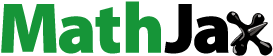
Abstract
Experimental analyses of 237Np, 241Am, and 243Am fission, as well as 237Np capture reaction rates, are conducted with the Serpent 2 code together with ENDF/B-VIII.0 and JENDL-5 using experimental data for the neutron spectra of thermal and intermediate regions obtained in the solid-moderated and solid-reflected cores with highly enriched uranium fuel at the Kyoto University Critical Assembly. Also, uncertainty quantification of the fission and capture reaction rate ratios of the test samples of 237Np, 241Am, and 243Am with reference samples of 235U and 197Au are evaluated by the MARBLE code system.
In terms of the fission reaction rate ratios of 237Np/235U, 241Am/235U, and 243Am/235U, a comparison between experiments and Serpent 2 calculations shows an accuracy of about 5%, 15%, and 10%, respectively, together with ENDF/B-VIII.0 and JENDL-5. For the capture reaction rate ratios of 237Np/197Au, Serpent 2 calculations reveal a fairly good accuracy at the thermal neutron spectrum. The total uncertainties of the 237Np/235U, 241Am/235U, and 243Am/235U fission reaction rate ratios by MARBLE with the covariance data of ENDF/B-VIII.0 and JENDL-5 are found to be about 4% at most in all cores, except for about 8% for 243Am/235U with ENDF/B-VIII.0 at the intermediate neutron spectrum.
I. INTRODUCTION
Nuclear transmutation of minor actinides (MAs) and long-lived fission products into stable short-life and long-life radionuclides is considered a promising approach leading to the volume and hazard reduction of radioactive wastes after partitioning high-level radioactive waste generated in light water reactors (LWRs) into some groups and nuclei. In particular, the nuclear transmutation of the 237Np, 241Am, and 243Am of MAs is foreseen as implementable in neutron irradiation systems, including thermal, fast, and spallation neutrons in LWRs, fast reactors, and accelerator-driven systems, respectively.
The feasibility of nuclear transmutation from the fission and capture reactions of MAs has been investigated at experimental facilities, and much experimental data have been archived by the IRPhE (International Handbook of Evaluated Reactor Physics Benchmark Experiments Project)[Citation1] and the ICSBEP (International Criticality Safety Benchmark Evaluation Project)[Citation2] under the leadership of the Organization for Economic Co-operation and Development, Nuclear Energy Agency.
Experimental analyses of MA nuclear transmutation are generally conducted with the use of irradiated MA foil or sample data in a critical core involving MA fission and capture reaction rates. The accuracy of the analyses is evaluated using the reaction rate ratio of the test (MAs) and reference materials of 235U, 239Pu, and 197Au and comparing the reaction rate ratios with experimental and numerical results. Some previous experimental data of MA irradiation in critical assemblies[Citation3–8] are summarized in .
TABLE I Summary of C/E Values of Reaction Rate Ratios in Previous MA Irradiation Experiments
In the range of thermal to fission neutrons for the fission and capture reaction rates of 237Np, 241Am, and 243Am, numerical analyses by Monte Carlo methods[Citation9–11] have been conducted together with numerous nuclear data libraries.[Citation12–19] Other experimental analyses have been conducted for the uncertainty of 237Np inelastic scattering cross sections for criticality at the Los Alamos Criticality Experiments Facility,[Citation20] the validity of cross sections for MAs in the JEFF-3.1 library[Citation21] at the fast reactor Phénix,[Citation22] and the evaluation of MA sample reactivity worth at the Fast Critical Assembly[Citation23] by JENDL-5.[Citation24]
Minor actinide irradiation experiments have been carried out at the neutron spectra of the thermal and intermediate regions obtained using highly enriched uranium (HEU) 93 wt% fuel in the solid-moderated and solid-reflected cores (A cores) at the Kyoto University Critical Assembly (KUCA). Experimental analyses of the fission reaction rate ratios of 237Np, 241Am, and 243Am with 235U, as well as the capture reaction rate ratios of 237Np with 197Au, have been conducted. In previous studies,[Citation7,Citation8] shown in , comparisons between the experimental and numerical reaction rate ratios showed that the relative difference between the calculations and experiments (C/E) was accurate to around 10% in the KUCA-A cores.
Adding a newly intermediate spectrum core to the KUCA-A cores in the present study, sensitivity analyses of the reaction rates and uncertainty quantification of the reaction rate ratios of MAs induced by nuclear data were carried out in numerical analyses using the Serpent 2 code[Citation25] and the MARBLE code system,[Citation26] respectively, together with the nuclear data libraries of ENDF/B-VIII.0 and JENDL-5.
The series of sensitivity and uncertainty analyses of criticality,[Citation27,Citation28] reactivity,[Citation29,Citation30] and reaction rates obtained at KUCA has been notably conducive to the validation of the precision of nuclear cross-section data, applying the data assimilation technique together with experimental data, such as the cross-section adjustment method, to the numerical calculations. To improve the completeness of the integral experiments for nuclear data validation, the uncertainty quantification of MA reaction rate ratios is the focus in the present study, as uncertainty analyses of criticality and reactivity have previously been studied in the KUCA-A cores with the use of the SCALE6.2.1[Citation31] and 6.2.4[Citation32] code systems.
The objective of this study was to examine quantitatively the uncertainty induced by cross sections in the fission and capture reactions using the measured reaction rate ratios of MAs and considering the effects of neutron spectra ranging between the thermal and intermediate regions on the reaction rate ratios of MAs. The details of the core components and experimental settings in the irradiation experiments in the KUCA-A cores are described in Sec. II. The sensitivity and uncertainty quantification of the reaction rate ratios of MAs using Serpent 2 and MARBLE are presented in Secs. III and IV, respectively. Finally, the conclusions of this study are summarized in Sec. V.
II. EXPERIMENTAL SETTINGS
II.A. Core Configurations
A series of critical irradiation experiments was previously carried out in solid-moderated and solid-reflected cores with HEU fuel involving HEU-lead (Pb) (I) and low-enriched uranium (LEU) model cores. The current experiments were additionally conducted in the HEU-Pb (II) core. Detailed information on the cores, irradiation zones, and unit cells in the experiments is listed in . Details of the structures, compositions, and dimensions of the fuel and core components are described in Ref. [Citation33].
TABLE II Unit Cells of the KUCA-A Cores in the MA Irradiation Experiments
II.A.1. HEU-Pb (I) Core
In the HEU-Pb (I) core with the HEU-Pb zone, shown in , the normal fuel rod termed “F” is composed of 60 unit cells sandwiched between upper and lower polyethylene (PE) blocks that are 24.35 in. and 20.75 in. long, respectively, in an aluminum (Al) sheath (2.1 × 2.1 × 60 in.), as shown in . A unit cell is composed of two HEU fuel plates (2 × 2 × 1/16 in. per plate) and one PE plate (2 × 2 × 1/8 in.). Fuel rod “f” is a Pb-zoned special fuel rod with 40 unit cells, two HEU plates, and one Pb plate (2 × 2 × 1/8 in.), as shown in . The “BTB” is a back-to-back special fission chamber for measuring the reaction rates of the test and reference samples, as shown in . The dimensions and structures of the BTB are described in Ref. [Citation7]. In the HEU-Pb (I) core with the HEU-PE zone shown in , numeral “10” corresponds to 10 unit cells of the normal fuel rod shown in .
Fig. 1. Core configuration and components of the HEU-Pb (I) core with the HEU-Pb zone.[Citation7]
![Fig. 1. Core configuration and components of the HEU-Pb (I) core with the HEU-Pb zone.[Citation7]](/cms/asset/288d2b09-c517-48f1-a054-5795158aca7c/unse_a_2380624_f0001a_oc.jpg)
Fig. 2. Core configuration and components of the HEU-Pb (I) core with the HEU-PE zone.[Citation7]
![Fig. 2. Core configuration and components of the HEU-Pb (I) core with the HEU-PE zone.[Citation7]](/cms/asset/9ca362a4-7a0d-453a-b127-d149d6e3910d/unse_a_2380624_f0002_oc.jpg)
II.A.2. HEU-Pb (II) Core
The HEU-Pb (II) core () contains the normal F and special f fuel rods shown in and , respectively. Numeral “54” corresponds to the 54 unit cells of the normal fuel rod shown in , and the “SFC” is a special single-fission chamber for measuring the reaction rates of the test and reference samples, as shown in . The dimensions and structures of the SFC are presented in Ref. [Citation8].
The current MA irradiation experiments of 237Np and 243Am were carried out in the HEU-Pb zone of the HEU-Pb (II) core in terms of the reaction rate analyses of 243Am in the neutron spectrum similar to the HEU-Pb zone of the HEU-Pb (I) core.[Citation8] The neutron spectrum at the detector position in the HEU-Pb zone of the HEU-Pb (II) core was softer than that of the HEU-Pb (I) core due to the difference in the number of special fuel rods loaded at the test zone.
II.A.3. LEU Model Core
In the LEU model core shown in , numeral “16” corresponds to the 16 unit cells of the normal fuel rod shown in . The special fuel rod “LEU” is a LEU model fuel rod with 20 unit cells, four HEU fuel plates, four Al plates (2 × 2 × 1/16 in. per plate), and one natural uranium (NU) plate (2 × 2 × 1/24 in.), as shown in . The average 235U enrichment per unit cell in the LEU model fuel is 16.87 wt%. The 20 unit cells of the LEU model fuel rod are sandwiched between 10 unit cells composed of two 1/16-in. HEU plates and one 1/8-in. PE plate of the normal fuel rod in both the upper and lower sides. Also, the SFC was used to measure the MA reaction rates in the HEU-Pb (II) core.
Fig. 4. Core configuration and components of the LEU model core with the HEU-NU-Al zone.[Citation8]
![Fig. 4. Core configuration and components of the LEU model core with the HEU-NU-Al zone.[Citation8]](/cms/asset/e67d87b0-73bf-454d-a7a1-d97f8933cda4/unse_a_2380624_f0004_oc.jpg)
II.B. Neutron Spectrum
The neutron spectra with a core-wide average of unit cells in the KUCA-A cores were numerically obtained by MCNP6.2[Citation34] with ENDF/B-VIII.0, demonstrating a thermal neutron spectrum in the HEU-PE zone of the HEU-Pb (I) core of around 0.1 eV and an intermediate spectrum in the HEU-Pb zones of the HEU-Pb (I) and (II) cores and the LEU model core ranging between 1 eV and 10 keV, in addition to that of fast neutrons of around a few megaelectron-volts, as shown in .
II.C. Measured Results of Reaction Rate Ratios
The measured results of the reaction rate ratios were acquired through KUCA-A core experiments[Citation7,Citation8] previously carried out in the HEU-Pb (I) and LEU model cores. The experiments were additionally carried out in the HEU-Pb (II) core. The fission reaction rates were evaluated using the ratios of 237Np/235U, 241Am/235U, and 243Am/235U in the HEU-Pb and HEU-PE zones of the HEU-Pb (I) and HEU-Pb (II) cores and the HEU-NU-Al zone of the LEU model core. Capture reaction rates were also evaluated using the ratio of 237Np/197Au in the HEU-Pb and HEU-PE zones of the HEU-Pb (I) core.
The uncertainty of the measured results of the test and reference samples mainly included the statistical errors of the counting rates obtained by the fission and capture events and the effects of the solid angle[Citation35] induced by varying the sample diameter on the detection efficiencies of the BTB and SFC.
III. NUMERICAL SIMULATIONS
III.A. Reaction Rate Ratios by Serpent 2
Numerical analyses of the MA fission and capture reaction rate ratios were performed by the Monte Carlo calculation code Serpent 2 together with the nuclear data libraries ENDF/B-VIII.0 and JENDL-5. The eigenvalue and reaction rate calculations were conducted simultaneously by Serpent 2 with a variation in the number of histories:
For the fission reaction rates, a total of 1.55 × 109 × 5 × 105 histories per cycle, 3.1 × 103 cycles, and a skipped cycle of 1 × 102.
For the capture reaction rates in the HEU-Pb zone of the HEU-Pb (I) core, a total of 1.75 × 109 × 5 × 105 histories per cycle, 3.5 × 103 cycles, and a skipped cycle of 1 × 102.
For the capture reaction rates in the HEU-PE zone of the HEU-PE (I) core, a total of 2.05 × 109 × 5 × 105 histories per cycle, 4.1 × 103 cycles, and a skipped cycle of 1 × 102.
The numerical errors of the eigenvalue, fission, and capture reaction rates were then obtained with a standard deviation within 2 pcm, 0.5%, and 1.5%, respectively. The numerical results of the reaction rate ratios by Serpent 2 were compared with those of the KUCA-A core experiments, as shown in , involving the fission reaction rate ratios of 237Np/235U, 241Am/235U, and 243Am/235U, as well as the capture reaction rate ratios of 237Np/197Au.
TABLE III Comparison Between Experimental and Numerical Results of the Fission and Capture Reaction Rate Ratios
III.B. Accuracy of Serpent 2
In terms of the fission reaction rate ratios of 237Np/235U, a comparison between the experiments and Serpent 2 calculations showed good agreement with a relative difference of around 5% for ENDF/B-VIII.0 and JENDL-5 in the HEU-Pb zones of the HEU-Pb (I) and HEU-Pb (II) cores, as shown in .
The 241Am/235U ratio demonstrated a relative difference of around 5% at the thermal neutron spectrum in the HEU-PE zone of the HEU-Pb (I) core for the two libraries, whereas 241Am/235U in the HEU-Pb zone of the HEU-Pb (I) core demonstrated a discrepancy in the C/E value with a relative difference of around 15%, which is clearly an overestimation by the Serpent 2 calculations. The same 241Am/235U discrepancy was found in an intermediate spectrum, as in the KUCA-B cores shown in , resulting in a meaningful C/E accuracy in Refs. [Citation5,Citation7].
For the ratios of 243Am/235U in the HEU-Pb and HEU-NU-Al zones of the HEU-Pb (II) and LEU model cores, respectively, the Serpent 2 calculations with JENDL-5 showed an accuracy with a relative difference of less than 10% as compared to those with ENDF/B-VIII.0, which demonstrated a relative difference of over 10%.
The capture reaction rate ratio of 237Np/197Au with ENDF/B-VIII.0 and JENDL-5 provided a fairly good agreement at the thermal neutron spectrum of the HEU-PE zone in the HEU-Pb (I) core, although the intermediate region of the HEU-Pb zone in the HEU-Pb (I) core showed a discrepancy in the C/E value of around 10% for the two libraries. It is noteworthy, however, that the experimental error of the HEU-Pb zone was larger than that of the HEU-PE zone.
The Serpent 2 calculations for these series of MA reaction rate ratios provided pertinent experimental results with certain accuracy, indicating a relative difference in the C/E value of around 5% together with ENDF/B-VIII.0 and JENDL-5, except for that of 241Am/235U, which was over 10% for the two libraries.
IV. UNCERTAINTY QUANTIFICATION OF THE REACTION RATE RATIOS BY MARBLE
The sensitivity coefficients of the reaction rates and the uncertainty of the MA reaction rate ratios were acquired by Serpent 2 and MARBLE based on eigenvalue calculations and sensitivity coefficients, respectively, together with the covariance data of ENDF/B-VIII.0 and JENDL-5. MARBLE is a versatile reactor analysis code system, and in this study, a utility in MARBLE was employed to generate the uncertainty of reaction rate ratios together with covariance data based on the sensitivity coefficients obtained by Serpent 2.
The uncertainty of the MA reaction rate ratios was examined in the main reactions of the test MA samples, 237Np, 241Am, and 243Am, and the reference samples, 235U and 197Au, including the fission, capture, elastic scattering, and inelastic scattering reactions. The statistical errors of the sensitivity of the reaction rates and the uncertainty of the reaction rate ratios estimated by Serpent 2 and MARBLE stood at less than 5% and 10%, respectively, for all the reactions of the test and reference samples. Meanwhile, the uncertainties of the other core components were negligibly small in the reaction rate ratios by MARBLE that involved fuel, moderators, reflectors, and assembly sheath. This was attributed to the very few correlations with the MA reaction rates of the test and reference samples.
IV.A. Fission Reaction Rate Ratios
In the fission reaction rate ratios of 237Np/235U, an impact involving 237Np samples in the HEU-Pb zones of the HEU-Pb (I) and (II) cores was about 3.0% and 1.5% in ENDF/B-VIII.0 and JENDL-5, respectively, as shown in . The impact of 241Am in the fission reaction rate ratios of 241Am/235U ranged between 1.7% and 4.3% for the two libraries, as shown in , indicating a difference between the neutron spectra in the HEU-Pb and HEU-PE zones of the HEU-Pb (I) cores, respectively. The 243Am in the fission reaction rate ratios of 243Am/235U demonstrated a different impact of about 8.0% and 2.3% by ENDF/B-VIII.0 and JENDL-5, respectively, as shown in .
TABLE IV Breakdown of Uncertainty of 237Np/235U for Fission Reaction Rates by Serpent 2 in the HEU-Pb Zone of the HEU-Pb (I) and (II) Cores*
TABLE V Breakdown of Uncertainty of 241Am/235U for Fission Reaction Rates by Serpent 2 in the HEU-Pb and HEU-PE Zones of the HEU-Pb (I) Core*
TABLE VI Breakdown of Uncertainty of 243Am/235U for Fission Reaction Rates by Serpent 2 in the HEU-Pb and HEU-NU-Al Zones of the HEU-Pb (II) and LEU Cores, Respectively*
From the results in , , and , the impact of the 235U fission reaction rates was notably smaller, around 0.3%, than that of the MA samples in all the zones and cores. Furthermore, in all the zones and cores, the total uncertainty of the MAs was between 1.5% and 8.0%, and the impact of the other reactions involving the capture, elastic scattering, and inelastic scattering reactions was very small.
IV.B. Capture Reaction Rate Ratios
For the capture reaction rate ratios of 237Np/197Au, the impact of 237Np on the uncertainty by ENDF/B-VIII.0 and JENDL-5 was between 3.3% and 4.3% in the HEU-Pb and HEU-PE zones of the HEU-Pb (I) cores, as shown in , together with a very small impact from the other reactions. Also, total uncertainties were dominant over the 237Np capture reaction rates. Here, the impact of the 197Au capture reactions by JENDL-5 was invisible due to no settings of its covariance data for the 197Au capture cross sections.
TABLE VII Breakdown of Uncertainty of 237Np/197Au for Capture Reaction Rates by Serpent 2 in the HEU-Pb and HEU-PE Zones of the HEU-Pb (I) Core*
IV.C. Correlation Coefficients
The correlation coefficients between the fission and capture reaction ratios by MARBLE were acquired by combining the sensitivity and efficient matrix and the nuclear data covariance matrix in all cores, as shown in . In terms of the fission reaction rate ratios, a strong correlation between identical test samples was observed in similar neutron spectra, while the correlation between the different test samples was small due to the variation between the neutron spectra.
TABLE VIII Correlation Coefficients Between the Fission and Capture Reaction Ratios by Combining the Sensitivity Coefficient Matrix and Nuclear Data Covariance Matrix in the HEU and LEU Model Cores
The correlation relevant to the fission reaction rate ratios was observed in the sensitivities of 237Np, 241Am, and 243Am by comparing the 235U fission reactions shown in , and , respectively. For the capture reactions, the correlation was reasonable in the HEU-Pb (I) core together with the sensitivity in the HEU-Pb and HEU-PE zones, shown in , whereas the correlation with the fission reactions was very minimal, as shown in .
Fig. 6. Regionwise contribution of energy of the 237Np and 235U fission reaction rates by Serpent 2 with ENDF/B-VIII.0 at locations (15, O) and (15, K) in and , respectively.
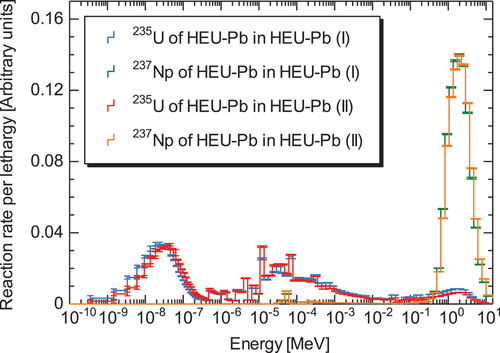
Fig. 7. Regionwise contribution of energy of the 241Am and 235U fission reaction rates by Serpent 2 with ENDF/B-VIII.0 at locations (15, O) and (15, K) in and , respectively.
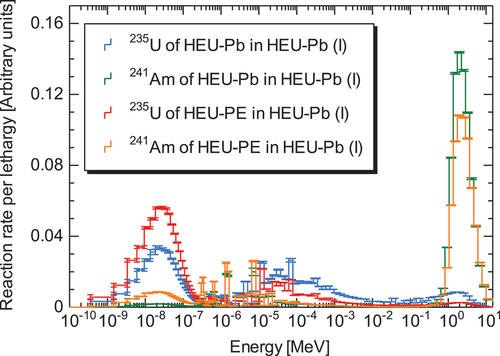
IV.D. Discussion
The 241Am/235U fission reaction rate ratio revealed a discrepancy of around 15% in the C/E in the KUCA-A core analyses, as shown in Sec. III.B. The experimental uncertainty of the 241Am fission reaction rates was investigated with the statistical errors of measured counting rates of the fission events and geometrical effects of the BTB and SFC. Also, the numerical results of the 241Am fission reaction rates were acquired based on an adequate standard deviation within 0.5% and a total uncertainty induced by the 235U and 241Am fission cross sections of about 4% at most.
From the experimental and numerical deviations, the discrepancy of the 241Am/235U fission reaction rate ratio for ENDF/B-VIII.0 and JENDL-5 in the KUCA cores remains a notable technical issue to be resolved in the analyses of the MA reaction rates, including unrecognized sources of uncertainties.
In a series of uncertainty analyses of the fission and capture reaction rate ratios, as shown in through , the difference between ENDF/B-VIII.0 and JENDL-5 was attributable to that of the covariance data of the nuclear cross sections in the two libraries. The total uncertainties of 237Np/235U, 241Am/235U, and 243Am/235U for fission and 237Np/197Au for the capture reactions ( through ) in the KUCA-A cores with the use of HEU fuel were negligibly small due to the variation in the neutron spectra and core components compared with the uncertainties of criticality[Citation25,Citation26] and reactivity[Citation27,Citation28] discussed in previous studies. Also, since the correlation between the target and other reactions was quite small, no effective values of uncertainties were found in various reactions, as shown in through .
V. CONCLUDING REMARKS
Uncertainty quantification of the MA fission and capture reaction rate ratios was conducted using Serpent 2 and MARBLE together with ENDF/B-VIII.0 and JENDL-5 and the experimental data obtained from the KUCA-A cores. The fission and capture reaction rate ratios of the test samples of 237Np, 241Am, and 243Am were evaluated using reference samples of 235U and 197Au.
In terms of the fission reaction rate ratios of 237Np/235U, 241Am/235U, and 243Am/235U, a comparison between the experiments and Serpent 2 calculations showed an accuracy of about 5%, 15%, and 10%, respectively, with ENDF/B-VIII.0 and JENDL-5. For the capture reaction rate ratios of 237Np/197Au, Serpent 2 calculations revealed a fairly good accuracy at the thermal neutron spectrum.
The uncertainty of the MA fission and capture reaction rate ratios was found to be around 3%, except for that of 243Am/235U, which was about 8%. Also, the total uncertainties of the MA fission reaction rate ratios with ENDF/B-VIII.0 and JENDL-5 were small, about 4% at most in the all cores, except for the fission reaction rate ratio of 243Am/235U with ENDF/B-VIII.0 at the intermediate neutron spectrum, which was about 8%. For the MA reaction rate analyses in the KUCA-A cores, the discrepancy in the fission reaction rate ratio of 241Am/235U remains a notable technical issue through a series of experimental analyses of the MA reaction rates.
Acknowledgments
The authors are grateful to all the technical staff at the KUCA for their assistance during the experiments.
Disclosure Statement
No potential conflict of interest was reported by the authors.
Additional information
Funding
References
- International Handbook of Evaluated Reactor Physics Benchmark Experiments (IRPhE) Project, NEA/NSC/DOC(2006)1, Organization for Economic Co-operation and Development, Nuclear Energy Agency (2018).
- International Criticality Safety Benchmark Evaluation Project (ICSBEP) Handbook, NEA/NSC/DOC(95)03, Organization for Economic Co-operation and Development, Nuclear Energy Agency (2018).
- T. IWASAKI, H. UNESAKI, and D. FUJIWARA, “Measurement and Analysis of Capture Reaction Rate of 237Np in Various Thermal Fields by Critical Assembly and Heavy Water Thermal Neutron Facility of Kyoto University,” Nucl. Sci. Eng., 136, 321 (2000); https://doi.org/10.13182/NSE00-A2162.
- H. UNESAKI et al., “Measurement of 237Np Fission Rate Ratio Relative to 235U Fission Rate in Cores with Various Thermal Neutron Spectrum at the Kyoto University Critical Assembly,” J. Nucl. Sci. Technol., 37, 627 (2000).
- H. UNESAKI et al., “Measurement and Analysis of 241Am Fission Rate Ratio Relative to 235U Fission Rate in Thermal Neutron Systems using Kyoto University Critical Assembly,” J. Nucl. Sci. Technol., 38, 600 (2001).
- M. FUKUSHIMA, K. TSUJIMOTO, and S. OKAJIMA, “Analyses with Latest Major Nuclear Data Libraries of the Fission Rate Ratios for Several TRU Nuclides in the FCA-IX Experiments,” J. Nucl. Sci. Technol., 54, 795 (2017).
- C. H. PYEON et al., “Integral Experiments on Critical Irradiation of 237Np and 241Am Foils at Kyoto University Critical Assembly,” Nucl. Sci. Eng., 193, 1023 (2019); https://doi.org/10.1080/00295639.2019.1603014.
- C. H. PYEON, A. OIZUMI, and M. FUKUSHIMA, “Experimental Analyses of 243Am and 235U Fission Reaction Rates at Kyoto University Critical Assembly,” Nucl. Sci. Eng., 195, 1144 (2021); https://doi.org/10.1080/00295639.2021.1932220.
- T. MORI and M. NAKAGAWA, “MVP/GMVP: General Purpose Monte Carlo Codes for Neutron and Photon Transport Calculations Based on Continuous Energy and Multigroup Methods,” JAERI-Data/Code 94-007, Japan Atomic Energy Research Institute (1994) (in Japanese).
- Y. NAGAYA et al., “MVP/GMVP II: General Purpose Monte Carlo Codes for Neutron and Photon Transport Calculations based on Continuous Energy and Multigroup Methods,” JAERI 1348, Japan Atomic Energy Research Institute (2005).
- J. T. GOORLEY et al., “Initial MCNP6 Release Overview—MCNP6 Version 1.0,” LA-UR-13-22934, Los Alamos National Laboratory (2013).
- T. NAKAGAWA et al., “Japanese Evaluated Nuclear Data Library Version 3 Revision-2: JENDL-3.2,” J. Nucl. Sci. Technol., 32, 1259 (1995).
- “ENDF-201, ENDF/B-VI Summary Documentation,” BNL-NCS-17541, 4th ed, P. F. ROSE, Ed. Brookhaven National Laboratory (1991).
- “JEF-2.2 Evaluated Data Library,” Organisation for Economic Co-operation and Development, Nuclear Energy Agency (April 2000); http://www.oecd-nea.org/dbforms/data/eva/evatapes/jef_22/ ( current as of Apr. 2024).
- K. SHIBATA et al., “JENDL-4.0: A New Library for Nuclear Science and Engineering,” J. Nucl. Sci. Technol., 48, 1 (2011).
- M. B. CHADWICK et al., “ENDF/VII.1 Nuclear Data for Science and Technology: Cross Sections, Covariances, Fission Product Yields and Decay Data,” Nucl. Data Sheet, 112, 2887 (2011).
- “JEFF-3.2 Evaluated Nuclear Data Library—Neutron Data,” Organisation for Economic Co-operation and Development, Nuclear Energy Agency (May 2014); http://www.oecd-nea.org/dbforms/data/eva/evatapes/jeff_32/ ( current as of Apr. 2024).
- “JEFF-3.3: The Joint Evaluated Fission and Fusion File (JEFF),” Organisation for Economic Co-operation and Development, Nuclear Energy Agency (November 2017); http://www.oecd-nea.org/dbdata/jeff/jeff33/ ( current as of Apr. 2024).
- D. A. BROWN et al., “ENDF/B-VIII.0: The 8th Major Release of the Nuclear Reaction Data Library with CIELO-Project Cross Sections, New Standards and Thermal Scattering Data,” Nucl. Data Sheets, 148, 1 (2018).
- D. LOAIZA et al., “Results and Analysis of the Spherical 237Np Critical Experiment Surrounded by Highly Enriched Uranium Hemispherical Shells,” Nucl. Sci. Eng., 152, 65 (2006); https://doi.org/10.13182/NSE06-A2564.
- “JEFF-3.1 Evaluated Data Library,” Organisation for Economic Co-operation and Development, Nuclear Energy Agency (May 2005); https://www.oecd-nea.org/dbforms/data/eva/evatapes/jeff_31/ ( current as of Apr. 2024).
- J. TOMMASI and G. NOGUÈRE, “Analysis of the PROFIL and PROFIL-2 Sample Irradiation Experiments in Phénix for JEFF-3.1 Nuclear Data Validation,” Nucl. Sci. Eng., 160, 232 (2008); https://doi.org/10.13182/NSE160-232.
- M. FUKUSHIMA, S. OKAJIMA, and T. MUKAIYAMA, “TRU Oxide Sample Reactivity Worths Measured in the FCA-IX Assemblies with Systematically Changed Neutron Energy Spectra,” J. Nucl. Sci. Technol., 61, 478 (2024).
- O. IWAMOTO et al., “Japanese Evaluated Nuclear Data Library Version 5: JENDL-5,” J. Nucl. Sci. Technol., 60, 1 (2023).
- J. LEPPÄNEN, M. PUSA, and E. FRIDMAN, “Overview of Methodology for Spatial Homogenization in the Serpent 2 Monte Carlo Code,” Ann. Nucl. Energy, 96, 126 (2016).
- K. YOKOYAMA et al., “Development of Comprehensive and Versatile Framework for Reactor Analysis, MARBLE,” Ann. Nucl. Energy, 66, 51 (2014).
- M. YAMANAKA and C. H. PYEON, “Benchmarks of Criticality in Solid-Moderated and Solid-Reflected Core at Kyoto University Critical Assembly,” Nucl. Sci. Eng., 193, 404 (2019); https://doi.org/10.1080/00295639.2018.1525978.
- C. H. PYEON and K. MORIOKA, “Nuclear Data-Induced Uncertainty of Criticality in Solid-Moderated and Solid-Reflected Cores with Highly Enriched Uranium Fuel at Kyoto University Critical Assembly,” Nucl. Sci. Eng., 196, 1147 (2022); https://doi.org/10.1080/00295639.2022.2070385.
- C. H. PYEON, M. YAMANAKA, and M. FUKUSHIMA, “Uncertainty Quantification of Lead and Bismuth Sample Reactivity Worth at Kyoto University Critical Assembly,” Nucl. Sci. Eng., 195, 877 (2021); https://doi.org/10.1080/00295639.2020.1870861.
- C. H. PYEON et al., “Void Reactivity in Lead and Bismuth Sample Reactivity Experiments at Kyoto University Critical Assembly,” Nucl. Sci. Eng., 197, 2902 (2023); https://doi.org/10.1080/00295639.2023.2172311.
- B. T. REARDEN and M. A. JESSEE, “SCALE Code System,” ORNL/TM-2005/39, Version 6.2.1, Oak Ridge National Laboratory (2016).
- “SCALE Code System,” ORNL/TM-2005/39, Version 6.2.4, W. A. WIESELQUIST, R. A. LEFEBVRE, and M. A. JESSEE, Eds. Oak Ridge National Laboratory (2020).
- Accelerator-Driven System at Kyoto University Critical Assembly, C. H. PYEON, Ed., Springer, Singapore (2021); https://link.springer.com/book/10.1007/978-981-16-0344-0 ( current as of Apr. 2024).
- C. J. WERNER et al., “MCNP6.2 Release Notes,” LA-UR-18-20808, Los Alamos National Laboratory (2018).
- L. MOENS et al., “Calculation of the Absolute Peak Efficiency of Gamma-Ray Detectors for Different Counting Geometries,” Nucl. Instrum. Methods, 187, 451 (1981).