Abstract
The modernization of nuclear power plants will require an advanced concept of operations, involving an integrated set of tightly coupled systems in which all stakeholders act in a coordinated manner. For this modernization effort to be enabled, we developed a human and organizational factors approach based on a broad sociotechnical framework. Starting from core human factors principles, we conducted a literature review of the methods and approaches relevant to the modernization problem. These included not only core disciplines such as cognitive systems engineering, systems theoretic accident modeling and processes, human systems integration, resilience engineering, and macroergonomics but also related topics of safety culture and organizational change. From this literature, we developed a conceptual framework centered around the work system with its four interacting components: people, technology, process, and governance. In an effective work system, these four components are jointly optimized according to three systems criteria: efficiency, effectiveness, and safety. System failure may result from excessive emphasis on any one criterion. The actual work of attaining joint optimization in a given work system can be accomplished by utilizing three high-level functions: knowledge elicitation, knowledge representation, and cross-functional integration. We illustrated the utility of this approach by applying it to practical problems and case studies.
I. PATHWAY FOR DIGITAL TRANSFORMATION
The Plant Modernization Pathway of the U.S. Department of Energy Light Water Reactor Sustainability (LWRS) Program contains a strategic action planCitation1 that lays the groundwork for a digital transformation of the nuclear industry, embodying an advanced concept of operations with an end-point vision as follows: “… the digital infrastructure for a nuclear plant must be designed as an integrated set of systems that together enable a technology centric operating model” (see ). Designing and operating such integrated systems will, of course, require new, automated technologies. In addition, a new way of working, both in the design and operational phases, will be required. A sustained commitment from top management in terms of visible priorities and goals must percolate downward to the level of systems engineering in design and operations. For this effort to succeed, a strict discipline is required for the integration of multiple tightly coupled functions to ensure that all stakeholders in the systems engineering process are participating in a coordinated manner.
Fig. 1. Technology-centric plant operations model.Citation1
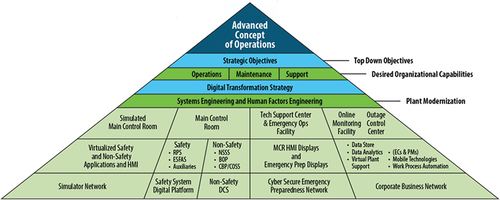
More recent work by Hunton and and EnglandCitation2 has provided a compelling business case against the like-for-like replacement strategy of individual instrumentation and control (I&C) systems. They found that the cost to maintain systems in their current form is economically unsustainable. This seems to be primarily due to the cost of maintaining obsolescent components, which continues to increase over time at an exponential rate.
Joe et al.Citation3 have extended the model in by providing a framework for a synthesized approach to research and development supporting the Plant Modernization Pathway.
As indicated in , the high-level objectives focus on modernization solutions within a sustainable business model that will achieve the high-level goals of extending life and improving performance of the existing fleet through modernization techniques and improved processes. Within the Pathway, four distinct research areas are identified: I&C (Digital) Architecture, Data Architecture and Analysis, Human and Technology Integration, and Integrated Operation for Nuclear. The third of these areas, i.e., Human and Technology Integration, has the responsibility integral to a systems engineering approach for the synthesis of all of these areas into a more integrated and organized set of solutions. This is the primary focus of the current paper.
Fig. 2. Conceptual overview of the LWRS Plant Modernization Pathway, with its objectives and goals highlighted.Citation3
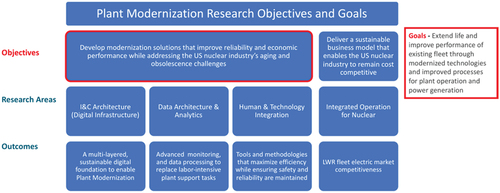
(CitationRef. 4) depicts a more detailed and refined set of potential strategies for plant modernization. Two separate but related aspects of the strategy are indicated. Capability Development (top half of ) consists of a planning process that focuses the transformation on those core capabilities that truly define value in the eyes of the ultimate customer. Capability involves the synthesis of four dimensions: people, technology, process, and governance (PTPG), with respect to resources needed, resources on hand, and resources that need to be developed. From an analytic perspective, capability starts at the individual function level, with functions becoming successively more integrated at higher levels. From a concept of operations perspective, however, the starting point is the highest level (stack model) one can achieve, applying a set of basic principles that reflect the end-state vision and effectively propagate downward. The bottom of reflects components of the work reduction focus, which will be systematically applied.
Fig. 3. A model of integrated operations merging capability development and work reduction strategies.Citation4
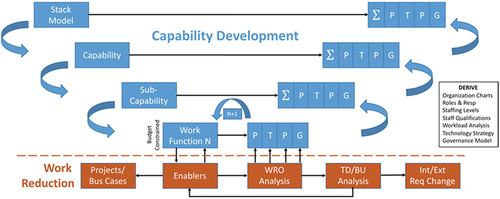
To enable the coordination required by the models depicted in , we developed the human and organizational factors approach to nuclear modernization described in detail in CitationRef. 5. The remainder of this paper discusses findings from that report. We must emphasize that there are many excellent sources of human factors engineering (HFE) guidance in the nuclear energy domain. These include CitationRefs. 6 through 9. We do not attempt to replace or modify these sources. Rather, the goal is to supplement this guidance by identifying methods to achieve the integration, consensus, and coordination called for in these publications.
II. LITERATURE REVIEW
Building on a traditional HFE foundation, we employed a human and organizational factors approach to the broad area of sociotechnical systems relevant to nuclear modernization. This approach is based on a literature review focused on the tools and methods that might be applicable. This review was constrained by limiting consideration to those methods for which there was evidence of active communities of practice in active engagement solving real-world problems.
Historically, the concept of the sociotechnical system was established to stress the reciprocal interrelationship between humans and machines and to foster the program of shaping both the technical and social conditions of work in such a way that efficiency and humanity would not contradict each other any longer.Citation10
The goal of the sociotechnical systems theory, as applied to systems design, is the joint optimization of social-organizational and technical subsystems. Additionally, an argument from the domain of resilience engineering describes the interdependence of three system performance criteria: effectiveness (accomplishment of mission), efficiency (optimization of resources), and safety (avoidance of injury or damage). Excessive emphasis on any one criterion at the expense of the others is likely, in the long run, to result in overall system failure.Citation11,Citation12
Sociotechnical systems theory provides a pathway to these goals and is foundational for several core disciplines within the HFE community, including macroergonomics, cognitive systems engineering (CSE), macrocognition/cognitive task analysis, and human systems integration.Citation13 Out of these core disciplines has emerged the “toolkit” of methods and techniques described in the next section of this paper. [Methods and techniques associated with these disciplines that will be referred to later are included in brackets.]
II.A. The Core Sociotechnical Disciplines
Macroergonomics, as its name suggests, was an expansion of traditional human factors and ergonomics to include social and organizational issues. The macroergonomic concept of a work systemCitation14 including the interaction of the social, technical, and organizational components has, as modified by the PTPG framework above, become our basic unit of analysis.
Cognitive systems engineering is a form of systems engineering and, as such, requires understanding and description at multiple levels of analysis. Several subdisciplines of CSE are considered. They include cognitive work analysis [Work Domain Analysis], which had its origins in nuclear engineeringCitation15; resilience engineering, which emerged from the postaccident analysis of the Columbia shuttle disasterCitation11; and System Theoretical Accident Model and ProcessCitation16 (STAMP), which has its origins in each of the previous developments. System Theoretic Process and Analysis [STPA] is a practical hazard analysis methodology based on STAMP.
Macrocognition/Cognitive Task Analysis is described as the collection of cognitive processes and functions that characterize how people think in natural settings.Citation17,Citation18 It is derived from the seminal work of Klein on naturalistic decision making.Citation19 A particular focus of this approach is the deep respect for the expertise of the subjects of their analysis as well as the recognition that this expertise is often distributed across multiple actors [Concept Maps, Structured Interviews, Consensus].
Human systems integration emerged within the context of the acquisition of large military and transportation systems and is simultaneously a high-level conceptual model for systems design and a formal U.S. Department of Defense requirement.Citation20 Although systems engineering and management theory have usually considered the interaction among people, technology, and organization to describe the top level of any complex system, it is through human systems integration that the most dramatic organizational benefits (in terms of increased performance and reduced costs and risk) can be achieved. These insights are reflected in the USS Zumwalt case study presented in CitationRef. 5.
II.B. Safety Culture and Organizational Change
The separate topics of safety culture and organizational change are not specifically included in the sociotechnical systems disciplines but provide important context. They are, therefore, included in CitationRef. 5.
Safety culture is specifically identified as a focal point for attention by the U.S. Nuclear Regulatory Commission [76 FR 34773 (CitationRef. 21)]; Institute of Electrical and Electronics Engineers [IEEE 1023-2020 (CitationRef. 9)]; U.S. Nuclear Regulatory Commission Regulation [NUREG-0711 (CitationRef. 8)]; and International Atomic Energy Agency [INSAG-15 (CitationRef. 22)]. However, the scientific validity of safety culture as a concept has recently been called into question.Citation23 At issue is the extent to which the factors included by the above references can be included within the integrated systems engineering framework specified in, for example, the Electric Power Research Institute (EPRI) “Digital Engineering Guide.”Citation6 Specifically, the challenge is to develop a set of top management core values upon which the modernization program is based and that can integrate safety culture concepts into the systems engineering framework.
It is a truism that the modernization of the nuclear industry will require major changes. Therefore, it would be prudent to pay some attention to the literature on organizational readiness for change.Citation24 A practical benefit is the identification of a methodCitation25 for accomplishing a change that combines user participation with an organizational goal alignment [Intervention Design and Analysis Scorecard (IDEAS)].
III. CONCEPTUAL FRAMEWORK
Based on the sociotechnical systems literature, we developed a strategic framework for the effective integration of human and organizational expertise within nuclear industry modernization efforts (see ).
Fig. 4. Strategic integration framework presented as a concept map. This figure was created using Cmap tools (cmap.ihmc.us).
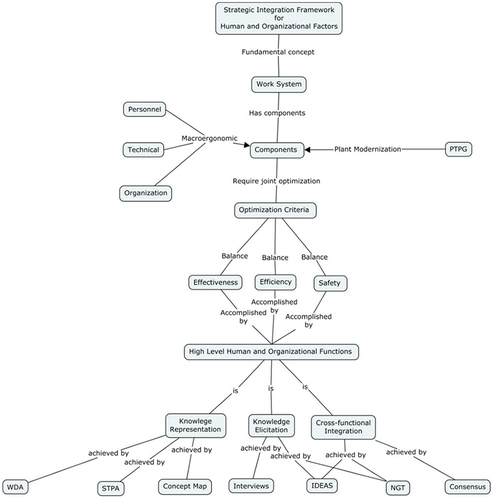
The basic unit of sociotechnical systems analysis is a work system. According to macroergonomic theory, a work system contains three components: personnel, technical, and organization and management. However, the four PTPG dimensions identified in the capability building framework of the Plant Modernization PathwayCitation4 can be mapped into the three components of the work system. An effective work system will jointly optimize these components. Joint optimization can be operationally defined, in the language of resilience engineering,Citation12 in terms of the interdependence among the three major systems performance criteria: effectiveness (accomplishment of mission), efficiency (optimization of resources), and safety (avoidance of injury, damage). As previously stated, excessive emphasis on any one criterion to the exclusion of others will, in the long run, likely lead to system failure. Case studies presented in CitationRef. 5 relating to the Boeing 737 MAX, Deepwater Horizon, and USS McCain disasters provide evidence of examples where an excessive emphasis on efficiency (particularly time pressures) over safety had catastrophic effects.
The practical work of actually bringing about joint optimization in the daily interactions within the work system can be described in terms of three problems: knowledge representation (how information about the work system is presented to those who need to operate on it), knowledge elicitation (how represented information is obtained from those who have the required expertise), and cross-functional integration (how information can flow freely between groups and help support collaboration and prevent silos).
Knowledge representation, knowledge elicitation, and cross-functional integration can be considered high-level human and organizational factors functions. These functions were applied to a set of practical problems described below. The intention was to elucidate how these functions could be used to enhance the practical work of stakeholders in the modernization design and operation in the same way that traditional HFE enhances the practical work of control room operators.
As shown in , the specific methodologies supporting the basic three functions above include Work Domain Analysis (WDA), STPA, Concept Mapping, Interviews, IDEAS, Nominal Group Technique (NGT), and Consensus. Note that itself is an example of a Concept Map application.
IV. EXAMPLES OF APPLICATIONS
The practical problems identified in CitationRef. 5 include top management consensus and the need for core values, HFE program management, obtaining tacit (undocumented) knowledge, new skills and capabilities of current employees, PTPG integration, and the silo problem. For each of these problems, proposed solutions involved a combination of at least two and usually three of the high-level human and organizational functions.
In the following sections, we will briefly describe two of these problems and associated solutions.
IV.A. Problem 1: HFE Program Management
The centrality of the cross-functional integration issue for HFE within the systems engineering process has been clearly stated. Section 4 of the EPRI “Digital Engineering Guide”Citation6 emphasizes that systems engineering is a collaborative enterprise in which no single perspective or discipline may be allowed to dominate. Accordingly, it is essential that HFE be a part of the system engineering process and, as such, be well integrated into the modification process from the beginning and not treated as a stand-alone process. In addition, HFE should be involved in gathering user input for design, for performing required design verification, and for providing a structured process for accomplishing these functions. The solutions discussed below are intended to enable that structured process.
IV.A.1. Solution 1 to Problem 1: Systematic Approach to Identification of Stakeholders Needs—The IDEAS Tool (Knowledge Elicitation, Cross-Functional Integration)
The EPRI “Digital Engineering Guide”Citation6 requires that as a starting point it is necessary to identify potential HFE impacts and stakeholder needs. If there are any HFE impacts, identify the stakeholders that may be affected by the change, and solicit participation from affected stakeholders.
The IDEAS tool, originally created for the development of health and safety interventions, represents a methodology for accomplishing these requirements.Citation25 As slightly modified for use in nuclear power plants (NPPs), the tool represents a framework for developing an overall end-point vision, concept of operations, and migration plan while considering HFE impacts and stakeholder needs.
The methodological framework assumes two levels of participation:
A design team, which identifies a focus area, evaluates needs and impacts, and based on needs assessment develops a set of alternative solutions.
A steering committee, to which the alternatives are submitted and which provides guidance and support throughout the process.
To summarize the process, the steering committee develops the overall general problem statement. The design team, through iterative and participative steps involving multiple stakeholders, defines the problem and conducts a systems analysis identifying needs, impacts, and contributing factors. From this input, a set of functional objectives is developed that addresses the needs and impacts developed in the previous step. Next, a set of selection criteria is developed to assess potential alternative solutions. Once the selection criteria are defined, the design team develops sets of proposed alternatives. The design team then assesses and/or combines proposed alternatives with respect to selection criteria. The outcome is a rating of each of the three alternatives on each of the selection criteria. This becomes the set of prioritized business cases for each of the alternatives. These are, in turn, submitted to the steering committee.
The steering committee reviews the alternatives, provides feedback to the design team, and makes a decision. This step includes the possibility of continued dialogue with the design team, resulting in possible modifications. The steering committee then implements the decision. Having done so, it then monitors and evaluates the impact of the decision. Modifications are made, if necessary, typically with further involvement of the design team.
The IDEAS tool provides a structured process by which the individual stakeholders can communicate their impacts and needs to the HFE team and to other stakeholders. When appropriate, Nominal Group Technique,Citation26 a structured method allowing each member of a group to have an equal opportunity to contribute, could be used at specific steps in the process. In addition, the tool can be easily adapted to the increased complexity of the NPP modification process. For example, identification of possible alternative solutions would likely need to be expanded to include input from vendors or other outside experts. Nevertheless, this tool provides a framework by which knowledge elicitation from stakeholders can be accomplished in a collaborative coordinated manner. At the same time, this information must be mapped into a form that affords efficient problem solving.
IV.A.2. Solution 2 to Problem 1: Representing the HFE Program Management Problem Space (Knowledge Representation)
This solution can be considered an extension of the previous solution in which the knowledge representation of the HFE program management problem space is characterized. Assume that the IDEAS tool discussed in Solution 1 has been utilized in this situation. The raw materials comprising the discussions and documentation utilized in Solution 1 would be represented in the upper left box of , “Actual Goals and Task Context of Domain.” The initial process of summarizing and integrating this material, typically by different stakeholders, would be represented by the lower left box of , “CSE Approximation of Domain.” As alternative automation possibilities become conceptualized, more formal representations, equivalent to “(Display)” in , are created. Effectively, the aim of these representations is to enable formation of mental models of proposed alternatives by participants. This would be the expectation initially for members of the design group and, ultimately, for the members of the steering committee.
Fig. 5. The mapping principle. (Adapted from CitationRef. 28, by Elm; originally in CitationRef. 29, by Woods).
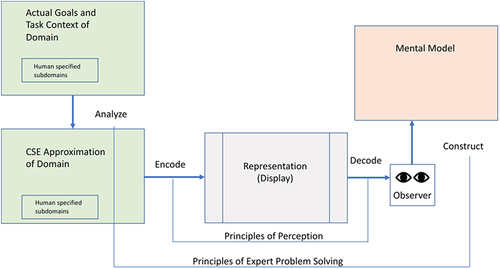
In the original report,Citation5 Work Domain AnalysisCitation27 was used as an example of an effective formal knowledge representation for this problem. However, Work Domain Analysis, as a component of Cognitive Work Analysis, has been widely used in the nuclear industry and elsewhere, and it is, therefore, not necessary to describe it further in this paper.
IV.B. Problem 2: Obtain Tacit Knowledge from Experts (Knowledge Elicitation)
The issue of tacit (undocumented) knowledge of the personnel within any complex sociotechnical work system presents many problems. The very complexity of the systems implies that it is difficult if not impossible to document completely the details of every procedure. Should any of these personnel leave without their knowledge being documented, critical gaps in organizational performance are likely to occur. In the Deep Water Horizon disaster, for example, only one senior employee had accurate (tacit) knowledge of how a critical test should be interpreted, and he was away on training the day of the explosion.Citation30 Understanding the tacit components of current processes would be particularly important in NPP modernization.
IV.B.1. Solution 1 to Problem 2: Using the EPRI Knowledge Elicitation Methods (Knowledge Elicitation)
EPRI performed a project to capture undocumented (tacit) task knowledge.Citation31 The process began by asking several levels of management at a facility which worker had the most valuable tacit knowledge. Next, a similar question would be asked of peers in an organization, and finally, when an individual had been identified, he or she was asked what tacit knowledge they held that was believed by them as most valuable. For these knowledge elicitation exercises, a list of questions was developed for each expert. The knowledge elicitation team discussed the questions with the expert and followed up on answers that provided valuable information.
Interestingly, several times the experts said that they did not believe they had valuable tacit knowledge but came to realize after the knowledge elicitation process that they did. For example, at an old fossil plant, two tacit knowledge experts were identified. One knew where buried pipes were located on the plant site, and no map of such pipe locations could be found. The second expert knew where all of the equipment drawings were located because they were more or less randomly stored. This was a very old plant, and when equipment needed to be replaced, the plant would need to provide the drawings for the part to be custom-built by an outside vendor because replacement parts or the entire unit were no longer available. Similarly, at a fuel fabrication plant, the identified expert was the only one who could quickly calculate future radiation levels. Others were able to perform calculations that would provide similar results, but the difference was that he could provide the results in 2 h and others might require at least a day.
IV.B.2. Solution 2 to Problem 2: Using the EPRI Knowledge Elicitation Methods (Knowledge Representation)
Typically, the interviewer would meet for 2 days with each expert. Between days, the interviewer would prepare a document that outlined the expertise provided. This was modified by the expert, and follow-up questions were discussed. Finally, the team would finalize an outline of the tacit knowledge that was elicited. This constituted the knowledge representation component of the solution and typically included the use of concept maps.
IV.C. Comment on Problems and Solutions
The tools and methods presented in this report are not meant to be used rigidly; they are a means to practical solutions. In many circumstances, a “mix and match” among parts of different tools and methods may be appropriate given the circumstances. In any case, the application of any of the approaches described herein should be subject to the following usability criteria.Citation32 Usability is defined as a combination of usefulness and feasibility. Usefulness, in turn, is a combination of impact (the effect on the outcome) and uniqueness (compared with alternative available methods). Feasibility relates to available time and resources. It can be argued that the above solutions are both useful and feasible.
The interaction of the PTPG components in the work system must be attended to in the application of this approach. In each of the above examples, it is possible to parse out the relative contributions of people, technology, process, and governance to the overall function of the system. In the case of Problem 2, “Tacit Knowledge,” an individual person was the only one who had knowledge of where equipment drawings were located (technology). According to the current governance, if equipment needed to be repaired, the process was to contact this individual. However, this revealed an in-balance in the relationship between the criteria: effectiveness, efficiency, and safety. The current system was efficient (quite cost-effective) but only if the key employee was available. If he or she was absent, or had left the company, the outcome would likely result in serious consequences for effectiveness (accomplishment of mission) and possibly safety. As mentioned above, this is exactly what happened at Deep Water Horizon.Citation30
However, there were particular challenges in the solution to the Tacit Knowledge Problem. Some expert respondents did not want to provide their expertise because they wanted to come back as consultants following retirement. Others were afraid of being forced to retire after their knowledge was elicited; they were viewed as the expert on a topic by peers and management, and they did not want to lose that status, or they were angry at the organization because they did not receive a promotion or the raise they thought they deserved.
Nevertheless, the organizational consequences of this kind of tacit knowledge being lost are so great that this effort is well worth the cost. This is particularly the case for plant modernization.
IV.D. Extended Application
An extended application of the sociotechnical approach described above is presented by Kovesdi et al.Citation33 These were conducted in partnership with a utility concerned about modernization. This approach was applied to the analysis and design of two separate modernization use cases within the utility partner’s operations.
The first, the Radiation Protection (RP) use case, focused on identifying potential design approaches for improving efficiency and performance of maintenance-related RP tasks without loss of safety. The second, the Information Support use case, focused on analysis and design of methods and tools to enhance efficiencies in managerial decision making and issue resolution, specifically regarding support of forcing function meetings such as management review meetings. While each case used different analytic and design tools to accomplish its objectives, they both shared a fundamental emphasis on the core sociotechnical principles presented above.
V. SUMMARY, CONCLUSIONS, AND RECOMMENDATIONS
V.A. Summary
It is assumed that the modernization of NPPs will require an advanced concept of operations involving an integrated set of tightly coupled systems in which all stakeholders act in a coordinated manner. For this modernization effort to be enabled, we developed a human and organizational factors approach based on a broad sociotechnical framework. Starting from core human factors principles, we conducted a literature review of the methods and approaches relevant to the modernization problem. These included core disciplines such as CSE, STAMP, human systems integration, resilience engineering, and macroergonomics but also related topics of safety culture and organizational change. From this literature, we developed a conceptual framework centered around the work system with its four interacting components: people, technology, process, and governance. In an effective work system, these four components are jointly optimized according to three systems criteria: efficiency, effectiveness, and safety. System failure may result from excessive emphasis on any one criterion. The actual work of attaining joint optimization in a given work system can be accomplished by utilizing three high-level functions: knowledge elicitation, knowledge representation, and cross-functional integration. We illustrated the utility of this approach by applying it to practical problems and case studies.
V.B. Conclusions
Utilities are and will be modernizing their NPPs. Many are pursuing a digital transformation instead of doing like-for-like replacements. A digital transformation process includes developing an advanced concept of operations applicable to plant design and operations, and the process needs to involve technology considerations, systems engineering, and human and organizational expertise.
In particular, the set of capabilities required for integrated operations, as seen in , necessarily involves the combined operation of four components: (1) people, (2) technology, (3) process, and (4) governance. We have developed a human and organizational factors approach based on sociotechnical systems theory (see ) in which these four components are conceptualized as the elements of a work system; such elements are jointly optimized with respect to maintaining a balance among effectiveness, efficiency, and safety. Operationally, joint optimization can be accomplished by applying three high-level human and organizational functions—knowledge acquisition, knowledge representation, and cross-functional integration—and their associated tools. (See .) Examples of the successful application of this approach have been presented. (See above and Ref. 33 by Kovesdi et al.)
V.C. Recommendations
It is recommended that the human and organizational strategic framework described above be considered in developing a HFE plan at NPPs that are planning a technology-centric modernization effort. This application could serve as a test case for the human and organizational strategic framework and will provide results that can be used to update and validate the framework.
It is further recommended that the human and organizational strategic framework be integrated into the Plant Modernization Pathway from the U.S. Department of Energy LWRS Program Strategic Action Plan and Technology Centric Plant Operations Model.
Acknowledgments
This manuscript has been authored by Battelle Energy Alliance, LLC under contract number DE-AC07-05ID14517 with the U.S. Department of Energy. The Idaho National Laboratory–issued document number for this paper is INL/JOU-22-57908.
Disclosure Statement
No potential conflict of interest was reported by the author(s).
References
- K. D. THOMAS and P. J. HUNTON, “Light Water Reactor Sustainability Program Nuclear Power Plant Modernization Strategy and Action Plan,” INL/EXT-19-55852, Idaho National Laboratory (2019).
- P. J. HUNTON and R. T. ENGLAND, “Vendor-Independent Design Requirements for a Boiling Water Reactor Safety System Upgrade,” INL/EXT-20-61079, Idaho National Laboratory (2020).
- J. C. JOE, T. MIYAKE, and A. HALL, “Guidance on Transforming Existing Light Water Reactors into Fully Modernized Nuclear Power Plants: The Role of Plant Modernization R&D,” INL/LTD-21-64369, Idaho National Laboratory (2021).
- K. THOMAS et al., “Analysis and Planning Framework for Nuclear Plant Transformation,” INL/EXT-20-59537, Idaho National Laboratory (2019).
- M. J. DAINOFF et al., “Addressing Human and Organizational Factors in Nuclear Industry Modernization: An Operationally Focused Approach to Process and Methodology,” INL/EXT-20-57908, Idaho National Laboratory (2020).
- “Digital Engineering Guide: Decision Making Using Systems Engineering,” Technical Report 3002011816, Electric Power Research Institute (2021).
- “Human Factors Guidance for Control Room and Digital Human-System Interface Design and Modification,” Technical Report 3002004310, Electric Power Research Institute (2015).
- “Human Factors Engineering Program Review Model,” NUREG-0711, Rev. 3, U.S. Nuclear Regulatory Commission (2012).
- “IEEE Recommended Practice for the Application of Human Factors Engineering to Systems, Equipment, and Facilities of Nuclear Power Generating Stations and Other Nuclear Facilities,” IEEE 1023-2020, Institute of Electrical and Electronics Engineers (2020).
- G. ROPOHL, “Philosophy of Socio-Technical Systems,” Soc. Philos. Technol. Q. Electron. J., 4, 3, 186 (1999); https://doi.org/10.5840/techne19994311.
- E. HOLLNAGEL, “Resilience—The Challenge of the Unstable,” Resilience Engineering: Concepts and Precepts, E. HOLLNAGEL, D. WOODS, and N. LEVESON, Eds., Ashgate Publishing Ltd., Farmham, United Kingdom (2006).
- E. HOLLNAGEL and D. D. WOODS, Joint Cognitive Systems: Foundations of Cognitive Systems Engineering, CRC Press, Boca Raton, Florida (2005).
- M. J. DAINOFF, “Can’t We All Just Get Along? Some Alternative Views of the Knowledge Worker in Complex HCI Systems,” Int. J. Hum.–Comput. Inter., 25, 5, 328 (2009); https://doi.org/10.1080/10447310902864944.
- B. M. KLEINER et al., “Sociotechnical Attributes of Safe and Unsafe Work Systems,” Ergonomics, 58, 4, 635 (2015); https://doi.org/10.1080/00140139.2015.1009175.
- J. RASMUSSEN, A. M. PEJTERSEN, and L. P. GOODSTEIN, Cognitive Systems Engineering, Wiley, New York (1994).
- N. LEVESON, Engineering a Safer World, MIT Press, Cambridge, Massachusetts (2011).
- B. CRANDALL, G. KLEIN, and R. HOFFMAN, Working Minds: A Practitioner’s Guide to Cognitive Task Analysis, MIT Press, Cambridge, Massachusetts (2006).
- J. M. SCHRAAGEN et al., Naturalistic Decision Making and Macrocognition, Ashgate, Burlington, Vermont (2008).
- G. KLEIN et al., Decision Making in Action: Models and Methods, Ablex Publishing, Norwood, New Jersey (1993).
- H. BOOHER, Handbook of Human Systems Integration, John Wiley & Sons, New York (2003).
- “Final Safety Culture Policy Statement – 76 FR 34773,” U.S. Nuclear Regulatory Commission (2011).
- “Key Practical Issues in Strengthening Safety Culture,” INSAG-15, International Atomic Energy Agency, International Nuclear Safety Advisory Group (2002).
- A. P. GONCALVES FILHO and P. WATERSON, “Maturity Models and Safety Culture: A Critical Review,” Saf. Sci., 105, 192 (2018); https://doi.org/10.1016/j.ssci.2018.02.017.
- M. M. ROBERTSON and D. TUBBS, “Organizational Readiness for Change: A Systematic Literature Review and Field Experience as Related to Safety and Wellness Improvements at Work,” presented at European Academy of Management 2016 Conference (EURAM 2016), Paris, France, June 1–4, 2016.
- M. ROBERTSON et al., “The Intervention Design and Analysis Scorecard: A Planning Tool for Participatory Design of Integrated Health and Safety Interventions in the Workplace,” J. Occup. Environ. Med., 55, 12, 86 (2013); https://doi.org/10.1097/JOM.0000000000000036.
- A. L. DELBECQ, A. H. VAN DE VEN, and D. H. GUSTAFSON, Group Techniques for Program Planning: A Guide to Nominal Group and Delphi Processes, Green Briar Press, Middleton, Wisconsin (1986).
- K. J. VICENTE, Cognitive Work Analysis: Toward Safe, Productive, and Healthy Computer-Based Work, Erlbaum, Mahwah, New Jersey (1999).
- W. ELM et al., “Pragmatic Use of Cognitive Work Analysis in System Design Extending Current Thinking by Adapting the Mapping Principle,” Applications of Cognitive Work Analysis, A. M. BISANTZ and C. M. BURNS, Eds., CRC Press, Boca Raton, Florida (2008).
- D. D. WOODS, “The Cognitive Engineering of Problem Representation,” Human-Computer Interaction and Complex Systems, G. WEIR and J. ALTY, Eds., Academic Press, London, United Kingdom (1991).
- E. BOEBERT and J. M. BLOSSOM, Deepwater Horizon, A Systems Analysis of the Macondo Disaster, Harvard University Press, Cambridge, Massachusetts (2016).
- “Real Time Expert Knowledge Acquisition and Transfer: Needs and Technology Assessment,” Technical Report 1009581, Electric Power Research Institute (2004).
- N. NAIKAR, “Beyond the Design of Ecological Interfaces: Applications of Work Domain Analysis and Control Task Analysis to the Evaluation of Design Proposals, Team Design, and Training,” Applications of Cognitive Work Analysis, A. M. BISANTZ and C. M. BURNS, Eds., CRC Press, Boca Raton, Florida (2008).
- C. KOVESDI et al., “Nuclear Work Function Innovation Tool Set Development for Performance Improvement and Human Systems Integration,” INL/EXT-21-64428, Idaho National Laboratory (2021).