalli. A–B, Clumps of B. hamifera showing irregular outlines. Scale bar = 1 cm. C–E, Filaments of B. hamifera with irregular to alternate branching. Scale bar = 100 µm. F–G, Portion of filaments with gland cells (arrows). Scale bar = 20 µm. H–I, Portions of filaments stained showing aniline blue with prominent, single rhodophysin granules in each cell, and pit connections between adjoining cells (arrows). Scale bar = 20 µm.
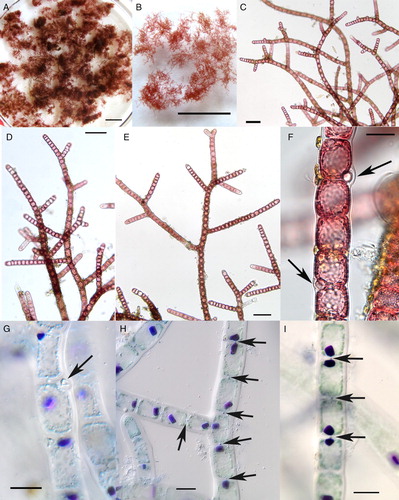
A curious cytological detail of the plastid-bearing cells was the presence of rhodospermin or rhodophysin granules that were single in each cell (G–I). In recently moribund material thalli appeared red, but microscopic inspection showed no evidence of pigments in the chloroplasts, i.e. they were bleached and the phycoerythrin pigment was aggregated into the rhodophysin granules (cf. Kylin Citation1956; Tandeau de Maesac Citation2003). Near filament apices these granules were round to irregular, with jagged edges. In older cells, they were mostly elongate with jagged ends and tended to aggregate near the pit connection of adjacent cells.
The rbcL sequence data obtained from New Zealand material were identical to GenBank entry KC130209.1, Bonnemaisonia hamifera, collected in Maine, USA, over 1353 bp. Three other sequences in GenBank attributed to B. hamifera (FJ195604.1, GQ252543.1 and GQ337066.1) differed from the New Zealand sequence by 2–4 bp over 1290-1353 bp (>99.6% sequence identity). A fourth sequence attributed to B. hamifera, U04044.1, collected from Brittany, France and sequenced prior to 1994, differs from the other four by more than 60 bp over 1353 and is likely misattributed.
Discussion
In New Zealand the red algal order Bonnemaisoniales is represented by eight species in the genera Asparagopsis, Delisea and Ptilonia. Species in these three genera were well described by Bonin and Hawkes (Citation1987, Citation1988a, Citation1988b) based on morphology of the gametophytic phases. Culture studies confirmed the presence of a trisiphonous ‘Falkenbergia’ stage in Asparagopsis armata Harvey and crustose stages in Delisea and Ptilonia. While the ‘Falkenbergia’ stage of Asparagopsis is known from field collections, the crustose tetrasporophytes of Delisea spp. and Ptilonia spp. have not been found in nature in New Zealand. Two additional genera of Bonnemaisoniales, Leptophyllis and Bonnemaisonia, were described by Womersley (Citation1996) from southern Australia. Leptophyllis has isomorphic gametophytes and tetrasporophytes; however, the tetrasporophytes of the two endemic Australian Bonnemaisonia species have not been characterised. Thus, the occurrence of a large population of a filamentous ‘Trailliella’ stage cannot be attributed to previously known New Zealand genera. Given that the rbcL sequences of the material from Waikouaiti were identical to a sequence of B. hamifera from the Atlantic Ocean, and the morphology of the material is identical to previous accounts from the North Atlantic and northwestern Pacific Oceans (e.g. Chihara Citation1961; Dixon and Irvine Citation1977; Verlaque et al. Citation2015; Mathieson and Dawes Citation2017), this filamentous material is clearly B. hamifera.
As a tetrasporophytic phase, the plants from Waikouaiti would be expected to form tetrasporangia. Since none of the material collected to date has been reproductive, despite the abundance and large size of the plants, this raises the possibility that the plants in New Zealand are sterile. Tetrasporangial formation in the filamentous phase of Bonnemaisonia hamifera is strongly associated with both temperature and daylength (Breeman Citation1988; Breeman et al. Citation1988). In northern Europe (Helgoland and Galway Bay) tetrasporangia were found to be produced in short days (8:16 h) and at relatively warm temperatures (11–17°C). In addition, differences in responses between the two populations in these studies suggest ecotypic differences. A subsequent study of Irish populations detailed how tides and time of day affect the response through changes in a threshold for irradiance and changes in microclimate (Breeman and Guiry Citation1989). Environmental conditions at the New Zealand site in March–June, when the population was still abundant, may be sufficient to induce sporulation [i.e. day:night between c. 13:11 (March) to 8.5:15.5 (June); mean sea surface temperature 15.5°C (March) and 13.5°C (June)]. Chen et al. (Citation1970) observed vegetative development of male gametophytic thalli from the filamentous phase after six months in culture. The occurrence of only male plants forming vegetatively was consistent with field observations on the Atlantic coast of Nova Scotia where only male plants were found (Chen et al. Citation1969).
Given the extent of the tetrasporophytic phase in the Waikouaiti estuary and on the nearby open coast on South Island, it would not be surprising to find gametophytic plants becoming established, even without the occurrence of female thalli and sexual reproduction. The abundance of the population at Waikouaiti and the ease of dispersal of the semi-buoyant colonies suggest that this phase will become widely distributed in New Zealand. While we became aware of the population only in March 2019, an aerial photograph taken in September 2018 () shows conspicuous accumulations of a red alga that was likely B. hamifera.
The source of the New Zealand population is uncertain. The species is likely to have been introduced to New Zealand by shipping. Otago Harbour is the nearest major port (where it has yet to be observed). Currents on the east coast of the South Island flow north to Waikouaiti from Dunedin. While native to the northwestern Pacific, B. hamifera was initially found in Europe from the south coast of England in 1890 (Batters Citation1896; see Dixon and Irvine Citation1977 for historical account), and it has since been regarded as an introduction from Japan (Guiry and Guiry Citation2019). It is now widespread on African and European coasts of the Mediterranean Sea (Verlaque et al. Citation2015), and from the Canary Islands through to northern Scandinavia in western Europe (Gollasch Citation2006). In the temperate western Atlantic Ocean it is known from Chesapeake Bay c. 35°N to Labrador 55°N and in the Caribbean Sea (Mathieson and Dawes Citation2017). Because of the more direct connection of New Zealand to the northeastern Pacific (i.e. Japan) it is tempting to speculate an origin from there rather than the North Atlantic. Regardless, more extensive geographic sampling of the genus and additional molecular markers (e.g. haplotypes, microsatellites) might enable resolution of the source of the New Zealand B. hamifera.
In Nova Scotia, eastern Canada, low intertidal rock pools can develop extensive growths of the filamentous stage that become entangled with other algae, especially Corallina officinalis Linnaeus and Mastocarpus stellatus (Stackhouse) Guiry. The collections made in June from Karitāne also showed Bonnemaisonia entangled with other species. As free-floating populations in Nova Scotia, they sometimes accumulate at the water’s edge to produce a beet-red ‘soup’ (Garbary, unpublished observations). Nuisance-scale blooms of the red alga Spyridia filamentosa have been reported in New Zealand, a species that, like B. hamifera, can be buoyant and is capable of propagating vegetatively (Nelson et al. Citation2015). Given the abundance of B. hamifera in the Waikouaiti River estuary and in nearby subtidal open coastal kelp beds, this species is becoming invasive and disrupting benthic turf-forming communities. The success of B. hamifera in Europe has been linked to the production of secondary metabolites. Svensson et al. (Citation2013) demonstrated that secondary metabolite of B. hamifera had strong allelopathic effects towards native competitors, particularly affecting the settlement of propagules, and suggested that this chemistry was important in the highly successful invasion of new ranges by B. hamifera. In 2017–2018, New Zealand experienced an unprecedented summer heat wave (Salinger et al. Citation2019), and we speculate that this and a continued series of heat waves detected though 2018 and 2019 (unpublished data) was responsible for the sudden abundance of B. hamifera.
Given the large population of B. hamifera at Karitāne, and its capacity for dispersal, the species may have an application in New Zealand. The red alga Asparagopsis taxiformis is in the same family as B. hamifera. The former has recently gained attention based on its ability to mitigate methane production in both cows and sheep when added in small amounts to their diet (Kinley et al. Citation2016; Li et al. Citation2018). This phenomenon is based on the presence of halogenentated compounds (Machado et al. Citation2016), that are also present in B. hamifera (McConnell and Fenical Citation1980). The free-floating nature of the plants is conducive to tank cultivation (Nash et al. Citation2005), similar to the terasporophyte of A. armata (‘Falkenbergia’) that has been grown using effluent from a fish farm in Portugal (Schuenhoff et al. Citation2006). We suggest that samples of tetrasporophytic B. hamifera from South Island be evaluated for its potential for reducing methane production by livestock following its use as a dietary supplement, and that land-based aquaculture be explored based on the extent of a positive response.
Conclusions
Tetrasporophytes of the red alga, Bonnemaisonia hamifera, that have become abundant at Karitāne, north of Dunedin, provide the first record of established populations in the Southern Hemisphere. The species was likely introduced by shipping into Otago Harbour. The irregularly branched filaments provide a growth form that becomes entangled with benthic seaweeds and then propagates through fragmentation. The highly buoyant filaments disperse through currents and wave action, and can readily initiate new populations. This combination of features, and the capacity of B. hamifera to grow in cold to warm temperate climates, suggest that this species has the potential to become invasive in intertidal and shallow subtidal zones through much of New Zealand.
Acknowledgements
We thank Becky Kerr and Kim Currie for additional field observations and temperature data, and Curt Pueschel for alerting us to the nature of the rhodophysin granules. Travel to New Zealand was funded by the Natural Sciences and Engineering Research Council, Discovery Grant to DJG. We acknowledge funding from SSIF – NIWA to the Coasts & Oceans Centre, Programme 2. Sub-surface temperature data was collected as part of the MBIE-funded Coastal Acidification: Rate, Impacts and Management (CARIM) project and provided by Kim Currie (NIWA).
Disclosure statement
No potential conflict of interest was reported by the authors.
ORCID
David J. Garbary http://orcid.org/0000-0001-5126-6608
Roberta D’Archino http://orcid.org/0000-0001-7025-3255
Wendy A. Nelson http://orcid.org/0000-0003-0014-5560
Judy. E. Sutherland http://orcid.org/0000-0002-1722-8218
Additional information
Funding
References
- Batters EAL. 1896. Some new British marine algae. J Bot. 34:6–11.
- Bonin DR, Hawkes MW. 1987. Systematics and life histories of New Zealand Bonnemaisoniaceae (Bonnemaisoniales, Rhodophyta): 1. The genus Asparagopsis. N Z J Bot. 25:577–590. doi: 10.1080/0028825X.1987.10410088
- Bonin DR, Hawkes MW. 1988a. Systematics and life histories of New Zealand Bonnemaisoniaceae (Bonnemaisoniales, Rhodophyta): 2. The genus Delisea. N Z J Bot 26:619–632. doi: 10.1080/0028825X.1988.10410664
- Bonin DR, Hawkes MW. 1988b. Systematics and life histories of New Zealand Bonnemaisoniaceae (Bonnemaisoniales, Rhodophyta): 3. The genus Ptilonia. N Z J Bot 26:633–644. doi: 10.1080/0028825X.1988.10410665
- Breeman AM. 1988. Relative importance of temperature and other factors in determining geographic boundaries of seaweeds: experimental and phenological evidence. Helgol Meeresunters. 42:199–241. doi: 10.1007/BF02366043
- Breeman AM, Guiry MD. 1989. Tidal influences on the photoperiodic induction of tetrasporogenesis in Bonnemaisonia hamifera (Rhodophyta). Mar Biol. 102:5–14. doi: 10.1007/BF00391318
- Breeman AM, Meulenhoff EJF, Guiry MD. 1988. Life history regulation and phenology of the red alga Bonnemaisonia hamifera. Helgol Meeresunters. 42:535–551. doi: 10.1007/BF02365625
- Chen LC-M, Edelstein T, McLachlan J. 1969. Bonnemaisonia hamifera in nature and in culture. J Phycol. 5:211–220. doi: 10.1111/j.1529-8817.1969.tb02605.x
- Chen LC-M, Edelstein T, McLachlan J. 1970. Vegetative development of the gametophyte of Bonnemaisonia hamifera from a filamentous state. Can J Bot. 48:523–525. doi: 10.1139/b70-073
- Chihara M. 1961. Life cycle of the bonnemaisoniaceous algae in Japan (1). Sci Rep Tokyo Kyoiku Daigaku, Sect B. 10(153):1–33.
- Dixon PS, Irvine LM. 1977. Seaweeds of the British Isles. Volume 1 Rhodophyta. Part 1 Introduction, Nemaliales, Gigartinales. London, UK: British Museum (Natural History).
- Garbary DJ, Bird CJ, Hymes B, Vandermeulen H. 2019. The marine macroalgae of Brier Island, Nova Scotia, Canada. Proc N S Inst Sci. 50:61–90.
- Gollasch S. 2006. Bonnemaisonia hamifera. DAISIE. http://www.europe-aliens.org/pdf/Bonnemaisonia_hamifera.pdf.
- Guiry MD, Guiry GM. 2019. AlgaeBase. World-wide electronic publication, National University of Ireland, Galway. [accessed 2019 Jun 7]. http://www.algaebase.org.
- Hariot P. 1891. Liste des algues marines rapportés de Yokosk (Japan) par M. le Dr. Savatier. Mém Soc Nat Sci Natur Math Cherbourg. 27:211–230.
- Kim MS, Kim SY, Nelson WA. 2010. Symphyocladia lithophila sp. nov. (Rhodomelaceae, Ceramiales), a new Korean red algal species based on morphology and rbcL sequences. Bot Mar. 53:233–241. doi: 10.1515/BOT.2010.031
- Kinley R, de Nys R, Vucko MJ, Machado L, Tompkins NW. 2016. The red macroalga Asparagopsis taxiformis is a potent natural antimethanogenic that reduces methane production during in vitro fermentation with rumen fluid. Anim Prod Sci. 56:282–289. doi: 10.1071/AN15576
- Kylin H. 1956. Die Gattungen der Rhodophyceen. Lund: C.W.K. Gleerups.
- Li XX, Norman HC, Kinley RD, Laurence M, Wilmot M, Bender H, de Nys R, Tompkins N. 2018. Asparagopsis taxiformis decreases enteric methane production from sheep. Anim Prod Sci. 58:681–688. doi: 10.1071/AN15883
- Machado L, Magnusson M, Paul NA, Kinley R, de Nys R, Tompkins N. 2016. Identification of bioactives from the red seaweed Asparagopsis taxiformis that promote antimenogenetic activity in vitro. J Appl Phycol. 28:3117–3126. doi: 10.1007/s10811-016-0830-7
- Mathieson AC, Dawes CJ. 2017. Seaweeds of the northwest Atlantic. Amherst, MA: University of Massachusetts Press.
- McConnell O, Fenical W. 1980. Halogen chemistry of the red alga Bonnemaisonia. Phytochemistry. 19:233–247. doi: 10.1016/S0031-9422(00)81967-6
- Nash R, Rindi F, Guiry MD. 2005. Optimum conditions for cultivation of the Trailliella phase of Bonnemaisonia hamifera Hariot (Bonnemaisoniales, Rhodophyta), a candidate species for secondary metabolite production. Bot Mar. 48:257–265. doi: 10.1515/BOT.2005.035
- Nelson WA, Neil KF, D’Archino R. 2015. When seaweeds go bad: an overview of outbreaks of nuisance quantities of marine macroalgae in New Zealand. N Z J Mar Fresh Res. 49:472–491. doi: 10.1080/00288330.2015.1064975
- Salinger MJ, Renwick J, Behrens E, Mullan AB, Diamond HJ, Sirguey P, Smith RO, Trought MCT, Alexander L, Cullen NJ, et al. 2019. The unprecedented coupled ocean-atmosphere summer heatwave in the New Zealand region 2017/18: drivers, mechanisms and impacts. Environ Res Lett. 14:044023. doi: 10.1088/1748-9326/ab012a
- Schuenhoff A, Mata L, Santos R. 2006. The tetrasporophyte of Asparagopsis armata as a novel seaweed biofilter. Aquaculture. 252:3–11. doi: 10.1016/j.aquaculture.2005.11.044
- Stegenga H, Bolton JJ, Anderson RJ. 1997. Seaweeds of the South African west coast. Cape Town: Bolus Herbarium, University of Cape Town.
- Svensson JR, Nylund GM, Cervin G, Toth GB, Pavia H. 2013. Novel chemical weapon of an exotic macroalga inhibits recruitment of native competitors in the invaded range. J Ecol. 101:140–148. doi: 10.1111/1365-2745.12028
- Tandeau de Maesac N. 2003. Phycobiliproteins and phycobilisomes: the early observations. Photosynthesis Res. 76:197–205.
- Thiers B. (continuously updated) Index Herbariorum: a global directory of public herbaria and associated staff. New York Botanical Garden’s Virtual Herbarium. http://sweetgum.nybg.org/ih/.
- Verlaque M, Ruitton S, Mineur F, Boudouresque C-F. 2015. CIESM atlas of exotic species in the Mediterranean. Vol. 4 macrophytes. Monaco: CIESM.
- Womersley HBS. 1996. The marine benthic flora of Southern Australia, part IIIB. Canberra: ABRS.
- Zuccarello GC, Lokhorst G. 2005. Molecular phylogeny of the genus Tribonema (Xanthophyceae) using rbcL gene sequence data: monophyly of morphologically simple algal species. Phycologia. 44:384–392. doi: 10.2216/0031-8884(2005)44[384:MPOTGT]2.0.CO;2