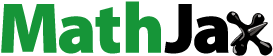
Abstract
Uranium has been identified as an element of interest for the safety assessment of a deep geological repository for used nuclear fuel. This paper examines the sorption behavior of U(VI) onto MX-80 bentonite and granite in Ca-Na-Cl solutions of varying ionic strengths [0.05 to 3 mol/kgw (m)] and across a pH range of 4 to 10. U(VI) sorption on MX-80 showed that U(VI) sorption gradually increased with pHm until pHm = 6, where it reached its maximum, and decreased slightly with pHm until pHm = 8, and then became constant. U(VI) sorption on granite increased along with pHm, reached the maximum around pHm = 7 to 8, and then slightly decreased with pHm. Both MX-80 and granite showed essentially no ionic strength dependence for sorption of U(VI). A nonelectrostatic surface complexation model successfully predicted sorption of U(VI) onto MX-80 and granite using the formation of an inner-sphere surface complex. Optimized values of surface complexation reaction constants (log K0) for the formation reactions of these surface species are proposed.
I. INTRODUCTION
Sorption of radionuclides onto engineered and natural barrier materials can be an important mechanism for slowing the transport of radionuclides to the surface environment (e.g., Refs. [Citation1–3]). Understanding sorption rates, therefore, is relevant for the performance of systems, such as deep geological repositories for used nuclear fuel waste. This paper explores the sorption of uranium under potential deep repository conditions in a granitic rock environment. Specifically, this paper considers conditions that may be observed in a Canadian deep geologic repository in the Canadian Shield.[Citation4] Bentonite clay will be used in the engineered barrier system for a deep geological repository; therefore, the study of sorption of radionuclides on bentonite is also of interest.
Uranium is a major radionuclide in used nuclear fuel waste and a parent element of many radioactive daughter elements. Used nuclear fuel wastes will be encapsulated in copper-coated steel used fuel containers and isolated and contained in a deep geological repository. Following repository closure, groundwater will be expected to eventually move through the repository engineered barriers (buffer and backfill). If the container fails, groundwater can enter and uranium (in UO2 matrix) will be dissolved into the groundwater. Under the oxidizing conditions, U may be in the oxidized form [U(VI)], which can be more mobile than the reduced form [U(IV)]. Hence, we focused on the sorption of U(VI) species in this study.
In this paper, sorption of U(VI) onto MX-80 bentonite and granite was studied in Ca-Na-Cl solutions with ionic strengths (I) of 0.05 to 3 mol/kgw (m), as well as in the reference groundwater, CR-10, representing the potential groundwater geochemical conditions at the repository depth of Canadian crystalline rock.[Citation5] CR-10 is Ca-Na-Cl–type water with a total dissolved solids concentration of 11.3 g/L or an ionic strength of 0.24 m. The Na/Ca mole ratio (1.6) of the Ca-Na-Cl solutions is the same as that of the CR-10 reference water. MX-80 is a bentonite clay from the Clay Spur bed in the U.S. state of Wyoming, consisting of about 80 wt% Na-rich montmorillonite. The granite rock sample was collected from Manitoba, Canada.
There have been many previous studies on the sorption of U(VI) on bentonite/montmorillonite and granite at low I (I ≤ 0.1 mol/L) (e.g., Refs. [Citation6–21]). However, only a few studies have been conducted on sorption of U(VI) on bentonite and granite at high ionic strengths. Stockmann et al.[Citation22] studied the sorption of U(VI) on SWy-2 Na-montmorillonite in NaCl or CaCl2 solutions at ionic strengths (I) = 0.3, 1.0, and 3.0 m in the absence of CO2 and 0.1, 0.3, 1.0, 2.0, and 3.0 m in the presence of CO2 by batch and spectroscopic experiments. They also applied the two-site protolysis nonelectrostatic surface complexation and cation exchange sorption model (2SPNE SC/CE), reported the surface complexation reactions and the values of the surface complexation reaction constants (log K0), and justified their sorption modeling by using literature data at I = 0.1 mol/L. As far as the authors know, Huitti et al.,[Citation23] Schmeide et al.,[Citation24] and Berry et al.[Citation25] investigated the sorption of U(VI) at I > 0.1 mol/L on rapakivi granite, Äspö diorite, and granodiorite, respectively. However, they did not study the pH and I dependence of U(VI) sorption in the wide range of pH and I as Stockmann et al. did.[Citation22]
In this study, sorption of U(VI) on MX-80 bentonite and granite was systematically investigated in Ca-Na-Cl solutions with ionic strengths of 0.05 to 3 m at pHm = 4 to 10 by batch experiments and the 2SPNE SC/CE model. We discuss whether the surface complexation reactions and the values of log K0 reported in the previous papers are applicable or not to the sorption of U(VI) onto MX-80. The surface complexation reactions and values of log K0 are proposed for U(VI) sorption onto granite.
II. EXPERIMENTAL
II.A. Materials
All chemicals used in this work were reagent grade and purchased from Fisher Scientific. The deionized water used was prepared with a Milli-Q Direct 8 (18.2 MΩ/cm) system. The MX-80 bentonite sample was provided by the American Colloid Company and was used as received. The specific surface area of MX-80 was 26.2 m2/g.[Citation26] Other information, including the mineralogy of this material, is described elsewhere.[Citation26] The granite sample was supplied by the Nuclear Waste Management Organization (NWMO) and originated from the Lac du Bonnet batholith in Manitoba, Canada. Batholiths are common in the Canadian Shield. The main mineralogical composition of the Lac du Bonnet batholith granite being used in this work is plagioclase feldspar (37%), quartz (34%), alkali-feldspar (19%), biotite (3%), myrmekite (2%), chlorite (1.4%), muscovite (1%), opaques (0.9%), hornblende (0.9%), epidote (0.2%), and sphene (0.2%) (provided by the NWMO). The specific surface area of the granite was 0.226 m2/g. Other information on the Lac du Bonnet batholith granite is described elsewhere.[Citation27] The granite sample was crushed using a tungsten carbide cylinder supplied by Nichika. Using a range of stainless steel sieves, granite particles with sizes between 150 to 300 μm were collected and used in all subsequent sorption experiments.
A glove box (GB) was used by filling it with N2 gas (>99.999%) to exclude CO2. All the sorption experiments were conducted in the GB.
A natural U(VI) stock solution (5 × 10−4 mol/L) in 0.01 mol/L HCl was provided by the Department of Health Physics, McMaster University. The Ca-Na-Cl solutions (Na/Ca mole ratio = 1.6) with I = 0.05, 0.1, 0.24, 1, and 3 m were prepared using pure NaCl and CaCl2•2H2O compounds. The CR-10 reference groundwater (Na/Ca mole ratio = 1.6, I = 0.24 m) was prepared using the masses of reagents shown in . All reagents were dissolved into 1 kg of deionized water in total.
TABLE I Masses of Chemical Compounds Added to 1 kg of Deionized Water for Preparation of the CR-10 Reference Groundwater
To perform pH measurements, a Fisherbrand Accumet AB200 Benchtop pH meter was used in conjunction with a Fisherbrand Accumet pH combination electrode. The calibration of the pH meter was completed using pH 4.0-, 7.0-, and 10.0-pH buffer solutions. The pH values measured by the pH meter pHmeasure were operational values; therefore, they needed to be converted. The conversion of pHmeasure to the molal H+ concentration (pHm = −log mH+) has been described elsewhere.[Citation28,Citation29] In this study, the pHm in solutions was determined by acid-base titration (Metrohm Ti-Touch 916).
The redox potentials of the solutions at sorption kinetics tests were measured using a Pt combined electrode with Ag/AgCl reference electrode (Fisher Scientific Accumet AB 150/Accumet ORP electrode) and converted into Eh versus SHE (standard hydrogen electrode). It was found that the Eh values were around 300 to 350 mV versus SHE. Therefore, we considered that the reducing conditions were not generated under the pure N2 atmosphere.
II.B. Sorption Experiment
II.B.1. Sorption Kinetics
All experiments were conducted at 25°C in triplicate in the GB. The experimental procedures were the same as those for our experiments on Np(IV) sorption in Na-Ca-Cl solutions.[Citation30] In the present work, the sorption distribution coefficient Rd (mL/g) was used to express the results of the sorption experiment:
where
C0 = | = | initial concentration of U(VI) in solution (1 × 10−6 m) |
Ce = | = | U(VI) concentration in solution at sorption equilibrium (m) |
V = | = | volume of the solution (mL) |
W = | = | mass of the MX-80 or granite (g). |
The solid/liquid ratio for all sorption experiments was 0.3 g/30 mL. All sorption experiments were preceded by pre-equilibration, where 30 mL of the Ca-Na-Cl solution with respective ionic strength (0.05, 0.1, 0.24, 1, or 3 m) was mixed with 0.3 g MX-80 or granite. The suspension was kept in the GB for a week, before the solution was separated by centrifugation (6 min at 3000 rpm), and then removed with a pipette. Then, 30 mL of the same Ca-Na-Cl solution (with the same ionic strength) was added to the tube and spiked with U(VI) stock solution to reach an initial concentration of 1 × 10−6 m. The solubility of UO2(OH)2 in the 0.1-m Ca-Na-Cl solution at pH = 7 was estimated as 5 × 10−6 m by PHREEQC[Citation31] [with the Japan Atomic Energy Agency (JAEA) thermodynamic database[Citation32]] calculation. Moreover, Stockmann et al.[Citation22] also used 1 × 10−6 m as their initial concentration of U(VI) for U(VI) sorption experiments. Thus, 1 × 10−6 m was considered smaller than the solubility limit of U(VI) under oxidizing conditions throughout this work.
The sorption kinetics of U(VI) on MX-80 and granite in Ca-Na-Cl solutions were conducted for I = 0.05 m and 3 m. After the U(VI) stock solution was spiked, the value of pHm was adjusted to 6 ± 0.3. During the experiment, the sample tubes containing the U(VI), solid and solution were gently shaken and kept at 25°C. The value of pHm was measured daily in the GB, and when the value shifted more than ±0.3 from the initial value, the pHm was adjusted by adding a small amount of HCl or NaOH solution. After the predecided period of time, the solution was separated from the solid by ultracentrifugation using an Optima™ Max-XP Biosafe Ultracentrifuge System, at 100 000 rpm for 15 min at 25°C. The pHm of the solution was measured in the GB, and an aliquot was taken and transferred to a test tube. The U(VI) concentration in the test tube was measured by inductively coupled plasma mass spectroscopy (ICP-MS) (Agilent ICP-MS 8800). The detection limit of U was determined to be smaller than 5 × 10−12 m.
II.B.2. pHm and Ionic Strength Dependence of Rd
The pHm and I dependences of the Rd of U(VI) sorption on MX-80 and granite in Ca-Na-Cl solutions were measured at pHm = 4 to 10, and I = 0.05, 0.1, 0.24, 1, and 3 m. The pHm of the solution was measured once a day in the GB. When the value of the pHm changed by more than ±0.3 from the initial one, the pHm was re-adjusted by the addition of a small amount of HCl or NaOH solution. Due to the small volume of the added HCl or NaOH solution, the effect of the HCl or NaOH addition on the final concentration of U(VI) and Rd calculation was considered to be negligible. As shown in Sec. III, the sorption equilibrium under the experimental conditions in this study was reached within 7 days. Therefore, the sorption period was established as 14 days for the dependence of pHm and I on Rd sorption experiments.
Furthermore, Rd values for U(VI) sorption onto MX-80 and granite in the CR-10 reference groundwater were also measured and calculated. The pHm was adjusted to 7 ± 0.5 using a small amount of HCl or NaOH solution when the value of the pHm changed by more than ±0.3 from the initial value. All procedures were the same as those for the sorption kinetics measurements.
III. RESULTS AND DISCUSSION
III.A. Sorption Kinetics
The sorption kinetics of U(VI) on MX-80 and granite in Ca-Na-Cl solutions at I = 0.05 m and 3 m are illustrated in . It was found that the sorption of U(VI) reached equilibrium within 7 days on both solids at both ionic strengths. Based on these results, the sorption reaction period was set for 14 days for experiments of the dependence of U(VI) sorption on the pHm and I for both MX-80 and granite.
III.B. Sorption on MX-80
The Rd values of U(VI) sorption onto MX-80 in Ca-Na-Cl solutions with different ionic strengths are plotted against the pHm in . A gradual increase of Rd is observed along with increasing pHm until pHm = 6, where the maximum Rd value is observed. Then the Rd values decrease slightly as the pHm increases, until pHm = 8. The Rd values become constant after a pHm of 8. Interestingly, the Rd values are similar for different I within the range of 0.05 and 3 m.
Fig. 2. pHm and ionic strength dependence of the Rd values of U(VI) sorption on MX-80 in Ca-Na-Cl solutions.

Stockmann et al.[Citation22] found a complete U(VI) sorption on SWy-2 Na-montmorillonite in the CO2-free NaCl solution above pHc 6. They also observed that U(VI) sorption on SWy-2 Na-montmorillonite in the CO2-free CaCl2 solution increased above pHc 4, with maximum values above pHc 6, where the latter were slightly lower than in the respective NaCl solution. They concluded that this slight reduction of sorption in the CaCl2 solution was due to a competition of Ca2+ and UO22+ for sorption sites.
The pHm dependence of the Rd values obtained in this study seems to be consistent with the observations of Stockmann et al.[Citation22] On the other hand, Stockmann et al.[Citation22] observed a very slight increase in U(VI) sorption with I both in the absence and presence of CO2 from the Attenuated Total Reflection Fourier-Transform Infrared Spectroscopy (ATR FT-IR) in situ sorption experiment. However, in their batch sorption experiment results, U(VI) sorption practically did not show dependence on I.
The Rd value of U(VI) on MX-80 in the CR-10 solution was estimated as 1.1 × 103 ± 8.0 × 101 ml/g (at pHm = 7.2). This is within the range of the Rd values measured in the Ca-Na-Cl solutions around pHm = 7, suggesting that the impact of minor ions, such as Mg2+ and Sr2+, on the sorption of U(VI) would be small.
The 2SPNE SC/CE model was developed based on a large number of experimental data on illite and montmorillonite. It has been successfully applied to study the sorption of numerous solids with oxidation states ranging from (+I) to (+VI) quantitatively.[Citation33–36] We also have applied the 2SPNE SC/CE model to the sorption of Np(V), Np(IV), Se(-II), and Eu(III) on montmorillonite, illite, and granite, and discussed their sorption reactions.[Citation30,Citation37–40]
In this study, we applied the 2SPNE SC/CE model incorporated in PHREEQC[Citation31] to all experimental data on the pHm and I dependences of the Rd values of U(VI) sorption on MX-80 shown in . This was done to determine the surface complexation reactions and the optimized value of reaction constant log K0 for each surface complexation reaction. Since the ionic strengths of the Ca-Na-Cl solutions being used in this study are ≤3 m, we adopted the specific ion interaction theory to estimate the activity coefficients of species in solutions.[Citation41] The used thermodynamic data of U(VI) were taken from the JAEA thermodynamic database.[Citation32] To initiate the fitting of the model, the values of log K0 reported by Stockmann et al.[Citation22] were used as the initial values of the constants. The values of other parameters, such as protolysis constants, were the same as those used by Walker et al.[Citation42]
In the study with the latest version of the 2SPNE SC/CE model by Stockmann et al.,[Citation22] only strong sites of montmorillonite (≡SSOH) were used for modeling cation surface complexation data at trace metal ion concentrations. Their calculations successfully predicted the experimental data,[Citation22] so we also considered only strong sites in this study. Furthermore, the contribution of the cation exchange reaction was small at pHc ≥ 4 in the modeling by Stockmann et al.,[Citation22] so we did not consider the cation exchange reaction.
The fitting results at I = 0.24 m are illustrated in . Other fitting results at I = 0.05, 0.1, 1, and 3 m are shown in the supplementary materials (Figs. S1 through S4). The optimized values of log K0 for MX-80 obtained in this study, and those for montmorillonite/bentonite in other studies,[Citation22,Citation34,Citation43] are summarized in .
TABLE II Comparison Between Surface Complexation Constants Log K0 of U(VI) on Montmorillonite Obtained in This Work and Literature Values[Citation22,Citation35,Citation36]
Fig. 3. Fitting results for U(VI) sorption on MX-80 at ionic strength 0.24 m in Ca-Na-Cl solution with the 2SPNE SC/CE model. The red, blue, and green lines represent ≡SOH + UO22+ ⇄ ≡SOUO2+ + H+, ≡SOH + UO22+ + H2O ⇄ ≡SOUO2OH + 2H+, and ≡SOH + UO22+ + 3H2O ⇄ ≡SOUO2(OH)32− + 4 H+, respectively. The black line represents the total of the modeled sorption.

The overall results suggested that the pHm and I dependences of U(VI) sorption on montmorillonite predicted by the 2SPNE SC/CE model were consistent with the experimental data obtained in this study. The value of log K0 (4.2 ± 0.2) for the surface complexation reaction ≡SOH + UO22+ ⇄ ≡SOUO2+ + H+ optimized in this study was larger than that reported by Stockmann et al.,[Citation22] Marques Fernandes et al.,[Citation43] and Bradbury and Baeyens.[Citation34] This was out of the range of the log K0 value of the linear free-energy relationship (3.0 ≤ log K0 ≤ 3.8). The modeling results seemed to slightly underestimate the value of Rd at pHm = 4 to 5. The Rd value at pHm = 4 to 5, which may be underestimated, and the validation of the log K0 value will be further studied.
The optimized values of log K0 for the surface complexation reactions ≡SOH + UO22+ + H2O ⇄ ≡SOUO2OH + 2H+ and ≡SOH + UO22+ + 3H2O ⇄ ≡SOUO2(OH)32− + 4 H+ were similar to those reported in previous studies.[Citation22,Citation34,Citation43] Furthermore, it was found that the surface complexation model could reproduce the experimental data efficiently with the model without considering the surface complexation reaction ≡SOH + UO22+ + 2H2O ⇄ ≡SOUO2(OH)2− + 3H+, which agreed with the conclusion of Stockmann et al.[Citation22]
These results suggest that the sorption of U(VI) on MX-80 in Ca-Na-Cl solution at a pH between 4 and 10, and an I between 0.05 and 3 m, could be simulated well by three inner-sphere surface complexation reactions, and that the optimized value of log K0 for the surface complexation reaction ≡SSOH + UO22+ ⇄ ≡SSOUO2+ + H+ on MX-80 was larger than that on SWy-2 Na-montmorillonite.[Citation22] However, the values of the other two reactions on MX-80 were consistent with those on SWy-2 Na-montmorillonite.[Citation22]
III.C. Sorption on Granite
The dependence of the Rd values on pHm and I is illustrated in . These results showed that the Rd values increase with pHm, reaching a maximum around pHm 7 to 8, and then slightly decreasing as the pHm increases. This trend is qualitatively similar to that observed in the previous studies with granite,[Citation9,Citation11,Citation15] orthoclase and muscovite,[Citation10,Citation13] and K-feldspar.[Citation12] On the other hand, the Rd values in the alkaline region in previous studies decreased more steeply than the Rd values in , although those were studied in the absence of CO2. Negligible dependence of Rd on I in this study has agreed with previous research.[Citation11,Citation15] The values of Rd obtained in this study might be slightly larger than those in previous works.[Citation9–13,Citation15] The reason, including a possibility of U(VI) reduction to U(IV), will be investigated in the future.
Fig. 4. pHm and ionic strength dependences of the Rd values of U(VI) sorption on granite in Ca-Na-Cl solutions.

The Rd value of U(VI) on granite in the CR-10 solution was estimated as 1.0 × 103 ± 1.1 × 102 ml/g (at pHm = 6.9). This is within the range of Rd values measured in Ca-Na-Cl solutions around pHm = 7. These results suggest that the impact of minor ions, such as Mg2+ and Sr2+, on the sorption of U(VI) onto granite would be small.
The 2SPNE SC/CE model was also simultaneously applied to all experimental data on the pHm and I dependences of the Rd values of U(VI) on granite. Granite is composed of feldspar [plagioclase feldspar (37%) and K-feldspar (19%)], quartz (34%), biotite (3%), myrmekite (2%), chlorite (1.4%), muscovite (1%), opaques (0.9%), hornblende (0.9%), epidote (0.2%), and sphene (0.2%). In the modeling of granite, we assumed that three main minerals (feldspar, quartz, and biotite) contribute to the sorption of U(VI). Feldspar is divided into two main groups: plagioclase feldspar [NaAlSi3O8-CaAl2Si2O8 (albite and anorthite)] and alkalic-feldspar [K/NaAlSi3O8 (albite and all K-feldspars)].[Citation44,Citation45] We assumed that the surface properties of plagioclase feldspar and K-feldspar are the same.
The protolysis reaction constants of these mineral components of granite from the Lac du Bonnet batholith in Manitoba, Canada, have not been studied yet. Hence, in this study we assumed that the protolysis reaction constants and the site densities of feldspar, quartz, and biotite were the same as those of Inada granite (albite, quartz, and biotite) used by Iida et al.[Citation46] also considered different sorption sites for each of the main mineral components (albite, quartz, and biotite), although most of the previous studies (e.g., Refs. [Citation11,Citation15]) only considered one sorption site for granite and could successfully fit the sorption modeling to the sorption data of Th onto granite. summarizes the protolysis reaction constants of the three main mineral components of granite being considered in the modeling.[Citation46] shows the site density of the three main mineral components of granite.[Citation46]
TABLE III Protolysis Reaction Constants of Feldspar, Quartz, and Biotite Used in This Study
TABLE IV Site Density of Feldspar, Quartz, Biotite, and Chlorite Used in This Study
To apply the 2SPNE SC/CE model to the sorption of U(VI) onto the granite mineral components (feldspar, quartz, and biotite), we assumed that U(VI) occupies the biotite site first. This was because previous studies reported that biotite shows strong sorption of U(VI).[Citation15,Citation47–49] Those studies showed not only the macroscopic data, but also the sorption mechanism with spectroscopic techniques and density functional theory (DFT) calculations. Therefore, in this study we considered that after all the sorption sites of biotite were occupied by U(VI), the sorption of U(VI) onto other mineral components would then start. Consequently, we found that the sorption of U(VI) only on biotite could fit the experimental data.
The fitting results at I = 0.24 m are shown in . Other fitting results for I = 0.05, 0.1, 1, and 3 m are shown in the supplementary materials (Figs. S5 through S8). The optimized values of log K0 for granite/biotite obtained in this study are summarized in .
TABLE V Surface Complexation Constants Log K0 of U(VI) Sorption on Granite/Biotite Obtained in This Work
Fig. 5. Fitting results for sorption of U(VI) onto granite/biotite at ionic strength of 0.24 m in Ca-Na-Cl solution with the 2SPNE SC/CE model. The red, blue, green, and orange lines represent ≡SBOH + UO22+ ⇄ ≡SBOUO2+ + H+, ≡SBOH + UO22+ + H2O ⇄ ≡SBOUO2OH + 2 H+, ≡SBOH + UO22+ + 3H2O ⇄ ≡SBOUO2(OH)32− + 4 H+, and ≡SBOH + UO22+ + Cl− ⇄ ≡SBOUO2Cl + H+, respectively. The black line represents the total of the modeled sorption.

The 2SPNE SC/CE model for biotite predicted well the pHm and I dependences of U(VI) sorption on granite observed in this study. In acidic conditions, SBOH + UO22+ = SBOUO2+ + H+ is a dominant reaction for the sorption of U(VI), which agrees with the report by Jin et al.[Citation11] However, they reported the log K0 as −0.1, which is smaller than the value found in this work. Furthermore, the other reactions they considered were different from those in this study.[Citation11] One possible reason might be that they did not consider the protolysis reactions of the mineral compositions of granite.
Another reaction under acidic conditions that we considered was SBOH + UO22+ + Cl− = SBOUO2Cl + H+. The contribution of this reaction to the sorption of U(VI) increased with an increase in ionic strength because high ionic strength with high chloride concentrations could promote the complexation of U(VI) with chloride. The contribution of these two reactions reduced with increasing pHm. We found that SBOH + UO22+ + H2O = SBOUO2OH + 2H+ is a possible reaction to fit the experimental data in neutral pH conditions. Under alkaline conditions, we found that the reaction SBOH + UO22+ + 3H2O = SBOUO2(OH)32− + 4H+ dominated the sorption of U(VI). Consequently, the four inner-sphere surface complexation reactions shown in predicted well the pHm and I dependences of U(VI) sorption on granite in this study.
The contributions of ion exchange and outer-sphere surface complexation were found to be negligible, and this may also explain the insignificant I dependence shown in . The surface complexation reaction, SBOH + UO22+ + Cl− = SBOUO2Cl + H+, was found to contribute to the sorption onto granite (namely, biotite), but not onto MX-80. The reason is not clear at present. This may be a topic for a future study.
On the other hand, since chlorite is generally known to be generated by hydrothermal alteration of biotite, not only the structure but also the sorption characteristics of chlorite might be similar to that of biotite. Therefore, although the composition of chlorite (1.4%) is about a half of that of biotite (3%), it might be possible that chlorite also dominates the sorption of U(VI) on granite. In this study, we also applied the 2SPNE SC/CE model to U(VI) sorption on chlorite. The surface characteristics of chlorite in the Lac du Bonnet batholith granite have not been studied, so the data of chlorite from other granites were used in this study. The protolysis reaction constants of the chlorite being used in this study are summarized in .[Citation50] The site density of 2.3 sites/nm2[Citation51] was used. In this case, we assumed that three minerals (feldspar, quartz, and chlorite) contribute to the sorption of U(VI).
TABLE VI Protolysis Reaction Constants of Chlorite Used in This Study
shows the result at I = 0.24 m when chlorite is considered instead of biotite. Other fitting results for I = 0.05, 0.1, 1, and 3 m are shown in the supplementary materials (Figs. S9 through S12). The optimized values of log K0 for granite/chlorite obtained in this study are summarized in . Although chlorite was considered instead of biotite as the sorbent, the predicted results by 2SPNE SC/CE were found to agree well with the pHm and I dependences of U(VI) sorption on granite observed in this study. It was also found that the surface complexation reactions to reproduce the experimental results were the same four inner-sphere surface complexation reactions as in the case of biotite. This suggests that U(VI) sorbed both on biotite and chlorite in our experimental conditions.
TABLE VII Surface Complexation Constants Log K0 of U(VI) Sorption on Granite/Chlorite Obtained in This Work
Fig. 6. Fitting results for sorption of U(VI) onto granite/chlorite at ionic strength of 0.24 m in Ca-Na-Cl solution with the 2SPNE SC/CE model. The red, blue, green, and orange lines represent ≡SChOH + UO22+ ⇄ ≡SChOUO2+ + H+, ≡SChOH + UO22+ + H2O ⇄ ≡ SChOUO2OH + 2 H+, ≡SChOH + UO22+ + 3H2O ⇄ ≡SChOUO2(OH)32− + 4 H+, and ≡SChOH + UO22+ + Cl− ⇄ ≡SChOUO2Cl + H+, respectively. The black line represents the total of the modeled sorption.

If the experiments using relatively high concentrations of U(VI) are carried out and additional experimental data, such as from electron probe micro analyzer and scanning electron microscopy/energy-dispersive X-ray analysis, can be obtained in the future, a more detailed discussion will be possible.
IV. CONCLUSION
A systematic study was carried out for the sorption behavior of U(VI) onto MX-80 and granite in Ca-Na-Cl solutions with an ionic strength of 0.05 to 3 m. It was found that 7 days were sufficient for both MX-80 and granite to reach sorption equilibrium. We observed that U(VI) sorption was independent of I in the range of 0.05 to 3 m for both MX-80 and granite. U(VI) sorption on MX-80 showed that U(VI) sorption gradually increased with pHm until pHm = 6, where it reached its maximum value. Then it decreased slightly as pHm kept increasing until pHm = 8, and thereafter became constant. U(VI) sorption onto granite increased with pHm, reached the maximum between pHm = 7 and 8, and then slightly decreased as the pHm kept increasing.
After comparing the results of U(VI) sorption in Ca-Na-Cl solutions and CR-10 reference groundwater, we found that the impact of minor ions, such as Mg2+ and Sr2+, on the sorption of U(VI) onto both MX-80 and granite was small. The 2SPNE SC/CE model was able to successfully predict the sorption of U(VI) onto MX-80 and granite. Three inner-sphere surface complexations were identified for U(VI) sorption onto MX-80. For sorption onto granite, four inner-sphere surface complexations of U(VI) sorption on biotite or chlorite could fit the experimental data of U(VI) sorption on granite. This may suggest that U(VI) sorbed both on biotite and chlorite in our experimental conditions. The optimized value of log K0 of each surface complexation reaction was evaluated.
Supplementary Materials
Download MS Word (1.1 MB)Acknowledgments
This work was funded by the NWMO of Canada.
Disclosure Statement
No potential conflict of interest was reported by the authors.
Supplementary material
Supplemental data for this article can be accessed online at https://doi.org/10.1080/00295450.2023.2300900
Additional information
Funding
References
- H. GECKEIS et al., “Mineral-Water Interface Reactions of Actinides,” Chem. Rev., 113, 2, 1016 (2013); https://doi.org/10.1021/cr300370h.
- J. K. LEE et al., “Development of a Web-Based Sorption Database (KAERI-SDB) and Application to the Safety Assessment of a Radioactive Waste Disposal,” Nucl. Eng. Des., 241, 12, 5316 (2011); https://doi.org/10.1016/j.nucengdes.2011.09.045.
- “A Study of the Isolation for Geologic Disposal of Radioactive Wastes,” Waste Isolation Systems Panels, Board on Radioactive Waste Management, National Academy of Science (1983).
- “Postclosure Safety Assessment of a Used Fuel Repository in Crystalline Rock,” Technical Report NWMO-TR-2017-02, Nuclear Waste Management Organization (2017).
- M. BEHAZIN et al., “State of Science Review of Sulfide Production in Deep Geological Repositories for Used Nuclear Fuel,” Technical Report NWMO-TR-2021-18, Nuclear Waste Management Organization (2021).
- M. H. BAIK, S. P. HYUN, and P. S. HAHN, “Surface and Bulk Sorption of Uranium(VI) onto Granite Rock,” J. Radioanal. Nucl. Chem., 256, 1, 11 (2003); https://doi.org/10.1023/A:1023331521718.
- M. H. BAIK et al., “Contribution of Minerals to the Sorption of U(VI) on Granite,” Radiochim. Acta, 92, 9–11, 663 (2004); https://doi.org/10.1524/ract.92.9.663.54980.
- M. H. BAIK, W. J. CHO, and P. S. HAHN, “Sorption of U(VI) onto Granite Surfaces: A Kinetic Approach,” J. Radioanal. Nucl. Chem., 260, 3, 495 (2004); https://doi.org/10.1023/B:JRNC.0000028207.55356.ec.
- C. NEBELUNG and V. BRENDLER, “U(VI) Sorption on Granite: Prediction and Experiments,” Radiochmica Acta, 98, 9–11, 621 (2010); https://doi.org/10.1524/ract.2010.1762.
- C. RICHTER et al., “Macroscopic and Spectroscopic Characterization of Uranium(VI) Sorption onto Orthoclase and Muscovite and the Influence of Competing Ca2+,” Geochim. Cosmochim. Acta, 189, 143 (2016); https://doi.org/10.1016/j.gca.2016.05.045.
- Q. JIN et al., “Surface Complexation Modeling of U(VI) Adsorption on Granite at Ambient/Elevated Temperature: Experimental and XPS Study,” Chem. Geol., 433, 81 (2016); https://doi.org/10.1016/j.chemgeo.2016.04.001.
- X. GAO et al., “Sorption Characteristic of Uranium(VI) Ion onto K-feldspar,” Applied Radiation and Isotopes, 128, 311 (2017); https://www.sciencedirect.com/science/article/abs/pii/S0969804317304116.
- Z. YIN et al., “Retardation of Hexavalent Uranium in Muscovite Environment: A Batch Study,” Radiochim. Acta, 106, 7, 559 (2018); https://doi.org/10.1515/ract-2017-2888.
- E. K. SKIERSZKAN et al., “Geochemical Controls on Uranium Release from Neutral-pH Rock Drainage Produced by Weathering of Granite, Gneiss, and Schist,” Minetals, 10, 1104 (2020); https://doi.org/10.3390/min10121104.
- D. PAN et al., “Insights into Sorption Speciation of Uranium on Phlogopite: Evidence from TRLFS and DFT Calculation,” J. Hazard. Mater., 427, 128164 (2022); https://doi.org/10.1016/j.jhazmat.2021.128164.
- R. T. PABALAN and D. R. TURNER, “Uranium(6+) Sorption on Montmorillonite: Experimental and Surface Complexation Modeling Study,” Aquat. Geochem., 2, 3, 203 (1997); https://doi.org/10.1007/BF00119855.
- M. H. BRADBURY and B. BAEYENS, “Sorption of Eu on Na- and Ca-Montmorillonites: Experimental Investigations and Modelling with Cation Exchange and Surface Complexation,” Geochim. Cosmochim. Acta, 66, 13, 2325 (2002); https://doi.org/10.1016/S0016-7037(02)00841-4.
- S. AMAYRI et al., “Distribution Coefficients for the Sorption of Th, U, Np, PU, and Am on Opalinus Clay,” Radiochim. Acta, 104, 1, 33 (2016); https://doi.org/10.1515/ract-2015-2409.
- E. L. TRAN et al., “Uranium and Cesium Sorption to Bentonite Colloids Under Carbonate-Rich Environments: Implications for Radionuclide Transport,” Sci. Total Environ., 643, 260 (2018); https://doi.org/10.1016/j.scitotenv.2018.06.162.
- M. A. GLAUS, S. FRICK, and L. R. VAN LOON, “A Coherent Approach for Cation Surface Diffusion in Clay Minerals and Cation Sorption Models: Diffusion of Cs+ and Eu3+ in Compacted Illite as Case Examples,” Geochim. Cosmochim. Acta, 274, 79 (2020); https://doi.org/10.1016/j.gca.2020.01.054.
- L. FRALOVA et al., “Effect of Organic Compounds on the Retention of Radionuclides in Clay Rocks: Mechanisms and Specificities of Eu(III), Th(IV), and U(VI),” Appl. Geochem., 127, 104859 (2021); https://doi.org/10.1016/j.apgeochem.2020.104859.
- M. STOCKMANN et al., “New Insights into U(VI) Sorption onto Montmorillonite from Batch Sorption and Spectroscopic Studies at Increased Ionic Strength,” Sci. Total Environ., 806, 150653 (2022); https://doi.org/10.1016/j.scitotenv.2021.150653.
- T. HUITTI, M. HAKANEN, and A. LINDBERG, “Sorption of Cesium, Radium, Protactinium, Uranium, Neptunium and Plutonium on Rapakivi Granite,” POSIVA-96-23, University of Helsinki (1996).
- K. SCHMEIDE et al., “Interaction of U(VI) with Äspö Diorite: A Batch and in situ ATR FT-IR Sorption Study,” Appl. Geochem., 49, 116 (2014); https://doi.org/10.1016/j.apgeochem.2014.05.003.
- J. A. BERRY, M. YUI, and A. KITAMURA, “Sorption Studies of Radioelements on Geological Materials,” JAEA-Research 2007-074, Japan Atomic Energy Agency (2007).
- P. BERTETTI, “Determination of Sorption Properties for Sedimentary Rocks Under Saline, Reducing Conditions—Key Radionuclides,” Technical Report NWMO TR-2-16-08, Nuclear Waste Management Organization (2016).
- P. ČERNÝ et al., “The Archean Lac du Bonnet Batholoth, Manitoba: Igneous History, Metamorphic Effects, and Fluid Overprinting,” Geochim. Cosmochim. Acta, 51, 3, 421 (1987); 10.1016/0016-7037(87)90059-7.
- M. ALTMAIER et al., “Solid-Liquid Equilibria of Mg(OH)2(cr) and Mg2(OH)3Cl·4H2O(cr) in the System Mg-Na-H-OH-Cl-H2O at 25°C,” Geochim. Cosmochim. Acta, 67, 19, 3595 (2003); https://doi.org/10.1016/S0016-7037(03)00165-0.
- M. ALTMAIER, V. NECK, and T. FANGHÄNEL, “Solubility of Zr(IV), Th(IV) and Pu(IV) Hydrous Oxides in CaCl2 Solutions and the Formation of Ternary Ca-M(IV)-OH Complexes,” Radiochim. Acta, 96, 9–11, 541 (2008); https://doi.org/10.1524/ract.2008.1535.
- S. NAGASAKI et al., “Sorption Behaviour of Np(IV) on Illite, Shale and MX-80 in High Ionic Strength Solutions,” J. Radioanal. Nucl. Chem., 313, 1, 1 (2017); https://doi.org/10.1007/s10967-017-5290-2.
- D. L. PARKHURST and C. A. J. APPELO, “Description of Input and Examples for PHREEQC Version 3—A Computer Program for Speciation, Batch-Reaction, One-Dimensional Transport, and Inverse Geochemical Calculations,” in U.S. Geological Survey Techniques and Methods, Book 6, Chap. A43, U.S. Geological Survey (2013).
- “Thermodynamic DataBase,” Japan Atomic Energy Agency (Apr. 27, 2021 Updated); https://migrationdb.jaea.go.jp/english.html (current as of April 29, 2023).
- M. H. BRADBURY and B. BAEYENS, “A Mechanistic Description of Ni and Zn Sorption on Na-Montmorillonite Part II: Modelling,” J. Contam. Hydrol., 27, 3–4, 223 (1997); https://doi.org/10.1016/S0169-7722(97)00007-7.
- M. H. BRADBURY and B. BAEYENS, “Modelling the Sorption of Mn(II), Co(II), Ni(II), Zn(II), Cd(II), Eu(III), Am(III), Sn(IV), Th(IV), Np(V) and U(VI) on Montmorillonite: Linear Free Energy Relationships and Estimates of Surface Binding Constants for Some Selected Heavy Metals and Actinides,” Geochim. Cosmochim. Acta, 69, 4, 875 (2005); https://doi.org/10.1016/j.gca.2004.07.020.
- M. H. BRADBURY and B. BAEYENS, “Sorption Modelling on Illite Part I: Titration Measurements and the Sorption of Ni, Co, Eu and Sn,” Geochim. Cosmochim. Acta, 73, 4, 990 (2009); https://doi.org/10.1016/j.gca.2008.11.017.
- M. H. BRADBURY and B. BAEYENS, “Sorption Modelling on Illite. Part II: Actinide Sorption and Linear Free Energy Relationships,” Geochim. Cosmochim. Acta, 73, 4, 1004 (2009); https://doi.org/10.1016/j.gca.2008.11.016.
- S. NAGASAKI, “Sorption Properties of Np on Shale, Illite and Bentonite Under Saline, Oxidizing and Reducing Conditions,” NWMO-TR-2018-02, Nuclear Waste Management Organization (2018).
- A. WALKER et al., “Sorption of Se(-II) on Illite, MX-80 Bentonite, Shale, and Limestone in Na-Ca-Cl Solution,” Nucl. Eng. Technol., 54, 5, 1616 (2022); https://doi.org/10.1016/j.net.2021.10.039.
- J. YANG et al., “Sorption of Eu on MX-80 Bentonite in Na-Ca-Cl Solutions,” J. Nucl. Fuel Cycle Waste Technol., 20, 2, 151 (2022); https://doi.org/10.7733/jnfcwt.2022.012.
- J. RACETTE et al., “Influence of Ca-Na-Cl Physicochemical Solution Properties on the Adsorption of Se(-II) onto Granite and MX-80 Bentonite,” Nucl. Eng. Technol., 55, 10, 3831 (2023); https://doi.org/10.1016/j.net.2023.06.049.
- L. CIAVATTA, “The Specific Interaction Theory in the Evaluating Ionic Equilibria,” Annali di Chimica (Rome), 70, 551 (1980).
- A. WALKER et al., “Sorption of Se(-II) on Illite, MX-80 Bentonite, Shale, and Limestone in Na-Ca-Cl Solutions,” Nucl. Eng. Technol., 54, 5, 1616 (2022); https://doi.org/10.1016/j.net.2021.10.039.
- M. MARQUES FERNANDES et al., “U(VI) Sorption on Montmorillonite in the Absence and Presence of Carbonate: A Macroscopic and Microscopic Study,” Geochim. Cosmochim. Acta, 93, 262 (2012); https://doi.org/10.1016/j.gca.2012.04.017.
- “K-feldspar and Plagioclase Feldspar,” Department of Geology and Planetary Science, University of Pittsburgh; https://sites.pitt.edu/~cejones/GeoImages/1Minerals/1IgneousMineralz/Feldspars.html (current as of Apr. 29, 2023).
- “Feldspars—All About the Plagioclases and K-feldspars,” MineralExpert.org; https://mineralexpert.org/article/overview-plagioclases-feldspars (current as of Apr. 29, 2023).
- Y. IIDA et al., “Sorption Behavior of Thorium onto Granite and Its Constituent Minerals,” J. Nucl. Sci. Technol., 53, 10, 1573 (2016); https://doi.org/10.1080/00223131.2016.1138901.
- Å. ZAZZI, A.-M. JACKOBSSON, and S. WOLD, “Ni(II) Sorption on Natural Chlorite,” Appl. Geochem., 27, 6, 1189 (2012); https://doi.org/10.1016/j.apgeochem.2012.03.001.
- S. Y. LEE, M. H. BAIK, and Y. B. LEE, “Adsorption of Uranyl Ions and Microscale Distribution on Fe-Bearing Mica,” Appl. Clay Sci., 446, 3–4, 259 (2009); https://doi.org/10.1016/j.clay.2009.03.002.
- S. Y. LEE et al., “Adsorption of U(VI) Ions on Biotite from Aqueous Solutions,” Appl. Clay Sci., 46, 3, 255 (2009); https://doi.org/10.1016/j.clay.2009.08.013.
- J. A. DAVIS and D. B. KENT, “Surface Complexation Modeling in Aqueous Geochemistry,” Reviews in Mineralogy, Vol. 23, M. F. HOCHELLA JR and A. F. WHITE, Eds., pp. 117–260, Mineral-Water Interface Geochemistry (1990); https://doi.org/10.1515/9781501509131-009.
- W. ZHOU et al., “Macroscopic and Spectroscopic Characterization of U(VI) Sorption on Biotite,” Chemosphere, 255, 126942 (2020); https://doi.org/10.1016/j.chemosphere.2020.126942.