Abstract
The objective of the present study is to show that it is feasible to establish the breed-and-burn (B&B) mode of operation with rotational fuel shuffling in the S-PRISM core based on neutronic and thermal-hydraulic analyses. The results quantified the impact of major core design choices on the criticality of a core that uses sodium as a coolant material and HT9 steel as structural material. The design variables examined include the binary metallic fuel U-Zr with different weight percentages of zirconium as well as different core heights and fuel rod pitch-to-diameter ratios (P/Ds) in the fuel assembly. We found that a core using the binary metallic fuel U-Zr with 2 wt% zirconium, with a core height of 200 cm, a P/D of 1.086, and a core power of 400 MW(thermal), could overcome some major design constraints.
It was also found that with shuffling intervals of 1125 to 1250 days, the core with rotational fuel shuffling was critical in the equilibrium state, and the possible average discharged burnup was from 274.8 to 305.3 GWd/ton HM. Reactor characteristics such as neutron flux and power profile were almost stable during the equilibrium cycle. A steady-state thermal-hydraulic analysis was performed for the hottest channel in the core. It revealed that both the fuel and cladding maximum temperatures were less than the melting point of the fuel and the chemical interaction temperature of the HT-9, respectively. The mixed coolant outlet temperature was somewhat below the temperature usually observed in sodium-cooled fast reactors. Thus, it appears that the S-PRISM can be principally designed to have a B&B core.
I. INTRODUCTION
The breed-and-burn (B&B) mode of operation, first proposed by Feinberg in 1958, is made self-sustainable by producing fissile materials such as 239Pu (or 233Pu) from 238U (or 232Th) during burnup.Citation1 In a B&B reactor, namely, a reactor using the B&B mode of operation, the fissile elements are first bred into the fuel by neutrons produced in the reactor. The resulting bred fuel is then directly burned in the same reactor to sustain the chain reaction and supply neutrons for breeding additional fuel. Therefore, B&B reactors can operate using primarily fertile fuel but also reload fuel, such as natural or depleted uranium, low-enriched uranium, used light water reactor fuel, or thorium.
Since a B&B core contains fertile materials and fission products (FPs), the neutron economy in the core must be improved to maintain criticality in operation, unlike other types of reactors with the same core size. To maintain critical operation, the feed fuel must be burned to a sufficient level such that the rate of neutron production increases with burnup and overtakes that of neutron absorption. A report by Petroski et al. in 2010 (CitationRef. 2) discusses the minimum burnup requirement of B&B reactors and shows that it is feasible to achieve criticality with the B&B mode of operation in three dimensions.
Recent B&B reactors studied at the Tokyo Institute of Technology are similar in concept to the University of California, BerkeleyCitation2,Citation3 (UCB), the Tokyo Institute of Technology CANDLE (CitationRef. 4), and the Terra Power Traveling WaveCitation5 reactors. In order to achieve criticality with the B&B mode of operation and a stable power profile in the core, the rotational-fuel-shuffling concept has been proposed.Citation6,Citation7 In this fuel-shuffling concept, fresh fuel is first loaded from the periphery of the core and then moved toward the center. After that, the fuel is moved outward and then discharged nearly at the periphery of the core.
In the previous reports,Citation6,Citation7 the rotational-fuel-shuffling concept was applied to small lead-bismuth−cooled nuclear reactors of 450 MW(thermal). High-reactivity fuels were located stably at the core center where the high-neutron-importance region was placed. As a result, the B&B region remained stationary, and the power profile and neutron flux distribution were thereby almost unchanged during operation in the equilibrium state.
In comparison to sodium coolant, lead-based coolant has several potential advantages, such as a higher boiling temperature and chemical nonreactivity with air and water. However, lead can be more corrosive and erosive than sodium if the lead chemistry is not adequately controlled. In addition, sodium coolant has a lower melting temperature, higher specific heat, and higher thermal conductivity than lead-based coolant.
Sodium-cooled B&B reactors have been intensively studied at the UCB in Project No. 09-769 (CitationRef. 8) and Project No. 12-3486 (CitationRef. 9). In these projects, the S-PRISM core developed by General ElectricCitation10 was chosen as a reference core. This reference core design was also chosen by the Argonne National Laboratory in its recent studies of the Advanced Burner Reactor.Citation11 The S-PRISM core design is well known for reference designs because of its detailed and practical design for an advanced reactor system. Therefore, it is valuable and worthy to apply the rotational-fuel-shuffling concept for a viable reactor design that is based on the S-PRISM core and/or that could fit within the S-PRISM reactor vessel developed by General Electric. The purpose of the present study was to assess the feasibility of establishing the B&B mode of operation with a rotational-fuel-shuffling scheme in a sodium-cooled reactor based on the S-PRISM reactor design.
II. REACTOR CONCEPT
The S-PRISM is a General Electric extension of the PRISM reactor developed by joint efforts of the U.S. Department of Energy and General Electric. The S-PRISM design has been reviewed and analyzed by many other organizations. S-PRISM is larger, has a simpler design, and is more economical than PRISM. S-PRISM has a modular design. The core has design options for metal and oxide fuel to generate a total power of 1000 MW(thermal) (CitationRef. 12). In this study, the core design that uses binary metallic fuel was selected as a reference core design. The reference S-PRISM metal-fueled core layout is composed of 138 driver fuel assemblies, 97 blanket fuel assemblies, 12 control assemblies, 6 gas expansion modules, 126 reflector assemblies, and 72 shield assemblies. It has an equivalent core diameter of 2.71 m and an equivalent reactor diameter of 3.62 m (CitationRef. 12). It uses binary metallic fuel U-10 wt% Zr and has a smear density of 75%. The structural and cladding material is HT-9 ferritic-martensitic alloy. The coolant and bond material is sodium. The fuel assemblies have a hexagonal geometry with a pitch; interduct gap; and duct thicknesses of 16.142, 0.432, and 0.394 cm, respectively. The fuel assemblies are made of 271 fuel rods with a pin pitch-to-diameter ratio (P/D) of 1.191. The fuel rods have a pin diameter, cladding thickness, and active fuel length of 0.744, 0.0559, and 101.6 cm, respectively. More detail on the S-PRISM metal-fueled core design can be found in CitationRef. 13.
As mentioned in Sec. I, because the B&B core contains fertile materials and FPs, the neutron economy in the core should be improved to maintain criticality in the B&B mode of operation of a reactor with the same core size. In this study, in order to determine the upper possible bound on the neutron economy of the metal-fueled core, fuel assemblies replaced the 12 control assemblies, 6 gas expansion modules, 126 reflector assemblies, and 72 shield assemblies in the reference core layout, and the fuel assembly at the core center was filled with coolant. As a result of this core layout modification, the metal-fueled core layout in this study was composed of 252 fuel assemblies, 1 coolant channel, 126 reflector assemblies, and 72 shield assemblies. The neutron economy of a B&B core deteriorates with reductions in the leaded heavy metal, active core height, and fuel volume fraction in the core. Thus, this study examined the binary metallic fuel U-Zr with different weight percentages of zirconium, core heights, and fuel rod P/D in the fuel assembly as design variables. The height of the gas plenum in this present study is the same as that of the S-PRISM metal-fueled core design. But, it was possible to shorten the gas plenum length by applying the vented fuel concept to reduce the overall fuel rod length as in the research.Citation14 Thereby, it would reduce potential fuel failure from fission gas in the gas plenum and pressure drop as well.
To estimate the maximum achievable power for the B&B core that would operate up to relatively high burnups, the following thermal neutronic and hydraulic constraints were accounted forCitation8,Citation9,Citation13,Citation15:
minimum effective multiplication factor (keff) over cycle: 1.000 to ensure to maintain the chain reaction in the core
coolant inlet/outlet temperatures: 355°C/510°C
maximum coolant velocity: 12 m/s to reduce the corrosion rate of materials during operation
maximum cladding temperature: 650°C, which is lower than the melting temperature of the clad and fuel at the eutectic point
maximum fuel temperature: 800°C, which is lower than the melting temperature of the fuel
maximum core coolant pressure drop: 1.0 MPa, assuming the pressure drop through the core is at least twice the value commonly used for the sodium fast reactor cores.
III. CALCULATION METHOD
We used the Monte Carlo Serpent neutronic codeCitation16 with the ENDF-B/VII nuclear data libraryCitation17 for the neutronic calculations with 5000 neutron histories per cycle and 200 active cycles while skipping the first 20 cycles for statistical treatment to obtain a target statistical error in keff of ~150 pcm. The cross-section library was utilized with the average temperatures of fuel, cladding, and coolant in the operating condition, which were set as 800, 700, and 700 K, respectively. The burnup calculation was carried out for the one-sixth core region in the azimuthal direction. The axial direction was symmetrically divided into 11 burnup regions.
The fresh fuel asrefsemblies were loaded from outside the core. The fuels were moved inward and reached the core center after the first 25 fuel-shuffling steps and then moved outward and discharged after the next 17 fuel-shuffling steps. shows the fuel-shuffling pattern and position identifier (ID). Each fuel assembly position was assigned a position ID, and the fuel-shuffling flow was from fuel assembly position IDs 1 through 42. In order to initiate the chain reaction in the B&B reactor, the core required to be loaded with an adequate amount of fissile fuel, such as enriched uranium, plutonium, or transuranium elements extracted from used nuclear fuel. Thereafter, continued operation of the core is possible while being fed with depleted uranium or natural uranium only. In order to assess the feasibility of establishing the B&B mode of operation with a rotational fuel-shuffling scheme, the present study focused on only the equilibrium core. In this work, the period of time in which fuel assemblies were either removed from or added to the core was defined as one fuel cycle length. The average fuel burnup in the equilibrium state was estimated as follows:
Fig. 1. Fuel-shuffling pattern and position ID of fuel assemblies in the core. In this rotational-fuel-shuffling scheme, a fresh fuel assembly is loaded at position 1. The fuel assembly is moved inward after the first 25 fuel-shuffling steps and then moved outward and discharged after 17 fuel-shuffling steps at position 42
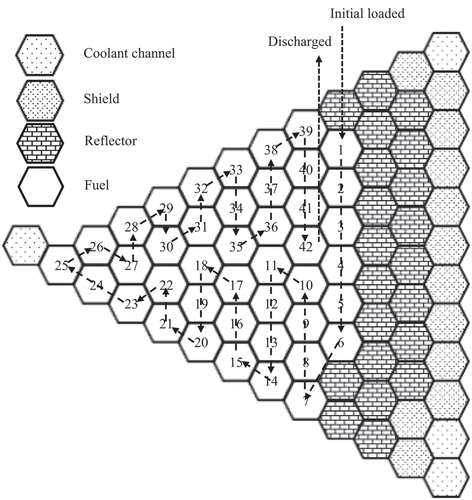
Average fuel burnup in MWd/ton = [Core power in MW(thermal)] × (Fuel cycle length in days)/(Consumed uranium per cycle in tons).
For the calculation procedure with the fuel movement method used to achieve the equilibrium state of the B&B reactor, a computer interface program was developed to automatically conduct core burnup calculations from cycle to cycle. In this procedure, the equilibrium condition is determined based on the convergence of the keff; neutron flux; and nuclide number density values of some isotopes such as 239Pu, 235U, and 238U. The calculation procedure was performed as follows:
Step 1: Design parameters such as core size, fuel assemblies, fuel-shuffling pattern, operational conditions, and fuel cycle length were prepared in the input file.
Step 2: The core burnup calculation was performed to obtain end-of-cycle fuel compositions.
Step 3: The input file was updated with the new fuel compositions obtained in the previous calculational cycle along with the fuel-shuffling pattern. The core burnup calculation for the next cycle was performed using the new input file.
Step 4: Steps 2 and 3 were repeated until the core reached equilibrium condition.
The thermal-hydraulic analysis was performed using COMSOL 5.4 multi-physics software.Citation18 The geometry model was developed with two-dimensional axisymmetry with R-Z coordinates consisting of the fuel slug, bond layer, cladding, coolant channel, lower end plug, plenum, and upper end plug, as in CitationRefs. 7, Citation19, Citation20, and Citation21.
provides the core layout and model geometry of the subchannel analysis for the whole length of the reactor. The hexagonal cell lattice was simplified so that it was equivalent to a cylinder, as can be seen in . This cell lattice model was the hottest channel of a fuel pin in the core. The sodium coolant flowed vertically upward through the cooling channel. For the conservative boundary condition, the outer boundary of the model geometry was specified as an adiabatic boundary, that is, with no heat crossing it.
In the thermal-hydraulic analysis, the power distribution of the fuel rod in the axial direction is obtained from the neutronic analysis. The power generation from the fuel rod at the center of the core can be calculated from the power density in the fuel zone in the core center with the assumption that power is generated from the fuel pin only. Other power generation sources, such as those in the bond layer, cladding, coolant, and reactor structure by the gamma heating effect, were ignored, as in CitationRefs. 7, Citation19, Citation20, and Citation21.
In order to improve accuracy in analysis, the new coupled neutron and gamma heating calculation scheme to determine pin and duct wall power distributions in sodium fast reactor cores using the VARIANT transport solutions was introduced in research.Citation22 As reported in the research,Citation22 the maximum assembly power error was ~1% in the fuel assemblies and ~2% in the instrumented structure assemblies, and the maximum error in the pin segment powers in an axial node of the fuel assembly was ~4% (CitationRef. 22). And, the maximum error in pin segment powers was increased due to the lower power level and the relatively large error in the nodal power of the VARIANT solution. In order to improve accuracy more, it was suggested that additional validation tests would also be performed by comparing the calculated heating rates with the measured data.Citation22
IV. RESULTS AND DISCUSSION
IV.A. Effects of the Different Metallic Fuel Types and P/Ds
Since the B&B core contains fertile materials and FPs, it is difficult to maintain criticality in a B&B reactor with the same core size. Thus, a maximized heavy metal density and fuel volume fraction in the core are preferable for a B&B reactor design. The effective heavy metal density in the core depends on the weight percent of Zircaloy and on the fuel volume fractions in the fuel assemblies. Therefore, the choice of binary metallic fuel with different weight percentages of Zircaloy and different P/Ds would significantly impact criticality in the B&B core. In the present study, the metallic fuels with the different weight fractions of 2%, 6%, and 10% zirconium, which have been tested in researchCitation23 and also investigated in research,Citation24 were examined. It was reported that these metallic fuels would provide acceptable fuel metallurgical and chemical performance over the burnup range of relevance for B&B reactors.Citation24 To investigate the effect of the choice of fuel type on attaining critical B&B cores in the equilibrium state without changing the sizes of the reactor and fuel assemblies, four B&B cores loaded with different fuel assembly configurations were examined:
a core loaded with fuel of U-10 wt% Zr and P/D = 1.191 in an active core height of 250 cm (i.e., 271 fuel rods in each fuel assembly), referred to as “U-10Zr & P/D = 1.191”
a core loaded with fuel of U-6 wt% Zr and P/D = 1.191 in an active core height of 250 cm, referred to as “U-6Zr & P/D = 1.191”
a core loaded with fuel of U-6 wt% Zr and P/D = 1.086 in an active core height of 250 cm (i.e., 331 fuel rods in each fuel assembly), referred to as “U-6Zr & P/D = 1.086”
a core loaded with fuel of U-2 wt% Zr and P/D = 1.086 in an active core height of 250 cm, referred to as “U-2Zr & P/D = 1.086.”
In these examinations, the fuel cycle length was adjusted in order to determine whether there was any possibility of achieving criticality in the investigated B&B reactors. compares the minimum keff over cycle in the equilibrium state of each of the four cores. The minimum keff over cycle increased as the heavy metal density increased in the core. Among the four examined cores, U-2Zr & P/D = 1.086 was the only one that could achieve criticality in the equilibrium state. The analysis results showed that the minimum and maximum attainable burnups of the U-2Zr & P/D = 1.086 core were 195.4 and 341.9 GWd/ton HM, respectively. These attainable burnups corresponded to fuel cycle lengths of 400 and 700 days, respectively. The core operating with a fuel cycle length of 570 days had the biggest excess reactivity in the equilibrium state.
summarizes the analysis results of the U-2Zr & P/D = 1.086 core. Even though the inlet coolant density was set to the maximum proper value, 12 m/s, the maximum fuel and cladding temperatures all met the design constraints. However, the outlet coolant temperature and the core coolant pressure drops were all higher than the design constraint values. This would be due to the combined effects of the high power densities in the core and of the high active core height.
TABLE I Summary of Analysis Results of the U-2Zr & P/D = 1.086 Core
IV.B. Effects of Core Height and Core Power
In Sec. IV.A, we investigated the effects of fuel type on attaining critical B&B cores in the equilibrium state. In these investigations, the active core size, conservatively assumed, was 250 cm. As the analyzed results showed, neither the outlet coolant temperature nor the core coolant pressure drops met the design constraint values. To model a more realistic active core, the 200-cm active core height model was examined. The fuel cycle length was adjusted to determine whether there was any possibility of achieving a proper core design in the investigated B&B reactors.
summarizes the analysis results for the core with a 200-cm active core height. The analyzed results showed that with a fuel cycle length of 450 to 500 days, the B&B reactor had sufficient reactivity to maintain criticality. In comparison with the 250-cm active core height, the minimum and maximum attainable burnups of the 200-cm active core were respectively higher and lower than those of the 250-cm active core because the 200-cm-tall active core had less total heavy metal. In B&B cores, the main portion of the power is generated at the middle core in the axial direction. The reduction of the active core height results in the flattening of the power distribution toward the axial core ends. Therefore, the maximum power density in the 200-cm active core was lower than that in the 250-cm active core. Consequently, the maximum fuel and cladding temperature, the outlet coolant temperature, and the core coolant pressure drops in the 200-cm active core were all lower than in the 250-cm active core. However, the outlet coolant temperature and the core coolant pressure drops were all still higher than the design constraint values. Especially, as shown in , the maximum power densities in the cores were above 450 W/cm3, that is, the peak power density in the available investigated B&B reactors.Citation25 Therefore, reducing core power seemed to be the logical choice for improving core design in this study.
TABLE II Summary of Analysis Results of the Core with a 200-cm Active Core Height
In the new approach, core power is reduced from 1000 to 400 MW(thermal). And, the same calculation methodology used above was also adopted for these analyses. We found that the B&B reactor had sufficient reactivity to maintain criticality with a fuel cycle length of 1125 to 1250 days, corresponding to peak power densities in the core of 403.2 and 356.7 W/cm3, respectively. The increase in fuel cycle length and the reduction of peak power densities were due to the reduction in core power. The detailed core performance of this proper core design is investigated in Sec. IV.C.
IV.C. Characteristics of the Equilibrium Cycle
In this section, we analyze the detailed core performance of the 400-MW(thermal) core with 200-cm active height for minimum and maximum achievable burnups. summarizes the analysis results of the 400-MW(thermal) core with 200-cm active height. The cores with fuel cycle lengths of 1125 and 1250 days are referred to as “1125 days” and “1250 days,” respectively. The average discharged burnup was from 247.8 to 305.3 GWd/ton HM. The reactivity swings at the beginning of the equilibrium cycle (BOEC) and at the end of the equilibrium cycle (EOEC) in both cases were less than 0.28%Δk/k.
TABLE III Summary of Analysis Results of the 400-MW(thermal) Core with a 200-cm Active Height
Regarding the isotopic composition of the discharged fuel, the fractions of 239Pu and 241Pu in the Pu discharged from the reactor were about 74.5% and 72.9%, respectively, when those cores reach the burnup required to sustain the B&B mode. The discharged fuel contains a significant amount of fissile plutonium, which poses a proliferation risk. The high concentration of the fed fissile in the Pu discharged from the reactor would also suggest that the discharged fuel could be reused to maximum fuel utilization for energy generation and proliferation resistance. In the B&B reactor, a high-neutron-flux region, approximated as a high-neutron-importance region based on the one-group perturbation theory, was located at the core center in this equilibrium cycle. Thus, the high-power-density region was retained at the core center region, as shown in . It is clear that the power density distribution was essentially constant from BOEC to EOEC. This would be a potentially easy and effective way to control the coolant flow rate of each fuel assembly.
Fig. 5. The power density in each fuel assembly: Each assembly is lined up by position ID, and the vertical axis shows the axial position
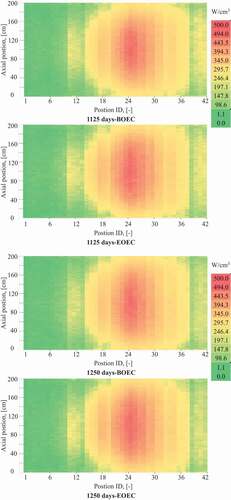
In the rotational-fuel-shuffling scheme, the fresh fuel assemblies were loaded at the core periphery and discharged at locations far from the core center. Therefore, the power distributions peaked at the core center and gradually decreased in the radial direction, as presented in . This reduced neutron leakage and improved the reactor’s neutron economy.
Because of the gradient of radial power distribution as shown in , the B&B reactor would adopt the orifice concept. In the orifice concept, the coolant flow rate of each fuel assembly will be adjusted by a coolant inlet orifice according to the assembly power as a function of the assembly location. In order to reduce the high change in the flow rate in the core, flow zoning devices, such as in research by Prakash et al. in 2011 (CitationRef. 26), would be applied to the current core design.
summarizes the analysis results for the 400-MW(thermal) core with 200-cm active height. The results showed that the minimum effective multiplication factor over cycle, the coolant inlet/outlet temperature, the maximum coolant velocity, the maximum cladding and fuel temperature, and the maximum core coolant pressure drop were all lower than the design constraint values. In the thermal-hydraulic analysis, this was performed for the no-wire-wrapped fuel model. This would have an effect on the accuracy in the analysis. However, it was reported in researchCitation27 that the wire-wrapped fuel design shows lower maximum pin temperature and lower maximum coolant temperature in the same flow rate condition in comparision with no-wire-wrapped fuel designs. Therefore, the results of the COMSOL-2D model would show larger design margin constraints than that of the analysis with wire-wrapped fuel designs. Regarding the pressure drop, one of the disadvantages of wire wrapping is increased pressure drop. But, it would be possible to reduce the pressure drop by applying the vented fuel concept as mentioned above.
One factor that should be considered for a more practical and realistic design is the high displacement per atom (dpa) values of discharged fuels. This is mainly due to the long fuel cycle length and long resident time of fuel in the core during operation. To reduce the maximum dpa value, the fuel cycle length of operation should be decreased or fuel reprocessing, such as the melt-refining process, should be applied.Citation19–21 These changes would result in an altered equilibrium state. Therefore, further analyses and optimizations for a practical reactor design must be performed in a future work.
The major challenge that the application of rotational fuel shuffling will face for the S-PRISM core is the high level of dpa’s of the HT-9 fuel clad. So far, the maximum radiation damage to which cladding and structural materials have been exposed is approximately 200 dpa in the Fast Flux Test Facility. However, this issue would be overcome by several promising approaches.
The first approach is to shorten the operational time and to reclad the fuel clad. In this approach, once the dpa of the fuel clad reaches 200 dpa, the reactor is stopped to allow recladding the fuel clad. The fuel with new clad is then reloaded, and the reactor continues to operate.
The second approach is to apply a melt-refining process similar to the one experimented with during the EBR-II project for fuel reconditioning. In this method, the reactor is stopped if the dpa of the fuel clad reaches the limit value. After the fuel is discharged from the reactor and is adequately cooled, the fuel is uncladded and melted. The gaseous and volatile FPs will be removed (and captured) in this process; there is no chemical separation. After making up fuel for the amount of fissioned heavy metal and losses during the melt-refining process, the resulting melt will be cast into new fuel slugs that will be inserted into a new clad. Fuel rods with the new clads are then fabricated into new fuel assemblies. The new fuel assemblies will provide the fuel loading for a subsequent recycling. The geometric parametric design of the fuel pins and fuel assemblies were unchanged after the melt-refining process.
As is mentioned in Sec. IV.B, the 12 control assemblies in the reference S-PRISM metal-fueled core layout were replaced with the binary metallic fuel assemblies. In order to operate the B&B reactor, the control systems were required to fulfill safety requirements. Once applying the control assemblies for the reactor, it would have an effect on the criticality of the cores with a rotational-fuel-shuffling scheme. However, it was mentioned in the previous studiesCitation19–21 that the excess reactivity would be increased by applying the melt-refining process because of FP removal during the reprocessing. Therefore, it was expected that criticality would be ensured throughout the cycle for the melt-refining core designed with the control systems.
V. CONCLUSIONS
In order to improve the neutron-economy B&B mode and to achieve a stable power profile in sodium-cooled fast reactors, rotational fuel shuffling was applied to the S-PRISM core. The results quantified the impacts of major core design choices on the criticality of a core that uses sodium as a coolant material and HT9 steel as structural material. The design variables examined include the binary metallic fuel U-Zr with different weight percentages of zirconium as well as different core heights and fuel rod P/Ds in the fuel assembly.
This preliminary analysis indicated the feasibility of establishing the B&B mode of operation with rotational fuel shuffling in the S-PRISM core. The core used the binary metallic fuel U-Zr with 2 wt% zirconium. To achieve criticality in the B&B strategy, the core height was increased from the reference 101.6 to 200 cm, and the P/D was decreased from the reference 1.191 to 1.086. In addition, the core power was decreased from the reference 1000 to 400 MW(thermal) to overcome some major design constraints. As a consequence, with shuffling intervals of 1125 to 1250 days, the core with rotational fuel shuffling was critical in the equilibrium state, and the possible average discharged burnup was from 274.8 to 305.3 GWd/ton HM. Reactor characteristics, such as neutron flux and power profile, were almost stable during the equilibrium cycle.
A steady-state thermal-hydraulic analysis was performed for the hottest channel in the core. It found that the fuel and cladding maximum temperatures were less than the melting point of the fuel and the chemical interaction temperature of the HT-9, respectively. The mixed coolant outlet temperature was somewhat below the temperature usually observed in sodium-cooled fast reactors. In a future work, further analyses will simulate the fuel recycling process for the core when dpa’s of the fuel clad reach the dpa limit. Investigation of a practical reactor design with this concept such as design of control systems and the initial core configuration, is also needed.
Acknowledgments
This work was supported by the Tokyo Tech World Research Hub Initiative (WRHI) Program of the Institute of Innovative Research, Tokyo Institute of Technology; by the Foundation for Science and Technology of Mongolia under grant number 2018/45; and by the Vietnam National Foundation for Science and Technology Development (NAFOSTED) under grant number 103.04-2019.37.
References
- S. M. FEINBERG, “Discussion Comment,” in Record of Proceedings Session B-10, Proc. Int. Conf. Peaceful Uses of Atomic Energy, Vol. 9, No. 2, p. 447, Geneva, Switzerland, 1958, United Nations.
- R. PETROSKI, B. FORGET, and C. FORSBERG, “Neutronic Evaluation of Breed-and-Burn Reactor Fuel Types Using an Infinite-Medium Depletion Approximation,” Proc. Physor 2010, Pittsburgh, Pennsylvania, May 9–14, 2010, American Nuclear Society (2010).
- F. HEIDET and E. GREENSPAN, “Neutron Balance Analysis for Sustainability of Breed and Burn Reactors,” Nucl. Sci. Eng., 171, 1, 13 (2012); https://doi.org/https://doi.org/10.13182/NSE10-114.
- H. SEKIMOTO and K. RYU, “A New Reactor Burnup Concept CANDLE,” Proc. Physor 2000, Pittsburgh, Pennsylvania, 2000, American Nuclear Society (2000).
- J. GILLELAND et al., “Novel Reactor Designs to Burn Non-Fissile Fuel,” Proc. 2008 Int. Congress Advances in Nuclear Power Plants (ICAPP 2008), Anaheim, California, June 8–12, 2008, American Nuclear Society (2008).
- T. OBARA, K. KUWAGAKI, and J. NISHIYAMA, “Feasibility of Burning Wave Fast Reactor Concept with Rotational Fuel Shuffling,” Proc. Int. Conf. Fast Reactors and Related Fuel Cycles: Next Generation Nuclear Systems for Sustainable Development (FR17)Yekaterinburg, Russian Federation, June 26–29, 2017, IAEA-CN245-051International Atomic Energy Agency, (2017).
- K. KUWAGAKI, J. NISHIYAMA, and T. OBARA, “Concept of Stationary Wave Reactor with Rotational Fuel Shuffling,” Nucl. Sci. Eng., 191, 2, 178 (2018); https://doi.org/https://doi.org/10.1080/00295639.2018.1463744.
- E. GREENSPAN, “Maximum Fuel Utilization in Fast Reactors Without Chemical Reprocessing,” Project No. 09-769,U.S. Department of Energy, Nuclear Energy University Programs (2012); https://neup.inl.gov/SiteAssets/Final%20%20Reports/FY%202009/NEUP_Project_No_09-769_Final_Report.pdf (current as of Dec. 7, 2020).
- E. GREENSPAN, “Advanced Burner Reactor with Breed-and-Burn Thorium Blankets for Improved Economics and Resource Utilization,” Project No. 12-3486, U.S. Department of Energy, Nuclear Energy University Programs (2015); https://neup.inl.gov/SiteAssets/Final%20%20Reports/FY%202012/12-3486%20NEUP%20Final%20Report.pdf#search=Project%20No%2E%2012%2D3486 (current as of Dec. 7, 2020).
- A. E. DUBBERLEY et al., “SuperPRISM Oxide and Metal Fuel Core Designs,” Proc. ICONE 8, Baltimore, Maryland, April 2–6, 2000.
- T. K. KIM et al., “Core Design Studies for a 1000 MWth Advanced Burner Reactor,” Ann. Nucl. Energy, 36, 3, 331 (2009); https://doi.org/https://doi.org/10.1016/j.anucene.2008.12.021.
- E. A. HOFFMAN, W. S. YANG, and R. N. HILL, “Preliminary Core Design Studies for the Advanced Burner Reactor over a Wide Range of Conversion Ratios,” ANL-AFCI-177, Argonne National Laboratory (2008).
- “Comparative Assessment of Thermophysical and Thermohydraulic Characteristics of Lead, Lead-Bismuth and Sodium Coolants for Fast Reactors,” International Atomic Energy Agency (2002).
- S. QVIST, J. HOU, and E. GREENSPAN, “Design and Performance of 2D and 3D-Shuffled Breed-and-Burn Cores,” Ann. Nucl. Energy, 85, 93 (2015); https://doi.org/https://doi.org/10.1016/j.anucene.2015.04.007.
- G. L. HOFMAN, L. C. WALTERS, and T. H. BAUER, “Metallic Fast Reactor Fuels,” Prog. Nucl. Energy, 31, 1–2, 83 (1997); https://doi.org/https://doi.org/10.1016/0149-1970(96)00005-4.
- J. LEPPÄNEN, “Serpent–A Continuous-Energy Monte Carlo Reactor Physics Burnup Calculation Code,” VTT Technical Research Centre of Finland (2015).
- M. B. CHADWICK et al., “ENDF/B-VII.0: Next Generation Evaluated Nuclear Data Library for Nuclear Science and Technology,” Nucl. Data Sheets, 107, 12, 2931 (2006); https://doi.org/https://doi.org/10.1016/j.nds.2006.11.001.
- “Multiphysics Modeling, Finite Element Analysis, and Engineering Simulation Software,” COMSOL 5.4a; https://www.comsol.com (current as of Apr. 5, 2020).
- V. K. HOANG, J. NISHIYAMA, and T. OBARA, “Design Concepts of Small CANDLE Reactor with Melt-Refining Process,” Prog. Nucl. Energy, 108, 233 (2018); https://doi.org/https://doi.org/10.1016/j.pnucene.2018.05.019.
- V. K. HOANG, J. NISHIYAMA, and T. OBARA, “Effects of Compensating for Fuel Losses During the Melt-Refining Process for a Small CANDLE Reactor,” Ann. Nucl. Energy, 135, 106969 (2020); https://doi.org/https://doi.org/10.1016/j.anucene.2019.106969.
- J. A. KARIM, J. NISHIYAMA, and T. OBARA, “Application of Melt and Refining Procedures in the CANDLE Reactor Concept,” Ann. Nucl. Energy, 90, 275 (2016); https://doi.org/https://doi.org/10.1016/j.anucene.2015.12.001.
- P. DENG et al., “Coupled Neutron and Gamma Heating Calculation Based on VARIANT Transport Solutions,” Nucl. Sci. Eng., 193, 12, 1310 (2019); https://doi.org/https://doi.org/10.1080/00295639.2019.1621617.
- D. C. CRAWFORD, D. L. PORTER, and S. L. HAYES, “Fuels for Sodium-Cooled Fast Reactors: US Perspective,” J. Nucl. Mater., 371, 202 (2007); https://doi.org/https://doi.org/10.1016/j.jnucmat.2007.05.010.
- S. QVIST and E. GREENSPAN, “Design Space Analysis for Breed-and-Burn Reactor Cores,” Nucl. Sci. Eng., 182, 197 (2016); https://doi.org/https://doi.org/10.13182/NSE14-135.
- C. D. SANZO and E. GREENSPAN, “A Search for Minimum Volume of Breed and Burn Cores,” Proc. ICAPP ’12, Chicago, Illinois, June 24–28, 2012, American Nuclear Society (2012).
- V. PRAKASH et al., “Experimental Qualification of Subassembly Design for Prototype Fast Breeder Reactor,” Nucl. Eng. Des., 241, 8, 3325 (2011); https://doi.org/https://doi.org/10.1016/j.nucengdes.2011.04.040.
- S. D. PARK et al., “Thermal-Hydraulic Analysis of a 7-Pin Sodium Fast Reactor Fuel Bundle with a New Pattern of Helical Wire Wrap Spacer,” Ann. Nucl. Energy, 114, 198 (2018); https://doi.org/https://doi.org/10.1016/j.anucene.2017.12.027.