ABSTRACT
We analysed internal dispersal of zooplankton by waterbirds (endozoochory) in New Zealand, quantifying zooplankton eggs in faecal droppings collected at two lakes, Lake Rotoroa (Hamilton) and Lake Rotorua. Sixty-seven faecal droppings were collected from Mallard Ducks (20), Canada Geese (11), Greylag Geese (6), Black Swans (20) and Australian Coots (10). Fifty eggs were found, with a mean of 0.75 eggs per dropping, indicating that waterbirds consume zooplankton eggs, and that these pass through the digestive system. No significant difference was observed in the abundance of eggs among waterbird species, and no eggs hatched in the laboratory. Our results suggest that waterbird dispersal of zooplankton in New Zealand is occurring, but numbers being transported are low. Further, as non-native waterbirds such as mallard ducks and geese do not migrate in New Zealand to the extent they do elsewhere, they are likely not primary vectors for zooplankton dispersal.
Introduction
Waterbirds are important dispersal vectors for diapausing stages of invertebrates and plants externally on their feathers and plumage (ectozoochory) and internally through their digestive tract (endozoochory) (e.g. Green et al. Citation2023). The abundance, distribution and mobility of waterbirds make them ideal vectors allowing for short-distance (among adjoining lakes) and long-distance (up to thousands of kilometres) transport of invertebrate eggs (Freeland et al. Citation2000; Charalambidou and Santamaría Citation2002; Clausen et al. Citation2002). Different bird species have been observed to carry varying abundances and species of zooplankton, and diapausing eggs have different probabilities of hatching following passage through the gut.
Reviewing movement of organisms by waterbirds, Green et al. (Citation2023) noted that there is a heavy geographical bias in endozoochory studies, with most research having been undertaken in Europe and North America. In New Zealand, the presence of plant seeds in mallard duck faeces has been examined, with at least nine of the twelve different seeds recovered from 29 faecal samples by Bartel et al. (Citation2018) belonging to non-native plant species. Endozoochory by waterfowl of zooplankton has not been investigated in New Zealand, despite the recent, rapid spread among waterbodies of non-native zooplankton taxa such as Daphnia galeata, Daphnia pulex and Skistodiaptomus pallidus (Duggan et al. Citation2006; Duggan et al. Citation2012; Branford and Duggan Citation2017). We examined waterbird faeces for zooplankton diapausing stages transported internally via waterbirds (endozoochory) in New Zealand. We aimed to determine the number and viability of zooplankton eggs passing through the gut and whether the abundance and viability of eggs differ among waterbird species.
Methods
Faecal samples were collected from two North Island, New Zealand, lakes. Lake Rotorua has a maximum depth of 10.7 m and a catchment size of 42 400 ha, comprising pasture, exotic forest and urban area, including the city of Rotorua (Rutherford Citation1984). Lake Rotoroa, an urban lake in Hamilton, has a maximum depth of 6 m, and a catchment of 138 ha made up of urban residential and recreational reserves (Tanner et al. Citation1990). Both lakes are considered eutrophic (De Winton et al. Citation2002; Smith et al. Citation2016).
Two bird species were common at Lake Rotoroa, Mallard Ducks (Anas platyrhynchos) and Canada Goose (Branta canadensis), and three at Lake Rotorua, Greylag Goose (Anser anser), Black Swan (Cygnus atratus) and Australian Coot (Fulica atra australis), the latter a subspecies of the Eurasian Coot. These species represent typical bird communities dominating urban New Zealand lakes (e.g. Bartel et al. Citation2018; Gill and West Citation2018). Mallard Ducks are native to Europe, Asia and North America, and are widespread across New Zealand (Guillemain et al. Citation2020). In New Zealand there has been extensive hybridisation and introgression with the native grey duck, and it is difficult to discriminate between mallards, grey ducks, and hybrids (Guay et al. Citation2014; McDougall and Amundson Citation2017). Mallards are omnivorous, consuming aquatic plants and aquatic invertebrates (Charalambidou and Santamaría Citation2002). Canada Geese were introduced to New Zealand from North America (White Citation1986; Allan et al. Citation1995), and are entirely herbivorous, feeding mainly on terrestrial grasses and some aquatic plants (Conover Citation1991). Greylag Goose were introduced from Europe and inhabit low lying or open areas with waterbodies, feeding on roots, grain, insects and snails (Heather and Robertson Citation2000). Black Swan were introduced from Australia, though many individuals may have arrived naturally; these inhabit lowland lakes, coastal lakes, and selected estuaries, and are entirely herbivorous, feeding primarily on submerged aquatic freshwater plants (Heather and Robertson Citation2000). Australian Coot are self-introduced from Australia, establishing in 1957, and are found through most of New Zealand (Heather and Robertson Citation2000). Coots are primarily herbivorous, feeding on grass, algae and seeds, but also consume aquatic invertebrates and eggs (Heather and Robertson Citation2000).
Three accessible locations were selected at each lake where birds congregate on the shore (). Waterbird faeces was collected from Lake Rotoroa during late winter (30 July) and from Rotorua in early spring (29 September) 2021. The collection protocol for faeces followed Moreno et al. (Citation2019). Faeces were collected where waterbirds were currently inhabiting to ensure droppings were fresh. Individual droppings were collected with a wooden spatula and placed into airtight Falcon tubes (50 ml), one dropping per tube. Contamination was minimised by selecting samples not in contact with soils or other materials, or removing any part of the faeces in contact with soil or other material with a knife before storage. New spatulas were used for every faecal sample to prevent cross-contamination. Falcon tubes were wrapped with aluminium foil, transported to the laboratory in a cool box with ice, and stored in the fridge at 4°C for a minimum of four-weeks before processing with the aim to induce hatching. Twenty-litre near shore zooplankton samples were collected concurrently and preserved in ethanol (70% final concentration). Zooplankton were identified under a stereo dissecting microscope (Olympus SZ40) at approximately 30x magnification using standard taxonomic keys (e.g. Shiel Citation1995; Chapman et al. Citation2011).
Figure 1. Site locations where bird faecal samples were collected from Lake Rotoroa and Lake Rotorua and the respective positions of both lakes in the North Island.
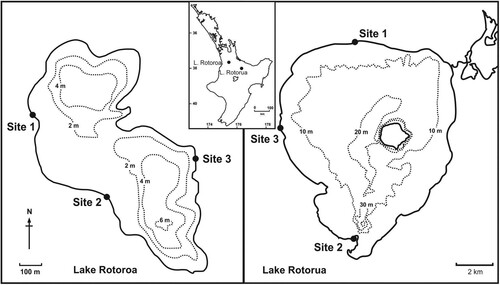
Following the storage period, each faecal sample was washed through a 40 µm sieve to remove fine sediment. Retained material was washed into a 50 ml centrifuge tube with a 1:1 weight:volume sugar:water solution (Parkes and Duggan Citation2012). Each sample was centrifuged (Hettich Zentrifugn: Universal 320 R) for 5 min at 54 g. The supernatant, containing organic material, was washed with water through a 40 µm sieve to remove the sugar solution. The organic material was poured into labelled Petri dishes using synthetic pond water (Hebert and Crease Citation1980). Each sample was immediately examined under a stereomicroscope and the abundance of eggs counted. Eggs found were placed into separate Petri dishes with synthetic pond water on a laboratory bench under ambient light to observe for hatching (sensu Green et al. Citation2008). Four Petri dishes with synthetic pond water acted as controls.
Fisher Exact tests were used to test for differences in the proportion of diapausing eggs among bird species. Kruskal–Wallis tests were used to determine whether differences occurred in abundances of diapausing eggs among bird species. Due to differences in bird species examined between lakes, and differences in collection timings, separate analyses were undertaken for each lake.
Results
Faecal droppings were collected from 67 waterbirds; 20 from Mallard Ducks, 11 from Canada Geese, 6 from Greylag Geese, 20 from Black Swans and 10 from Australian Coots. In total, 31 samples were collected from Lake Rotoroa and 36 from Lake Rotorua. Greylag Goose (18.7 g) and Canada Goose (15.3 g) had the highest mean faecal weights, followed by Black Swan (13.1 g), Australian Coot (11.7 g) and Mallard Duck (9.3 g) (). Faecal contents from all birds contained large amounts of plant material.
Table 1. Abundances and frequency of diapausing eggs from 67 faecal samples collected from waterbirds around Lake Rotorua (Greylag Goose, Black Swan and Australian Coot) and Lake Rotoroa (Mallard Duck and Canada Goose).
In total, 50 eggs were obtained from the 67 faecal droppings (0.75 eggs per faeces), with 22.4% of individual droppings carrying eggs. In Lake Rotorua, 8 eggs were found from 10 Australian Coot droppings (i.e. 0.8 diapausing eggs per faecal sample), 26 from 20 Black Swan (1.4), and 6 eggs from 6 Greylag Goose droppings (1.0; ). In Lake Rotoroa, 2 eggs were found from 11 Canada Goose (0.2) droppings and 8 eggs from 20 Mallard duck (0.4) droppings. The maximum number of eggs in any one dropping was 16, from a Black Swan. Eggs abundances per faecal sample was not significantly different between Mallard Ducks and Geese in Lake Rotoroa (Kruskal–Wallis test, H = 0.043; P = 0.836), or among Geese, Swans and Coot in Lake Rotorua (Kruskal–Wallis test, H = 0.757; P = 0.685). No significant differences were found between the number of eggs recorded in the faecal samples from Lake Rotoroa and Lake Rotorua irrespective of bird species (Kruskal–Wallis test, H = 0.313; P = 0.576).
From Lake Rotorua, the frequency of waterbird faeces containing eggs was 33% for Greylag Goose and 30% for Black Swan. From Lake Rotoroa, 20% of Mallard Duck droppings contained eggs, 18% of Canada Geese droppings, and 10% of Australian Coot faeces. No significant differences were found in the proportion of bird droppings containing diapausing eggs among the different waterbird species at each lake (Lake Rotorua 2 × 3 Fisher Exact test, P = 0.5179; Lake Rotoroa 2 × 2 Fisher Exact test, P = 0.7605).
No hatching was observed from the isolated eggs, and no zooplankton were observed in the controls. Eggs could not confidently be assigned to taxon. However, most eggs were oval shaped, measuring between 100 and 150 µm in length, and likely represented cladoceran eggs free of ephippia, or possibly non-diapausing (i.e. parthenogenetic) eggs. During sampling, Alona sp. and Chydorus sp. were found in concentrations >2 ind./L in Lake Rotoroa, and Bosmina meridionalis >15 ind./L. In Lake Rotorua, B. meridionalis was recorded in abundances >4 ind./L. Other eggs found were round and 160 µm in diameter, and were likely from calanoid copepods; both Lake Rotoroa (11.9 ind./L) and Lake Rotorua (2.6 ind./L) had moderate abundances of Calamoecia lucasi inhabiting the shallow littoral water during sampling. No diapausing eggs were observed resembling those from rotifers, despite high abundances in each lake during sampling (e.g. Duggan et al. Citation2002).
Discussion
We found evidence for zooplankton dispersal in New Zealand by endozoochory, with eggs found in the droppings of a variety of bird species. Eggs were recorded at low numbers and frequencies among birds, though similar to what has been recorded elsewhere. For example, a mean number of 0.9 cladoceran and rotifer diapausing eggs per dropping was recorded across several different waterbird species, including Mallard Ducks and Eurasian Coot, at lakes in Doñana National Park, Spain (Moreno et al. Citation2019). Eggs were recorded in 16% of droppings across a variety of bird species in South Africa by Reynolds and Cumming (Citation2015), while Charalambidou and Santamaría (Citation2002) found invertebrate diapausing eggs in 14% of bird droppings across three species collected in Denmark, England and France. Variability existed in diapausing egg abundances among bird species in our study. However, numbers were low across all species, ranging from 0.2 eggs per dropping in Canada Goose to 1.4 in Black Swan, while the frequency of droppings with eggs ranged from 10% for Australian Coot to 33% for Greylag Goose. Nevertheless, no significant differences were found among birds, through this was likely affected by low sample sizes for some bird species. Similar variability has been found elsewhere. For example, Moreno et al. (Citation2019), comparing between two lakes in Doñana National Park, found Eurasian Coots to carry 0.4 and 0.9 zooplankton eggs per dropping at each lake, while Mallard Ducks had 0.7 and 1.4 eggs per dropping. These Coot findings are similar to ours, having 0.8 eggs per dropping in our study, while our Mallard Ducks carried fewer, with 0.4 eggs per dropping. From droppings collected in England, France and Denmark, Charalambidou and Santamaría (Citation2002) found Eurasian Coot to carry 0.1 and Mallard Ducks 0.9 diapausing eggs per dropping on average, with 44% of Coot and 12% of Mallard Ducks carrying eggs in their droppings. In an extreme case, Green et al. (Citation2008) found 95% of Australian Coot to carry zooplankton eggs in an Australian arid zone wetland. We found only 10% of Coots to carry eggs, but 30% of Mallard Ducks. While our abundances in Mallard Duck droppings appear low compared to these studies, Figuerola et al. (Citation2003), also from Doñana National Park, found cladoceran eggs to be carried by mallard ducks at a rate of 0.2 eggs per dropping, while Eurasian Coot carried higher numbers, with 2.4 eggs per faeces. Overall, these results demonstrate that both the proportions of eggs per dropping, and the egg abundances, vary greatly among bird species, and among the same bird species in spatially, suggesting that the numbers and likelihood of carrying eggs have site specific influences. For example, Charalambidou and Santamaría (Citation2002), examining droppings from green winged teal, found 20% of birds collected in England, 13% in France and 6.5% in Denmark to carry eggs. As such, we might expect a variety of results when examining bird droppings in different sites even within New Zealand.
Several factors can influence the numbers of diapausing eggs within bird droppings. Firstly, waterbird species vary in diet. The faeces from all waterbird species in our study contained large amounts of plant material, much of which appeared to be terrestrial in origin, particularly grass. Nevertheless, ingestion of diapausing eggs likely occurred while feeding on aquatic vegetation. Following diapausing egg production, most will sink, though some can float and attach to plants (Brendonck and De Meester Citation2003). Consumption of eggs could have occurred through ingestion of live, swimming individuals. As zooplankton species vary in the timings that they produce diapausing eggs seasonally (Jarnagin et al. Citation2004), eggs of particular species are more likely to be consumed at some times than others. Temporal variability of diapausing eggs found in bird droppings has been observed by several authors (e.g. Figuerola et al. Citation2003; Moreno et al. Citation2019). Overall, our study represents a snapshot in time, and the numbers found in droppings are likely to vary seasonally. Finally, once ingested, diapausing eggs need to pass through the gut. For example, Charalambidou et al. (Citation2003) fed Bythotrephes eggs to mallard ducks, of which only 7% were retrieved.
No hatching of diapausing eggs from droppings was observed. Viability of diapausing eggs following passage through the gut has been observed to be highly variable among studies. For example, Jenkins and Underwood (Citation1998) examined droppings from Mallard Ducks from an Illinois, U.S.A., pond; similar to our study, no rotifers, copepods, ostracods or cladocerans were hatched from faecal samples. Of the 7% of Bythotrephes eggs retrieved from fed mallard ducks, Charalambidou et al. (Citation2003) found none could be induced to hatch. However, Moreno et al. (Citation2019) hatched a wide variety of rotifers and cladocerans from waterbird faeces from Doñana National Park. In the same area, Figuerola et al. (Citation2003) hatched cladocerans from 2 of 36 mallard ducks, while from Eurasian Coot, 34 eggs hatched from 85 droppings. As such, it is likely that New Zealand waterbirds will on some occasions carry viable diapausing eggs.
Viable eggs must be transported to, and be deposited in, water at a new site. In Europe, hundreds of thousands of birds move among water bodies, especially during autumn and spring migrations (Charalambidou et al. Citation2003). In New Zealand, however, such migrations are less important. For example, while most Mallard Duck populations in North America are highly migratory, exhibiting long seasonal migrations each spring and autumn, Mallard Ducks in New Zealand do not undertake regular directional and seasonal migrations. Recoveries of Mallard Ducks from banding studies in New Zealand show little movement from banding sites. For example, 87% of returns of wild Mallard Ducks have been found to occur within 80 km of ringing sites, with only 2.9% recovered more than 160 km distant (Balham and Miers Citation1959). Nevertheless, Bartel et al. (Citation2018), using radiotracking data of 248 mallard ducks, found maximum travel distances of 19 km within 48 h, and argued this could be significant for spread of plants, at least locally. This limited movement is similar for other waterfowl species in New Zealand also. For example, Canada Geese are less migratory in New Zealand than elsewhere, tending to be restricted to breeding and wintering habitats (White Citation1986). Nevertheless, while the species examined had low abundances of eggs overall, some Shag (Phalacrocorax spp.) species are widespread, mobile and feed on fish and invertebrates (Heather and Robertson Citation2000), which may make them ideal candidates for future study (e.g. van Leeuwen et al. Citation2017).
While the probability of dispersal by individual waterfowl is low, it may become significant when considering the numbers of birds moving between waterbodies (Charalambidou et al. Citation2003). The size of waterfowl populations moving among New Zealand lakes is unknown. However, Mallard Ducks are hunted at a rate of 500,000 per year (McDougall and Amundson Citation2017), indicating a large potential movement of individuals. Further, we have considered only taxa being carried internally, while it is known that birds may carry significant numbers of eggs externally (e.g. Reynolds and Cumming Citation2015). However, our study does not support waterfowl being a major factor in moving non-native Daphnia or copepods in New Zealand, particularly compared with human mediated vectors. For example, while non-native Daphnia are now found in New Zealand at high frequencies in accessible urban ponds and lakes where human traffic is frequent (Duggan et al. Citation2006; Duggan et al. Citation2012; Branford and Duggan Citation2017), farm ponds isolated from human visitation have relatively few established non-native Daphnia populations (Le Quesne et al. Citation2021). Parkes and Duggan (Citation2012) found for New Zealand constructed lakes (i.e. dams, infilled quarries and ornamental ponds) that zooplankton community composition was most greatly influenced by the number of water bodies within 20 km and the distance to nearest lake, rather than by trophic state, as was the case for natural lakes. This suggests that birds may be important for movement of zooplankton species over short distances in a New Zealand context, but not for broader spread.
Acknowledgements
We thank Andy Green and an anonymous referee for their comments on our manuscript.
Disclosure statement
No potential conflict of interest was reported by the author(s).
References
- Allan JR, Kirby JS, Feare CJ. 1995. The biology of Canada geese Branta canadensis in relation to the management of feral populations. Wildlife Biology. 1:129–143. doi:10.2981/wlb.1995.018.
- Balham RW, Miers KH. 1959. Mortality and survival of mallard and grey ducks banded in New Zealand. New Zealand Department of Internal Affairs Wildlife Publication. 5:56.
- Bartel RD, Sheppard JL, Lovas-Kiss Á, Green AJ. 2018. Endozoochory by mallard in New Zealand: what seeds are dispersed and how far? PeerJ. 6:e4811. doi:10.7717/peerj.4811.
- Branford SN, Duggan IC. 2017. Grass carp (Ctenopharyngodon idella) translocations, including hitchhiker introductions, alter zooplankton communities in receiving ponds. Marine and Freshwater Research. 68:2216–2227. doi:10.1071/MF17051.
- Brendonck L, De Meester L. 2003. Egg banks in freshwater zooplankton: evolutionary and ecological archives in the sediment. Hydrobiologia. 491:65–84. doi:10.1023/A:1024454905119.
- Chapman MA, Lewis MH, Winterbourn MJ. 2011. Guide to the freshwater crustacea of New Zealand. Christchurch: New Zealand Freshwater Sciences Society. 188.
- Charalambidou I, Ketelaars HA, Santamaría L. 2003. Endozoochory by ducks: influence of developmental stage of Bythotrephes diapause eggs on dispersal probability. Diversity and Distributions. 9:367–374. doi:10.1046/j.1472-4642.2003.00026.x.
- Charalambidou I, Santamaría L. 2002. Waterbirds as endozoochorous dispersers of aquatic organisms: a review of experimental evidence. Acta Oecologica. 23:165–176. doi:10.1016/S1146-609X(02)01148-7.
- Clausen P, Nolet BA, Fox A, Klaassen M. 2002. Long-distance endozoochorous dispersal of submerged macrophyte seeds by migratory waterbirds in northern Europe—a critical review of possibilities and limitations. Acta Oecologica. 23:191–203. doi:10.1016/S1146-609X(02)01150-5.
- Conover MR. 1991. Herbivory by Canada geese: diet selection and effect on lawns. Ecological Applications. 1:231–236. doi:10.2307/1941816.
- De Winton MD, Taumoepeau AT, Clayton JS. 2002. Fish effects on charophyte establishment in a shallow, eutrophic New Zealand lake. New Zealand Journal of Marine and Freshwater Research. 36:815–823. doi:10.1080/00288330.2002.9517134.
- Duggan IC, Green JD, Burger DF. 2006. First New Zealand records of three non-indigenous zooplankton species: Skistodiaptomus pallidus, Sinodiaptomus valkanovi, and Daphnia dentifera. New Zealand Journal of Marine and Freshwater Research. 40:561–569. doi:10.1080/00288330.2006.9517445.
- Duggan IC, Green JD, Shiel RJ. 2002. Rotifer resting egg densities in lakes of different trophic state, and their assessment using emergence and egg counts. Archiv fur Hydrobiologie. 153:409–420. doi:10.1127/archiv-hydrobiol/153/2002/409.
- Duggan IC, Robinson KV, Burns CW, Banks JC, Hogg ID. 2012. Identifying invertebrate invasions using morphological and molecular analyses: North American Daphnia ‘pulex’ in New Zealand fresh waters. Aquatic Invasions. 7:585–590. doi:10.3391/ai.2012.7.4.015.
- Figuerola J, Green AJ, Santamaría L. 2003. Passive internal transport of aquatic organisms by waterfowl in Doñana, south-west Spain. Global Ecology and Biogeography. 12:427–436. doi:10.1046/j.1466-822X.2003.00043.x.
- Freeland JR, Romualdi C, Okamura B. 2000. Gene flow and genetic diversity: a comparison of freshwater bryozoan populations in Europe and North America. Heredity. 85:498–508. doi:10.1046/j.1365-2540.2000.00780.x.
- Gill RJ, West RC. 2018. High densities of water-birds at two New Zealand fresh-water urban lakes. Notornis. 65:84–91.
- Green AJ, Jenkins KM, Bell D, Morris PJ, Kingsford RT. 2008. The potential role of waterbirds in dispersing invertebrates and plants in arid Australia. Freshwater Biology. 53:380–392.
- Green AJ, Lovas-Kiss Á, Reynolds C, Sebastián-González E, Silva GG, van Leeuwen CHA, Wilkinson DM. 2023. Dispersal of aquatic and terrestrial organisms by waterbirds: a review of current knowledge and future priorities. Freshwater Biology. 68:173–190. doi:10.1111/fwb.14038.
- Guay PJ, Taysom A, Robinson R, Tracey JP. 2014. Hybridization between the mallard and native dabbling ducks: causes, consequences and management. Pacific Conservation Biology. 20:41–47. doi:10.1071/PC140041.
- Guillemain M, Söderquist P, Champagnon J, Elmberg J. 2020. Mallard (Anas platyrhynchos Linnaeus, 1758). In: Downs CT, Hart LA, editors. Invasive birds: Global trends and impacts. Wallingford: CABI International; p. 194–199.
- Heather BD, Robertson HA. 2000. The field guide to the birds of New Zealand. Auckland: Penguin Books.
- Hebert PD, Crease TJ. 1980. Clonal coexistence in Daphnia pulex (Leydig): another planktonic paradox. Science. 207:1363–1365. doi:10.1126/science.207.4437.1363.
- Jarnagin ST, Kerfoot WC, Swan BK. 2004. Zooplankton life cycles: direct documentation of pelagic births and deaths relative to diapausing egg production. Limnology and Oceanography. 49:1317–1332. doi:10.4319/lo.2004.49.4_part_2.1317.
- Jenkins DG, Underwood MO. 1998. Zooplankton may not disperse readily in wind, rain or waterfowl. Hydrobiologia. 387:15–21. doi:10.1023/A:1017080029317.
- Le Quesne KS, Özkundakci D, Duggan IC. 2021. Life on the farm: Are zooplankton communities in natural ponds and constructed dams the same? Marine and Freshwater Research. 72:330–340. doi:10.1071/MF20030.
- McDougall MB, Amundson CL. 2017. Harvest dynamics and annual survival of mallards and grey ducks. The Journal of Wildlife Management. 81:449–460. doi:10.1002/jwmg.21213.
- Moreno E, Pérez-Martínez C, Conde-Porcuna J. 2019. Dispersal of rotifers and cladocerans by waterbirds: seasonal changes and hatching success. Hydrobiologia. 834:145–162. doi:10.1007/s10750-019-3919-6.
- Parkes SM, Duggan IC. 2012. Are zooplankton invasions in constructed waters facilitated by simple communities? Diversity and Distributions. 18:1199–1210. doi:10.1111/j.1472-4642.2012.00913.x.
- Reynolds C, Cumming GS. 2015. The role of waterbirds in the dispersal of freshwater Cladocera and Bryozoa in southern Africa. African Zoology. 50:307–311. doi:10.1080/15627020.2015.1108164.
- Rutherford J. 1984. Trends in Lake Rotorua water quality. New Zealand Journal of Marine and Freshwater Research. 18:355–365. doi:10.1080/00288330.1984.9516056.
- Shiel RJ. 1995. A guide to identification of rotifers, cladocerans and copepods from Australian inland waters. Cooperative research centre for freshwater ecology. Albury, New South Wales: Murray-Darling Freshwater Research Centre.
- Smith VH, Wood SA, McBride CG, Atalah J, Hamilton DP, Abell J. 2016. Phosphorus and nitrogen loading restraints are essential for successful eutrophication control of Lake Rotorua, New Zealand. Inland Waters. 6:273–283. doi:10.5268/IW-6.2.998.
- Tanner CC, Clayton JS, Coffey BT. 1990. Submerged-vegetation changes in Lake Rotoroa (Hamilton, New Zealand) related to herbicide treatment and invasion by Egeria densa. New Zealand Journal of Marine and Freshwater Research. 24:45–57. doi:10.1080/00288330.1990.9516401.
- van Leeuwen CH, Lovas-Kiss Á, Ovegård M, Green AJ. 2017. Great cormorants reveal overlooked secondary dispersal of plants and invertebrates by piscivorous waterbirds. Biology Letters. 13:20170406. doi:10.1098/rsbl.2017.0406.
- White EG. 1986. Canada geese in New Zealand. Information Paper No. 4, Centre for Resource Management, Lincoln College, Lincoln. 40 p.