ABSTRACT
Seaweed aquaculture is a relatively young industry in the United States compared to Asian countries. Early attempts at seaweed aquaculture in California, Washington State, New York and the Gulf of Maine in the 1980s and 1990s did not result in commercial production but provided important lessons. Since 2010, commercial cultivation of kelp (Saccharina latissima, Laminaria digitata, and Alaria esculenta) and other seaweeds (Palmaria palmata and Porphyra umbilicalis) began in the Gulf of Maine and Long Island Sound. Seaweed aquaculture is now a fast-growing maritime industry, especially in New England. If seaweed aquaculture is to maintain its momentum, it is important to (1) emphasise the environmental benefits; (2) domesticate a variety of local species; and (3) diversify seaweed products for food, animal feed, phycocolloids, cosmeceuticals, nutraceuticals, and ultimately biofuels if it becomes economically viable due to the cost of production. The exclusive economic zone (EEZ) of the United States offers opportunities for expansion of seaweed aquaculture in an area greater than the entire land mass of the United States and with limited user conflicts. This study reviews the past and current status of seaweed aquaculture in the United States and discusses potential opportunities and challenges of open-water seaweed aquaculture.
INTRODUCTION
Seaweeds have been consumed by humans around the world for centuries, possibly millennia. A recent study suggested that consuming seaweeds might have been important for human brain growth of early Homo ancestors who lived along coasts (Cornish et al. Citation2017). Various seaweeds have also been an important part of Asian cuisine. However, in Western countries, direct consumption of seaweeds is restricted to a few scattered coastal areas (Hunter Citation1975; Mouritsen et al. Citation2013). In the United States, harvest and consumption of Palmaria (dulse) have a long tradition in the state of Maine, USA and in the Canadian Maritime Provinces, probably for more than a century (Mouritsen et al. Citation2013). Ancient Hawaiians also ate seaweeds (called limu) as a regular part of their daily diet. Reed (Citation1907) reported that over 70 seaweed species were consumed by Hawaiians. Hawaiians ‘cultivated’ natural populations of seaweeds growing in their coastal ponds in order to increase their harvest and the quality of limu by weeding out undesirable seaweeds. Certain favourite limu varieties were transplanted from one island to another in Hawaii (Reed Citation1907). Hawaiians still consume limu (principally Gracilaria spp.), although most seaweeds are wild harvested. Beginning in the 1970s, overharvesting severely depleted natural stocks, resulting in a conservation law which limited the harvest of wild seaweeds in Hawaii (Glenn et al. Citation1998; Nagler et al. Citation2003). Alaskan natives also wild harvest and use selected seaweeds on a subsistence basis (Dombrowski Citation2007). For example, the subsistence harvest of ‘black seaweed’, a species of Pyropia, was estimated to be more than 150 metric tons (fresh weight, FW) in Sitka in 2013 (ADFG Citation2013). Other wild-harvested seaweeds in Alaska include the bull kelp (Nereocystis), giant kelp (Macrocystis), and red ribbon seaweed (Palmaria).
Figs 1–3. Pyropia yezoensis farm at Eastport, Maine. Photos by I. Levine.
Fig. 1. Ikada nursery system. Fig. 2. Production farm. Fig. 3. Aerial view of the Pyropia farm.
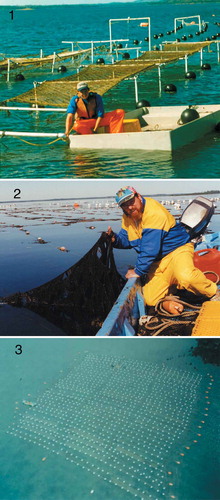
Global seaweed aquaculture production is over 30 million metric tons (FW) and, in 2016, had an annual value of $11.7 billion (Food and Agriculture Organization [FAO] 2018). Over 99% of the production (i.e. over 29.9 million metric tons) is grown by various methods in Asia. Typically, only five major countries (China, Indonesia, the Philippines, Korea and Japan) produce over 97% of seaweed globally (FAO 2018). Seaweed aquaculture is a relatively new industry in the United States, where commercial cultivation of the brown seaweed Saccharina latissima (Linnaeus) C.E.Lane, C.Mayes, Druehl & G.W.Saunders was initiated during the last decade (Flavin et al. Citation2013; Kim et al. Citation2015; Kraemer et al. Citation2014; Redmond et al. Citation2014a; Rose et al. Citation2015). Currently, seaweed aquaculture is one of the fastest growing maritime industries in the coastal waters of New England, USA. Demand by American markets is rapidly increasing due to (1) consumer desire for new protein sources and healthy food supplements; (2) the food industry’s interest in sustainable textural additives including phycocolloids; and (3) the need for enhanced food security. Domestic production is well over 1000 metric tons (FW), but this is below the threshold to be included in FAO statistics (FAO 2016). US Department of Agriculture’s National Agricultural Statistics Service does not include seaweed aquaculture production in its statistical data, although the wild harvest of seaweeds was recorded at about 6500 metric tons with a commercial value of more than US$1 million in 2015. At the same time, the import of seaweed raw materials was more than 10,000 metric tons (over US$73 million; National Marine Fisheries Service Office of Science and Technology Citation2016), which was used mostly for food and colloids. The present study reviews the past and current status of open-water seaweed aquaculture in the United States and discusses potential opportunities and challenges.
PAST: 1970s–1990s
Marine Biomass Program in California and New York
The US Marine Biomass Program was a research and development programme to develop integrated processes for producing and harvesting seaweeds of interest in the ocean and converting their biomass to methane. The programme began in the early 1970s in California (Flowers & Bird Citation1984; Neushul Citation1980, Citation1986; North et al. Citation1982; Tompkins Citation1982) as a reaction to the energy crisis caused by an oil embargo imposed by members of the Organization of Arab Petroleum Exporting Countries. The programme in California was initiated by H. Wilcox of the US Naval Undersea Warfare Center. It was supported by the US National Science Foundation and the US Navy from 1972 to 1973. Wilcox continued his work on the Marine Biomass Program with the support of the US Energy Research and Development Agency and American Gas Association from 1974 to 1975 (Neushul Citation1981, Citation1986). From 1976, General Electric led this programme with support from the American Gas Association and US Department of Energy’s Solar Energy Research Institute. Support for the programme was then transferred to the Gas Research Institute (GRI; Chicago, Illinois USA). General Electric directed the programme until 1984. GRI discontinued the programme in December 1986 (Neushul Citation1986).
Wheeler J. North and Michael Neushul were involved in the GRI programme in California. North (California Institute of Technology) conducted the development of an offshore kelp farm, using Macrocystis pyrifera (Linnaeus) C.Agardh as the key crop, near San Clemente Island, California, in 1972 and 1973. Macrocystis was transplanted onto a 150 m × 180 m grid made of ropes, placed 20 m under the surface. However, this initial attempt at seaweed farming failed because the plants and the ropes became tangled, resulting in the complete loss of the kelp and farming structure. North made another attempt with some modifications of the grid using a nearshore site off Corona del Mar, California. Deep water was brought up to nourish the kelp, using airlift bubbling, and the Macrocystis grew well (North Citation1976, Citation1987; North et al. Citation1982; Wheeler et al. Citation1981). After these attempts, North conducted offshore cultivation of Macrocystis again with A. N. Tompkins (General Electric) 6 miles offshore from Laguna Beach. To counter nutrient depletion in this offshore environment, they also brought deep-sea water to the surface and hung a curtain around the farm to retain the nutrients long enough for uptake by the kelps. They transplanted 103 giant kelp plants on a modified module, but a storm event tore off the curtain and damaged the cultivation structure (North Citation1987; North et al. Citation1982). The final attempt by North, with support from General Electric, was in 1982 at Catalina Island, California. For this, General Electric constructed and installed a large ring structure, which looked like a floating doughnut, 15 m in diameter, supporting a plastic bag. This system was a half sphere in appearance, so it was called a hemi-dome. Macrocystis grew well in this system, showing potential for offshore kelp farming. However, in June 1982, the biggest El Niño of the century hit the area, dramatically reducing kelp growth (Neushul Citation1986; North Citation1994).
Neushul (University of California at Santa Barbara) also received programme support from 1980 to 1986. In 1981, Neushul cultivated Macrocystis near Goleta, California, and provided biomass yield information for Macrocystis (Neushul Citation1986; Neushul & Harger Citation1985) that North had failed to produce in his offshore work. Whereas North created a completely novel cultivation system, without much consideration of the natural habitats of the kelp, Neushul tried to model his farm system on a natural Macrocystis bed. Seven hundred twenty-two individuals were transplanted from nearby kelp beds using a gravel-filled bag planting method. He obtained an average annual highest growth rate of 7 g m−2 d−1 (Harger & Neushul Citation1983; Neushul & Harger Citation1985). The kelp was continuously cultured through the following year at the nearshore farm site, and 11 metric tons (FW) of standing crop was harvested in 12 months (Neushul Citation1986; Neushul & Harger Citation1985). A novel planting technology using fertiliser was applied to the nutrient-limited waters of Santa Barbara. Genetic studies of Macrocystis were conducted. Neushul developed about 800 strains of M. pyrifera. Clones were crossed and morphologically distinctive sporophytes were produced (Harger & Neushul Citation1983; Lewis et al. Citation1986; Neushul Citation1986).
The Marine Biomass Program in New York, USA, was initiated in 1980 with support from the GRI, New York State Energy Research and Development Authority, New York Gas Group and the New York Sea Grant Institute (Brinkhuis & Hanisak 1981; Brinkhuis et al. Citation1983, Citation1984a, Citation1984b, Citation1987; Squires & McKay Citation1983). After a thorough initial screening process to select seaweed species suitable for methane production, ‘Laminaria saccharina’ [now Saccharina latissima (Linnaeus) C.E.Lane, C.Mayes, Druehl & G.W.Saunders] was selected as the prime candidate for the Marine Biomass Program in New York (Brinkhuis et al. Citation1987). After numerous laboratory and tank experiments were conducted to determine optimal growth conditions for this alga (Brinkhuis et al. Citation1984a), S. latissima seed-string was outplanted in an open-water farm using two different cultivation technologies: (1) the Chinese style of attaching individual plants by entwining stipes/holdfast with the line and (2) the Japanese method of inserting segments of seed-string into the culture rope. The kelps were cultivated at different depths at Crane Neck, Long Island, New York (Brinkhuis et al. Citation1983, Citation1984a, Citation1987). In 1983, Brinkhuis and associates also developed a new seaweed farm design, the Biological Engineering Experimental Farm, and crossed gametophytes of Saccharina from different populations. Brinkhuis and associates outplanted these crosses at their open-water farm site (Brinkhuis et al. Citation1984b). In late 1980, additional inter- and intraspecific crosses of North Atlantic kelp species were made by another research group led by Yarish and colleagues, with support from the Connecticut Sea Grant College Program to develop suitable strains for kelp aquaculture in Long Island Sound (Egan et al. Citation1989, Citation1990; Egan & Yarish Citation1990; Yarish & Egan Citation1987, Citation1989; Yarish et al. Citation1990a, Citation1990b).
Although these early attempts at kelp aquaculture in California, Connecticut and New York showed that nearshore farming of Macrocystis and Saccharina was viable, the Marine Biomass Program was discontinued in 1986. However, the Connecticut Sea Grant Program continued to support kelp research until 1991 (Egan et al. Citation1990). One of the main reasons for ending the New York research programme was that geologists and oil and gas specialists estimated that there would be no shortage of oil and natural gas for decades to come. In addition, in 1986, the US Department of Energy switched their research focus to microalgal energy production (Neushul Citation1986).
There were important findings from these earlier studies which were pertinent for subsequent kelp aquaculture attempts in the 2010s (see below). The reproductive peaks of Saccharina in Long Island Sound (i.e. late spring and fall/early winter) and the optimal conditions for gametophyte culture for maturation and reproduction were determined through these studies (Brinkhuis et al. Citation1987; Egan et al. Citation1989; Yarish et al. Citation1990a). This information was critical for nursery cultivation technology which was developed later in the 2010s by the Yarish–UConn Labs (Kim et al. Citation2015; Redmond et al. Citation2014a). Understanding the seasonal variation of kelp sporophytes in Long Island Sound (Egan et al. Citation1989; Egan & Yarish Citation1990; Yarish et al. Citation1990b) would become important information for the advance of open-water cultivation technologies not only in Long Island Sound but throughout New England, Alaska, Washington, and California.
Challenges and Lessons Learned
The Marine Biomass Program provided some US$20 million over a 12-year period (Neushul Citation1986) before the programme was discontinued. The biggest drawback of the programme was that kelp farming could not compete on an economic basis with fossil fuel production. In addition to the lack of economic viability, there was criticism from the public and the media. Communications with, and support from, the public are key to receiving a social licence for the success of seaweed aquaculture in the United States. The results of these earlier attempts also underlined the importance of cultivation technologies for both nearshore and offshore waters (e.g. development of new cultivars, improvement of nursery and seeding technology, development of farm gear for offshore environments). Finally, diversification of commercial products derived from cultivated biomass of various seaweeds is critical because the price for one product (e.g. bioenergy) must be low enough to compete with fossil fuel production. The work of North, Neushul, Brinkhuis and others was foundational because they documented their attempts at seaweed aquaculture for the future success of applied seaweed aquaculture research (Brinkhuis et al. Citation1987).
Pyropia (Porphyra) farming in Washington state
Another attempt at open-water seaweed aquaculture in the United States occurred in Puget Sound, Washington state in the 1980s. The initiation of cultivating Pyropia yezoensis (Ueda) M.S. Hwang & H.G. Choi (formerly ‘Porphyra yezoensis’) was led by the Washington State Department of Natural Resources (T.F. Mumford) and the University of Washington (R. Waaland and J.E. Merrill). These scientists worked with Japanese experts (A. Miura and the Zen-nori Cooperative) to obtain technologies from Japan, who provided seed nets of Pyropia to growers in Puget Sound. Two reasons were identified for the selection of Pyropia for aquaculture (Mumford Citation1990). First, surface seawater temperature along Washington coasts was found to be ideal for year-round cultivation of Pyropia; whereas, the major Asian countries for Pyropia production (e.g. China, Korea and Japan) could cultivate only during winter. In addition, in the United States in the 1980s there was a boom of sushi restaurants and a high demand for nori sheets which are manufactured from Pyropia. The strains of Pyropia used were imported from Japan [six strains of Pyropia yezoensis and one strain of Py. tenera (Kjellman) N.Kikuchi, M.Miyata, M.S.Hwang & H.G.Choi]. Additionally, local strains of Pyropia were developed and cultivated [Pyropia abbottiae (V.Krishnamurthy) S.C.Lindstrom, Py. torta (V.Krishnamurthy) S.C.Lindstrom, Py. nereocystis (C.L.Anderson) S.C.Lindstrom and Py. pseudolanceolata (V.Krishnamurthy) S.C.Lindstrom]. The decision to import Japanese strains of Pyropia was based upon literature which indicated that the conchocelis of Japanese strains would not reproduce in the cold water and short daylengths prevalent in Washington state (Mumford & Hansen Citation1987). In addition, the aquaculture trade of spat-bearing shells from Japan to the United States for oyster farms might have already relocated the Japanese conchocelis to Washington state. However, no Japanese Pyropia species used in aquaculture have been recorded in either Washington or any neighbouring US states or Canada (Guiry & Guiry Citation2012). However, they have appeared on the east coast of the United States (Neefus et al. Citation2008; details below).
Pyropia was cultivated in pilot-scale seaweed farms during the 1982 to 1983 winter growing season using modifications of Japanese technologies for nursery and open-water farming – that is, raft-oriented style – utilising floating seeding rafts, nursery sets and production frames (Merrill Citation1981; Mumford Citation1987). Only three of seven Japanese strains grew successfully; whereas, all four Washington strains were successful (Mumford Citation1990). Subsequently, a number of private-sector companies obtained permits for seaweed farming and collaborated in Pyropia aquaculture. Based on trials at locations in Washington state, the San Juan Islands appeared to be best suited for Pyropia farming due to a favourable mixture of oceanic waters and freshwater run-off, as well as high ambient nutrient levels. However, Pyropia did not grow well in lower or central Puget Sound (Mumford Citation1990). During this period, an organisation for Pyropia farming, the Pacific Northwest Nori Growers Association, was formed. Other new businesses such as American Sea Vegetable, New Channel Nori and Pacific Link were founded in the state (Mumford Citation1990). However, Pyropia farming in Washington was discontinued due to the issues listed below, and none of those businesses associated with Pyropia currently exist.
Challenges and Lessons Learned
Although aquaculture of Pyropia in Washington state had many advantages, including technological support from a Washington state agency and the University of Washington (a public university) and demand for production and interest by the industry, prevailing political and social resistance resulted in a negative outcome. In Washington state, at least three permits were needed for seaweed aquaculture, requiring time, money and effort from growers (Mumford Citation1987). Washington was not the only state with this permitting issue. The legal regime governing coastal waters in the United States gives jurisdiction to individual states. Aquaculture regulations vary from state to state and sometimes even from coastal town to town within a single state, which can complicate the application process (Duff et al. Citation2003; Getchis & Rose Citation2011; National Research Council Citation2010). At least 120 federal laws were identified which affected aquaculture, either directly (50 laws) or indirectly (70 laws), and more than 1200 state statutes regulated aquaculture in 32 states (Aspen Research and Information Center Citation1981). Regulatory complexity was further increased when towns or counties had jurisdiction over local waters. To site and operate, aquaculture enterprises might require more than 30 permits under the purview of local, state and federal agencies, and, permitting could take years to process (Duff et al. Citation2003; Getchis & Rose Citation2011; Langan et al. Citation2006). In addition, open-water aquaculture activities elicited considerable opposition from shoreline property owners due to the phenomenon known as NIMBY (Not In My Back Yard). NIMBY increases the complexity of permitting processes even more by introducing politics into the process (Hansen Citation1989). Opposition by stakeholder groups eventually terminated Pyropia aquaculture in coastal waters of Washington state (Hansen Citation1989). In the1980s, the US economy was booming and per capita gross domestic product increased by nearly 23% (Schaller Citation1992). During this economic boom, there was little urgency to develop coastal environments for seaweed aquaculture.
In 1994, Washington state announced a moratorium on all commercial seaweed harvesting from natural populations (Waaland Citation2004). Seaweed aquaculture was considered to be a viable strategy to support the industry but had been limited to land-based systems (Hall Citation2011; Waaland Citation2004). Open-water seaweed aquaculture using Saccharina latissima has recently resumed in Puget Sound. However, the main purpose of this project has been to use the nutrient extractive properties of kelp cultivation to mitigate environmental issues of eutrophication and ocean acidification (Puget Sound Restoration Fund Citation2019).
Pyropia farming in Maine
When attempts to farm Pyropia failed in Washington state, the cultivars (i.e. two Japanese strains of Py. yezoensis, U51 and H25) and locally developed cultivation technologies were transferred to Coastal Plantations International of Maine (later incorporated into PhycoGen, Inc., Portland, Maine, USA). Unlike Washington state, seaweed farming in Maine was welcomed by both regulators and the public. There was an expectation that seaweed aquaculture could be an additional source of income for impoverished coastal communities in Maine. Additionally, Mainers had eaten seaweeds for more than a century. Harvest of wild seaweed there was already an important industry (Chopin et al. Citation1998; Levine Citation1997; Levin & Cheney Citation1998; Yarish et al. Citation1998).
During the 1994–1995 growing season, Coastal Plantations International farmed Pyropia yezoensis in Cobscook Bay, Eastport, Maine, using Japanese cultivation technologies. They used both pole and floating culture systems in order to determine the most appropriate farming technique for the Gulf of Maine region (–). The first attempt was unsuccessful because Cobscook Bay was oligotrophic, and insufficient nutrients were available for growth of Pyropia. In 1996, this company, in collaboration with the University of Connecticut (C. Yarish) and University of New Brunswick (T. Chopin), moved their ‘seeded’ nori nets to an area adjacent to an Atlantic salmon (Salmo salar Linnaeus) farm at Deep Cove, Eastport, Maine (Connors Aquaculture Inc.; McVey et al. Citation2002; Yarish et al. Citation1997, Citation1998). This was the first open-water, integrated multitrophic aquaculture practise in the United States. Pyropia received a high concentration of nutrients from the farmed fish effluents and grew better than clonal strains of Pyropia at the oligotrophic site in Cobscook Bay (Chopin & Yarish Citation1998; Chopin et al. Citation1998, Citation1999). However, PhycoGen went bankrupt due to a downturn in the investment environment. No further integrated seaweed aquaculture was attempted until recently in Maine (Levine Citation2006).
Following this attempt in Maine, cultivar development and strain selection were conducted using native Pyropia linearis (Ueda) N.Kikuchi, M.Miyata, M.S.Hwang & H.G.Choi, Py. yezoensis, Py. leucosticta (Thuret) Neefus & J.Brodie, Porphyra purpurea (Roth) C.Agardh, and Wildemania amplissima (Kjellman) Foslie. Optimal environmental conditions for eco-physiological activities, including growth, nutrient uptake, pigment content, and photosynthesis, were determined for these target species (Carmona et al. Citation2006; Day Citation2003; Kim et al. Citation2007, Citation2008; Kraemer & Yarish Citation1999; Kraemer et al. Citation2004; Yarish et al. Citation1998, Citation1999). Critical controlling factors for the life cycles of the putative crop species were studied. For example, He & Yarish (Citation2006) cultured the conchocelis of Py. yezoensis (misidentified as Py. leucosticta in their paper at the time) not only by using shells (as per the traditional cultivation technology in Asia) but also in a novel, ‘free-living’ suspension in 13-litre Pyrex jar culture vessels. They determined the conditions for vegetative propagation of ‘free-living’ conchocelis filaments, which led to the continuous maintenance of stock cultures (i.e. conditions of 15 °C, 40 μmol m−2 s−1 and 16:8 light:dark). To induce conchosporangia, temperature was increased to 20 °C, photoperiod decreased to 8:16 light:dark, and photon fluence rate was maintained as low as 40 μmol m−2 s−1. Conchosporangial filaments were vegetatively propagated and maintained under these conditions for up to 24 weeks. Conchospores were released after 10 days by decreasing the temperature to 15 °C, increasing the photon fluence rate to 60–100 μmol m−2 s−1, and increasing the photoperiod to 12:12 light:dark. He & Yarish (Citation2006) estimated that, at their peak release, 1 g (DW) of free conchosporangia could release about 20 million conchospores. These conchospores were seeded onto 16 standard Pyropia nets (1.5 × 18 m) and eight small nets (2.0 × 2.5 m). Four seeded standard Pyropia nets were then transferred to a site in western Long Island Sound (Bridgeport, Connecticut) for nursery culture. After 43 days, blades grew up to 1.5 cm in length, thereby establishing the effectiveness of ‘free-living’ conchocelis suspension cultures as an alternative technology for Pyropia aquaculture. However, for reasons unknown, the Pyropia did not grow after the nets were moved to a nearshore seaweed farm, which was also in close proximity to a wastewater treatment plant outfall in western Long Island Sound (Fairfield, Connecticut; He & Yarish Citation2006).
Challenges and Lessons Learned
Obtaining permits for seaweed aquaculture in Maine was, and continues to be, not as difficult as in other states. Maine had a programme that granted limited production aquaculture licence permits to enable prospective aquaculturists to develop their skill set for aquaculture of a particular species (see Maine Department of Marine Resources Citation2019). Therefore, permits might not be the biggest hurdle for the development of seaweed aquaculture in Maine. A major problem with farming Pyropia in Maine was the lack of knowledge related to the ecophysiology of the nonnative Japanese strain and lack of understanding of the oceanography of the Gulf of Maine itself. The Japanese strain of Py. yezoensis is a warm–temperate species. This strain had difficulty adapting to prevailing low temperatures, the relatively short growing season and the nutrient-replete waters of the Gulf of Maine (Cheney et al. Citation1998; Levine Citation1998, Citation2006; Watson et al. Citation1999).
One must use extra caution before a nonindigenous species is imported for aquaculture (Minchin Citation2007). Aquaculture permits for cultivating Japanese strains of nori were issued based on evidence that endemic environmental conditions (i.e. sea surface temperature and photoperiod) in the Gulf of Maine were not favourable for the sexual reproduction of these strains (Levine et al. Citation2001; Neefus et al. Citation2008; Watson et al. Citation1999; West et al. Citation2005). In fact, the Japanese commercial cultivar of Py. yezoensis was not found in the Gulf of Maine. However, interestingly, this strain was found in Long Island Sound, over 400 km south of the Gulf of Maine, where, as far as can be determined, Japanese cultivars had never been cultivated (Neefus et al. Citation2008; Niwa et al. Citation2005; West et al. Citation2005). Neefus et al. (Citation2008) suggested that there had been a single introduction of this strain of Pyropia to Long Island Sound, but the vector for this introduction remains unknown. These results suggested that investment in cultivar development is critical for local, native Pyropia, Porphyra and Wildemania species, which are better adapted to the prevailing conditions of the Gulf of Maine (Blouin et al. Citation2007, Citation2011).
Pyropia farming in Alaska
The University of Alaska engaged in a 3-year programme funded by the Saltonstall-Kennedy Grant Program in the late 1990s. Research focussed on controlling the life cycles of several different species of Pyropia, including Py. abbottiae, Py. torta, Py. pseudolinearis (Ueda) N.Kikuchi, M.Miyata, M.S.Hwang & H.G.Choi, Py. fallax (S.C.Lindstrom & K.M.Cole) S.C.Lindstrom, Py. cuneiformis (= Wildemania amplissima), and Py. pseudolanceolata Ueda complex (Stekoll et al. Citation1999). Results of studies that were conducted on the response of conchocelis cultures to applied phytohormones found that higher concentrations of plant hormones increased the growth of Pyropia conchocelis but did not directly induce conchosporangial formation (Lin & Stekoll Citation2007). Juvenile blades of Py. torta showed differential growth depending on seeding density and substratum composition (Conitz et al. Citation2013). Photosynthesis of the small blades of Py. torta was maximal at 30 psu salinity, 12 °C and over 160 μmol m−2 s−1 photon fluence rate (Conitz et al. Citation2001). Photosynthesis and respiration rates were investigated for free-living conchocelis cultures (Lin et al. Citation2008). A multifactored experiment showed the importance of nitrogen in the growth of juvenile blades (Conitz et al. Citation2001). Several Pyropia species were exposed to various combinations of irradiance, photoperiod and temperature in an effort to define conditions that would reliably induce conchospore maturation and release (Lindstrom et al. Citation2008). Although some success was achieved, only Py. torta could be consistently induced to release spores. Preliminary experiments with shell cultures of the conchocelis phase led to the development of techniques for maintaining seeded shells over extended periods. Nets seeded with Py. torta from free-living conchocelis were placed in both the greenhouse and in the field for grow-out. Success in outplanting depended on the time of year, method of outplanting and genetic isolate (Stekoll et al. Citation1999). Seawater quality was monitored periodically to correlate with outplantings. Surface water temperatures were low, and salinity and nutrients were high in winter. In late summer, water temperatures were high and nutrients and salinity were very low. Currently, no species of Pyropia has been developed to the point where commercial production is feasible in Alaska.
Challenges and Lessons Learned
Alaska has set up a process for the permitting of aquatic farms jointly administrated by the Alaska Department of Fish and Game (ADFG) and the Alaska Department of Natural Resources (ADFG Citation2019). In 2015, there were 54 permitted aquatic farms, only a few of which were permitted for seaweed (Pring-Ham & Politano Citation2016).
The state of Alaska bans the import of nonindigenous species. Therefore, for now, only two species of Pyropia would appear to be candidates for further development. These are Py. abbottiae and Py. torta, or the ‘black seaweeds’. The conditions required for Py. abbottiae to reliably produce conchospores are still unknown in Alaska. Initial work indicated that conditions are not the same as those found for this species in Washington state (Lindstrom et al. Citation2008). The main obstacle with Py. torta is finding environmental conditions and/or a strain that will produce conchospores en masse in order to seed the cultivation nets. To date, release of conchospores takes too long, several days, to seed a net. Other obstacles are the timing and location of outplanting. Late fall may be the optimal time for outplanting because this may minimise fouling of the nets by drifting filamentous algae. Similarly, ideal locations have not been determined. It also needs to be determined whether commercial production would work better using free-living conchocelis cultures or using shell cultures (He & Yarish Citation2006; Pereira & Yarish Citation2008). Permitting is not a great hurdle because the ADFG and Alaska Department of Natural Resources have recently been processing several aquatic farm permits for kelp, and presently the NIMBY response is relatively rare. Finally, not enough is known about the population genetics of these nori species for the ADFG to create workable regulations which would govern the collection of parent plants (seed stock) and locations for seaweed farms.
Kelp farming in Alaska
Alaska has a viable fishery of herring spawn on kelp (SOK), also called ‘roe on kelp’ (Stekoll Citation2006). Each spring and into early summer, schools of herring spawn on nearshore vegetation such as eelgrass (Zostera), Fucus, Saccharina, Alaria and Macrocystis. An impoundment fishery using Macrocystis as the vegetative substratum has proved to be the most valuable. One issue for the SOK fishery is close proximity of Macrocystis beds to spawning herring. This has not been the case, and in the 1980s seaweed had to be transported nearly 1000 km from southeast Alaska to spawning grounds in Prince William Sound. A study funded by the Japan Overseas Fishery Cooperation Foundation and the National Coastal Resources Research and Development Institute was initiated in 1988 in Sitka to develop the mariculture of Macrocystis for use in the SOK fishery. The study followed the Japanese model for ‘kombu’ production (Stekoll & Else Citation1992). It was found that the plants would have to be in culture for at least 2 years because they were not sufficiently large after only a few months of growth. In addition, seeded lines put out later than January showed declining growth in summer (Stekoll Citation1989; Stekoll & Else Citation1990). High water temperatures and salinities and low nutrients in August and September weakened the plants to the extent that they were unable to survive winter. However, adding fertiliser to the outplanted lines in August enabled survival and growth throughout the winter and into spring (Stekoll Citation1999). For this trial, a slow-release solid fertiliser (Osmocote®; The Scott’s Company Citation2019) was placed in nylon sacks tied to the longline and placed between plant sections.
Challenges and Lessons Learned
It was found that starting outplanting in late fall enabled fronds to reach a size and vitality that enabled them to survive the following fall/winter, without the necessity of adding fertiliser in late summer. Outplanting sites should be located in areas of moderate swell. Grazing by sea urchins was not an issue for outplants on longlines situated above the bottom. However, there was no further need to refine the mariculture of Macrocystis after the main SOK fisheries relocated to southeast Alaska at sites much closer to natural beds.
CURRENT: SEAWEED FARMING BOOM IN THE 2010s
Seaweed farming in the Northeast
A dramatic advance in seaweed aquaculture occurred in the United States in 2010. The first commercial kelp farmer in the United States, Ocean Approved, cultivated the native kelp species Saccharina latissima, Laminaria digitata (Hudson) J.V.Lamouroux and Alaria esculenta (Linnaeus) Greville in the Gulf of Maine. This was enabled by technology transfer from the University of Connecticut, through grants from the National Oceanic and Atmospheric (NOAA) Small Business Innovation Research Program (ADFG Citation2019; SBIR Citation2010). Ocean Approved has successfully marketed its products since 2010. Before farming kelps, Ocean Approved wild harvested their kelp but could not meet the demand for volume of their customers. Ocean Approved was concerned with long-term dependence on wild-harvested kelp and related environmental impacts. The company has since developed novel kelp products; for example, freshly frozen kelp noodles, salads, slaws (Atlantic Sea Farms Citation2019). Ocean Approved has created a strong domestic kelp market because consumers appreciate fresh and fresh-frozen, locally grown kelp products.
Concurrently, seaweed farming was also being developed in southern New England for the purposes of environmental improvement, science education and the culinary market. This work was initiated by the University of Connecticut with the support of the Connecticut Sea Grant Program, the US Environmental Protection Agency’s (EPA) Long Island Sound Study and the National Fish and Wildlife Foundation. With nursery technology developed at the University of Connecticut, the cold-water brown seaweed S. latissima and the warm-temperate red seaweed Gracilaria tikvahiae McLachlan were successfully cultivated in open-water farms in Long Island Sound and New York coastal waters. After the outplanting of juveniles (under 1 mm), the kelp grew as much as 7.0 m in length and had yields of up to 24 kg FW m−1 after 6 months (from December to May) in Long Island Sound and New York estuaries (; Kim et al. Citation2015; Kraemer et al. Citation2014). Gracilaria also grew rapidly with growth rates of up to 16.5% d−1 in the New York estuary even during summer (Kim et al. Citation2014). Seaweed aquaculture provided ecosystem services by removing excess nutrients (carbon and nitrogen) from their varied habitats, potentially reducing ocean acidification (US EPA Citation2013).
Figs 4, 5 Saccharina latissima farms in Long Island Sound.
Fig. 4. Kelp outplanting using seed spool on longlines. Fig. 5. Saccharina latissima grown at Long Island Sound, Connecticut.
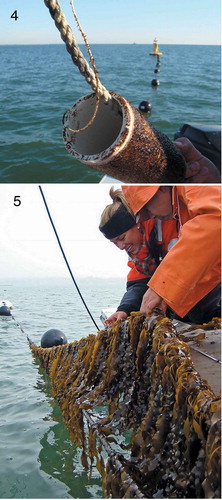
Figs 6–8. Kelp nursery systems in the United States.
Fig. 6. Standard unit of kelp nursery system using seed spools developed at the University of Connecticut. Fig. 7. Juvenile sporophytes growing on seed-string. Fig. 8. Commercial kelp nursery at Kodiak, Alaska.
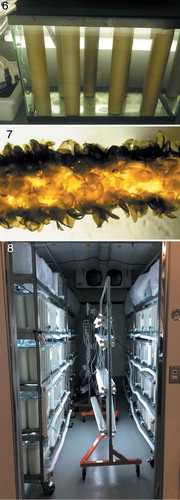
In 2011, the first commercial seaweed farm in southern New England, Thimble Island Oyster Co., started cultivation of S. latissima with the assistance of the University of Connecticut (Yarish et al. Citation2014, Citation2015). Bren Smith (owner of Thimble Island Oyster Co., New Haven, Connecticut, USA) co-founded a not-for-profit organisation called GreenWave (www.GreenWave.org) which was dedicated to outreach and extension in order to expand kelp farms regionally and nationally in the United States. Eventually, GreenWave developed a for-profit company, Sea Green Farms, LLC, which provided produce to specialty food markets and restaurants throughout the United States, with offerings of fresh and freshly frozen seaweed products. There are now more than 27 commercially operated open-water seaweed farms throughout the New England states and New York (Peconic Bays, New York), all cultivating S. latissima. Open-water Gracilaria still faces several regulatory and food safety issues. Gracilaria grows during summer when farmers are occupied with their principal business; that is, shellfish culture. Hence, farmers have limited time to devote to the cultivation of Gracilaria and its husbandry. Summer cultivation also conflicts with recreational boating and fishing. Finally, heavy fouling by other seaweeds and associated fauna on Gracilaria makes it a less desirable product for market (Concepcion et al. Citation2018; Lindell et al. Citation2015).
Kelp aquaculture is one of the fastest growing industries in the northeastern United States. Success of the industry in the Northeast is attributed to (1) the development of suitable aquaculture technologies specifically for operating in US waters, (2) strong domestic markets, and (3) strong support from coastal managers, stakeholders and the public due to the demonstrable environmental benefits provided by seaweed aquaculture. A critical approach to seaweed aquaculture in the Northeast, especially in southern New England and the New York estuary, was the overt emphasis placed on environmental benefits (ecosystem services). Long Island Sound and New York coastal waters suffer from high levels of anthropogenic eutrophication, resulting in harmful algal blooms and even hypoxia (Capriulo et al. Citation2002; Kim et al. Citation2015; Lopez et al. Citation2014; Varekamp et al. Citation2014). Seaweed aquaculture was developed in these areas to determine whether year-round seaweed aquaculture could improve water quality by removing excess nutrients. Recent studies showed that seaweed aquaculture can be an efficient way to manage nutrient issues in urbanised estuaries (Kim et al. Citation2014, Citation2015; Kraemer et al. Citation2014; Rose et al. Citation2015; Tedesco et al. Citation2014). This technology is referred to as ‘nutrient bio-extraction’ (Kim et al. Citation2017; Rose et al. Citation2015). The US EPA has acknowledged that nutrient bio-extraction is a best management practise (US EPA Citation2013). With this environmental benefit, along with the economic benefits in the Northeast, seaweed aquaculture received a lot of attention and a strong level of support from coastal managers, stakeholders and the public alike. Efforts to streamline and simplify the permitting process in Connecticut coastal waters are an ongoing process being led by the Connecticut Sea Grant, the Bureau of Aquaculture (Connecticut Department of Agriculture) and the Milford Laboratory, US National Marine Fisheries Service (DeRosia-Banick et al. Citation2015; Getchis & Rose Citation2011; Getchis et al. Citation2012; Getchis et al. Citation2017; Atlantic Sea Farms Citation2019). In addition, seaweed aquaculture was recently added to the Noninsured Crop Disaster Assistance Program in the United States, thereby enabling seaweed aquaculturists to apply for federal crop insurance (Department of Agriculture, Farm Service Agency 7 CFR Part 718, Commodity Credit Corporation 7 CFR Parts 1412, 1416, and 1437, Noninsured Crop Disaster Assistance Program; USDA Citation2014; Hurlburt Citation2016). Positive support via print, online media and social media has also enhanced the public’s perception of seaweed aquaculture in coastal waters of the United States (Aljazeera Citation2018; Mustain Citation2014; Goodyear Citation2015; Smith &; Romanoff Citation2012; Stahl Citation2018).
Nursery cultivation technologies developed for seaweeds of commercial interest at the University of Connecticut were transferred to private and public sectors throughout New England, New York and, more recently, Alaska (–; Walker Citation2018). For example, currently, several commercial-scale kelp nurseries are in operation which provide sufficient kelp seed-string for growers in the Northeast and Northwest regions of the United States (GreenWave for southern New England and New York; Maine Fresh Sea Farms, Bristol, Maine; University of New England, Biddeford, Maine; Springtide Seaweed and Ocean Approved for Gulf of Maine; and Blue Evolution, Kodiak, Alaska). The University of Connecticut and University of Alaska Southeast maintain kelp research hatcheries. Other public institutions, such as the Milford Laboratory, National Marine Fisheries Service, NOAA and Bridgeport Regional Aquaculture Science and Technology Education Center, also maintain kelp nursery systems for production, research and educational purposes.
Processing technologies have been developed in Northeastern United States for the domestic market. Ocean Approved has its own kelp processing facility in Portland, Maine, but their processing technology is proprietary. The University of Connecticut also independently developed a mobile processing facility producing fresh frozen products and transferred this novel technology to the private sector, including Maine Fresh Sea Farms and Sea Green Farms (Yarish et al. Citation2017). With these processing technologies, kelp shelf life increased significantly, from days to months or even years. Kelp products are provided sustainably to consumers year-round, helping to expand seaweed markets; however, much work remains to advance drying technology for kelp faming in the United States.
Seaweed farming in Alaska
Although for several years there has been interest in seaweed farming, as indicated by aquatic farm applications to ADFG (Pring-Ham & Politano Citation2016), commercial-scale farming of seaweeds has occurred only relatively recently. In 2015, applied research on seaweed mariculture by the University of Alaska (M. Stekoll) was funded by the private company Premium Oceanic (Blue Evolution of San Francisco, California, USA). Their initial emphasis was on the aquaculture of several seaweed species, including Saccharina latissima, S. groenlandica, Nereocystis luetkeana (K.Mertens) Postels & Ruprecht, Alaria marginata Postels & Ruprecht, Ulva sp. and Palmaria mollis (Setchell & N.L.Gardner) van der Meer & C.J.Bird. Subsequently, focus was on kelp species, using seeded strings for outplanting on longlines. Most success was achieved with S. latissima and Alaria. Follow-up funding from Sea Grant refined the methods for kelp mariculture in Alaska. Generally, the Japanese and University of Connecticut models have been followed. In brief, parent plants are collected in late summer and spore release induced onto seed-string-wrapped PVC pipes. These are incubated under specific conditions of temperature, photoperiod, light and nutrients until sporophyte blades are ready for outplanting. Optimal time for outplanting was determined to be late fall, after upwelling, which increases ambient seawater nutrient concentration. Outplants on longlines were found to grow best at 2–3 m below the surface, reaching a maximum size and quality in April–May. Quality criteria included healthy fronds without fouling. Blue Evolution, in collaboration with the University of Alaska, obtained a commercial ‘hatchery’ permit for producing seed-string of S. latissima, Alaria and other kelp species for sale to farmers. In 2016, three seaweed farms were permitted by the state: two in Kodiak and one in Ketchikan. Outplants of both Saccharina and Alaria were successful, with over 5000 kg wet weight harvested and sold to Blue Evolution (). There are now three permitted seaweed hatcheries in the state. However, only the Blue Evolution hatchery in Kodiak produced commercial seeded string in the fall of 2017 ().
Figs 9-10. Saccharina latissima harvest at Kodiak, Alaska. Photo by Tamsen Peeples, Blue Evolution, LLC.
Fig.10. Kelp farm design for the New England waters designed by C. Goudey (Goudey and Associates; Yarish et al. Citation2014).
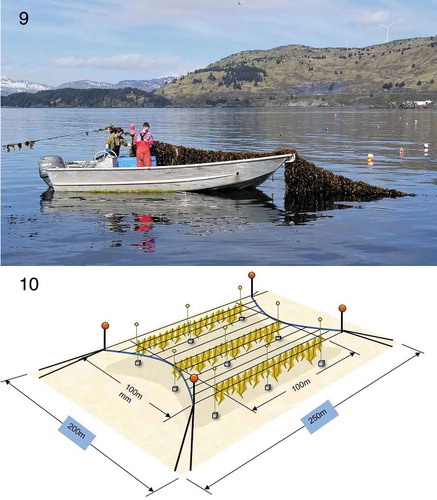
Applications for aquatic farm permits in Alaska are submitted only during the first quarter of each year. In 2017, there was a large increase in aquatic farm applications for seaweeds (C. Pring-Ham, personal communication). The state was unprepared for so many applications, and with budget cuts, only a few permits were processed in time for outplanting in the fall of 2017. Nevertheless, about 20 km of seeded strings of Saccharina, Alaria, Nereocystis and Eualaria fistulosa (Postels & Ruprecht) M.J.Wynne were produced in Blue Evolution’s Kodiak hatchery and shipped to four commercial kelp farms. Blue Evolution processed about 18,000 kg (FW) of seaweed in 2018 (Blue Evolution, personal communication).
Blue Evolution has been the main driver for seaweed aquaculture in Alaska. Its activities, along with the increasing popularity of seaweeds in the United States, created a great deal of interest in seaweed aquaculture in the state. However, there are still challenges. ADFG is concerned about the effect of farms on natural seaweed populations. The State of Alaska constitution mandates that all natural resources be managed on a sustained yield principle. ADFG has interpreted this to mean that the natural populations must be protected as much as possible. Consequently, ADFG is conservative about the effects of aquatic farms on local population genetics of natural seaweed systems. This led to regulations for seaweed farming that have been difficult to adhere to. For example, for each separate farm, 50 ‘unrelated’ fertile parent plants must be collected from different sites within 50 km of the outplanting site, and all plants need to be harvested from longlines before they become fertile. In addition, it is illegal to select for specific traits. The logic to this regulation is that genetic diversity on the longlines would be essentially clones, and if they were released into the environment, they could upset the natural genetic diversity of these seaweeds. Other issues to be addressed are (1) the delays inherent in the current permitting process, (2) cost of labour in the state, (3) costs of transportation of raw materials and products to markets, (4) the large capital expense required to start an aquatic farm (e.g. multiple permit fees, leases, insurance, equipment, uplands support for hatchery and processing), and (5) the need to develop profitable products.
In 2016, the governor of Alaska, in order to help aquatic farming become a success, created a Mariculture Task Force (ADFG, Citation2018) to make recommendations for aquaculture support in the state. This task force addressed a number of issues seen as bottlenecks for Alaskan mariculture development, and their final report (ADFG Citation2018) was officially approved by the governor in the spring of 2018.
Seaweed farming in other states
In 2015, seaweed farming in Washington state resumed in Puget Sound. The Puget Sound Restoration Fund, in collaboration with the University of Washington, Washington Sea Grant, and NOAA Pacific Marine Environmental Laboratory, received a $1.5 million grant from the Paul G. Allen Family Foundation to grow sugar and bull kelp in Puget Sound (Paul G. Allen Family Foundation Citation2015; Stahl Citation2018). The purpose of this project was to develop a local strategy to ameliorate the effects of ocean acidification. A pilot-scale co-cultivation of Gracilaria and oysters was also conducted in the Chesapeake Bay to evaluate the combined ecosystem service (i.e. nutrient bio-extraction potential) role of seaweed aquaculture, with additional support from the Maryland Sea Grant. However, these practices are still in an early stage of development or too small-scale to evaluate at this time (Li et al. Citation2012).
Challenges and Lessons Learned from Current Practices
Although seaweed aquaculture has been a considerable success economically and ecologically, especially in the Northeast during the past 8 years, challenges still exist. The permit process continues to be a major hurdle for prospective growers. Many variables must be considered in the nearshore environment when growers apply for a seaweed aquaculture permit, including recreational boat and fishing activities, marine mammal populations, and water quality (Buck Citation2007; Duff et al. Citation2003; Hopkins et al. Citation1997; Würsig & Gailey Citation2002), resulting in limited areas available for seaweed farming in nearshore environments. Offshore waters do not have as many user conflicts. Therefore, offshore areas should be considered for sustainable seaweed farming (Buck et al. Citation2004; Cicin-Sain et al. Citation2001; Tiller et al. Citation2013). To this end, the MARINER (Macro Algae Research Inspiring Novel Energy Resources) programme (ARPAe-DOE Citation2017), Advanced Research Projects Agency–Energy (ARPA-E), US Department of Energy, contracted with the NOAA in the development and deployment of information tools for mariculture (MARINER AquaMapper). Specifically, in the near term, NOAA is working to provide geospatial data to help participating MARINER-supported teams determine the scalability of their respective mariculture production and harvesting in the US exclusive economic zone (EEZ). Scalability is important because only very large farms will be economically viable for energy production. In order to better support this effort, NOAA developed biogeophysical data layers and metrics that will be used for scalability analysis in an atlas that is to be publicly released by the end of December 2018 (NCCOS Citation2019).
Global climate change has pushed some kelp populations northwards along the northeast coast of the United States. For example, some kelp species, including A. esculenta and L. digitata, have become rarer in their southern New England distribution. Populations of sugar kelp (S. latissima) also declined over recent decades (Augyte et al. Citation2017; Egan & Yarish Citation1988, Citation1990; Gerard Citation1997; Redmond Citation2013; Witman & Lamb Citation2018; Yarish et al. Citation2017). Developments of gametophyte-based seed banks and new strains of kelp produced via hybridisation may be critical to help resolve such issues. With these technologies, temperature-tolerant kelp germplasm could be provided to growers in southern New England, similar to what has been done traditionally in Asia (Hwang et al. Citation2017, Citation2018, Citation2019; Zhang et al. Citation2016, Citation2018).
Kelps have been the major seaweed aquaculture species in the United States for several reasons; for example, publicly funded cultivation technologies for nursery and open-water farming have been made available through open-source portals (Connecticut Sea Grant Citation2014; Flavin et al. Citation2013; Redmond et al. Citation2014a). Kelps are relatively easy to grow, even for novices. However, there has been demand for other seaweed species, including Palmaria, Porphyra/Pyropia, Chondrus, Gracilaria, and Sargassum. Although cultivation technologies for these species are more complex than those for kelp (Redmond et al. Citation2014a, Citation2014b), domesticating a diversified range of seaweed species will be critical for the aquaculture industry to grow sustainably in the United States. Several research projects in academia are underway to develop the required species-specific, open-water cultivation technologies (Blouin et al. Citation2007, Citation2011; Kim et al. Citation2014; Stekoll et al. Citation1999). However, to date, none of these species has been commercialised.
OFFSHORE AQUACULTURE: THE FUTURE OF SEAWEED AQUACULTURE IN THE UNITED STATES?
The year 2018 may be important in the history of seaweed aquaculture in not only the United States but possibly the world. ARPA-E of the US Department of Energy supported 18 innovative projects with a total of US$22 million to develop offshore seaweed aquaculture technologies. This programme, called MARINER, is the largest funding opportunity for seaweed aquaculture in the United States and probably one of the largest investments for offshore seaweed aquaculture in the world. The length of the US shoreline is over 20,000 km, with the longest shoreline in Alaska (over 10,000 km), followed by Florida (more than 2000 km) and Louisiana (1350 km). A number of nearshore areas are potentially available for seaweed farming. However, suitable areas for seaweed aquaculture and permits may be very limited because nearshore areas in the United States are intensively utilised for recreation, with the exception of Alaska where the major competing issues are fishing and the limited number of roads along the coast. The United States has the largest EEZ in the world at over 11,350,000 km2 (NOAA Citation2018). The EEZ may provide the best opportunities for seaweed aquaculture to expand in the United States because (1) sufficient areas to produce large amount seaweeds are available without conflict with recreational and/or fishing activities, and (2) permits for seaweed aquaculture can be more easily obtained than for nearshore waters.
The purpose of the MARINER programme is to develop critical tools that will allow the nascent macroalgal industry in the United States to leverage this tremendous resource and become a world leader in the production of marine biomass and, therefore, to improve energy security and economic competitiveness of the United States. Seaweed biomass can potentially be used for biofuels; other applications include biorefinery (Zollmann et al. 2019), human food and animal feeds. The challenge of this programme is to dramatically reduce capital and operating costs of seaweed cultivation, while significantly increasing the range of its deployment by expansion into the offshore environment (ARPAe-DOE Citation2017; ADFG Citationn.d.). Giant kelp (M. pyrifera), sugar kelp (S. latissima), Sargassum spp., Eucheuma isiforme (C.Agardh) J.Agardh and other seaweed species are planned for cultivation in the EEZ offshore environments in many biogeographic regions of the United States, including the Gulf of Mexico, Hawaii, Washington, Alaska, New England, California, and the Caribbean Sea. These projects include many different aspects of seaweed aquaculture, from breeding and seeding technologies to farm system design and management technologies (e.g. Fernández et al. 2019) to novel harvest technologies required for offshore environments (). It is expected that a selective breeding programme will improve both productivity and composition of the kelps S. latissima and M. pyrifera, which could serve as feedstock for biofuels. ARPA-E’s goal is to develop tools and a pathway towards low-cost (under US$100/dry weight [DWT]) seaweed feedstock that could supply 10% of transportation fuel in the United States (Lindell et al. Citation2018). Innovative cultivation and harvest systems also need to be developed. These include free-floating farm systems, pumping deep seawater to fuel seaweed growth; self-diving buoy systems to protect farms from wave motion; automated monitoring systems; drone technology to move farm systems to safe locations during storm events; and for harvest (ARPAe Citation2015).
CONCLUSIONS
Seaweed aquaculture history in the United States is relatively recent compared to Asian countries such as China, Korea and Japan (see Hwang et al. 2019). However, seaweed aquaculture globally is a fast-growing industry. Efforts at seaweed aquaculture in Washington state and the Gulf of Maine during the 1980s and 1990s provided important practical lessons and foundational science, and the success of kelp aquaculture since 2010 was built on knowledge obtained from earlier attempts. For seaweed aquaculture to further succeed and gain public acceptance in the United States, it is important to emphasise the associated environmental benefits. The EEZ of the United States, the blue ocean, offers opportunities for expansion of seaweed aquaculture into an environment greater than the entire land mass of the United States, in an area where there has been limited human activity. Domestication of various indigenous species is critical. Product diversification is also needed to further develop products ranging from food and phycocolloids to animal feeds and biofuels. Seaweeds for energy will be economically viable not only if oil prices increase but also if seaweed production costs can be significantly decreased, which is one goal of the MARINER programme. Therefore, continuous efforts must be made not only to increase productivity by developing cultivation technologies (nursery and open water, including offshore) and new cultivars but also to enhance biomass-to-biofuel conversion efficiency.
Acknowledgements
We thank P. Dobbins (Ocean Approved LLC), B. Smith (GreenWave) and B. Perry (Blue Evolution) for current information about industry farming. We also thank Prof. I. Levine (University of Southern Maine) and Dr C. Goudey (C. A. Goudey and Associates) for providing photos of seaweed farming and kelp farm design images, respectively.
Additional information
Funding
References
- ADFG. 2013. Alaska Department of Fish and Game. http://www.adfg.alaska.gov/sb/CSIS/; searched on 18 June 2019
- ADFG. 2018. Alaska Department of Fish and Game. Alaska Governor’s Mariculture Task Force. http://www.adfg.alaska.gov/index.cfm?adfg=amtf.main; searched on 18 June 2019.
- ADFG. 2019. Alaska Department of Fish and Game. http://www.adfg.alaska.gov/index.cfm?adfg=fishingaquaticfarming.main; searched on 18 June 2019.
- Alaska Department of Fish and Game. n.d. http://www.adfg.alaska.gov/sb/CSIS/; searched on 18 June 2019.
- Aljazeera, 2018. Food for thought: Food security, population growth & over-farming. https://www.aljazeera.com/programmes/earthrise/2018/05/food-thought-food-security-population-growth-farming-180507063857949.html; searched on 18 June 2019.
- ARPAe, 2015. BIOFUEL PRODUCTION FROM KELP. https://arpa-e.energy.gov/?q=slick-sheet-project/biofuel-production-kelp; searched on 18 June 2019.
- ARPAe-DOE. 2017. MARINER (Macroalgae Research Inspiring Novel Energy Resources). https://arpa-e.energy.gov/?q=arpa-e-programs/mariner; searched on 18 June 2019
- Aspen Research and Information Center. 1981. Aquaculture in the United States: regulatory constraints. Aspen Systems Corporation, Rockville, Maryland, USA.
- Atlantic Sea Farms, 2019. https://atlanticseafarms.com/; searched on 18 June 2019.
- Augyte S., Yarish C., Redmond S. & Kim J.K. 2017. Cultivation of a morphologically distinct strain of the sugar kelp, Saccharina latissima forma angustissima, from coastal Maine, USA, with implications for ecosystem services. Journal of Applied Phycology 29: 1967–1976. DOI:10.1007/s10811-017-1102-x.
- Blouin N., Brodie J., Pu X., Grossman A. & Brawley S.H. 2011. Porphyra: a marine crop shaped by stress. Trends in Plant Science 16: 29–37. DOI:10.1016/j.tplants.2010.10.004.
- Blouin N., Xiugeng F., Peng J., Yarish C. & Brawley S.H. 2007. Seeding nets with neutral spores of the red alga Porphyra umbilicalis (L.) Kützing for use in integrated multi-trophic aquaculture (IMTA). Aquaculture 270: 77–91. DOI:10.1016/j.aquaculture.2007.03.002.
- Brinkhuis B.H., Breda V.A., Tobin S. & Macler B.A. 1983. New York Marine Biomass Program-culture of Laminaria saccharina. Journal of the World Aquaculture Society 14: 360–379.
- Brinkhuis B.H. & Hanisak M.D. 1982. Development of a marine biomass program in New York. Proceedings of International Gas Research Conference, Government Institutes, Rockville, Maryland, USA. pp. 682–692.
- Brinkhuis B.H., Levine H.G. & Schlenk C.G. 1984a. Recent progress in the New York Marine biomass program. Proceedings of 3rd Technical Review Meeting on Energy from Biomass Illinois ANL/DOE. Argonne, Illinois, USA. 295: 304–397.
- Brinkhuis B.H., Levine H.G., Schlenk C.G. & Tobin S. 1987. Laminaria cultivation in the far east and North America. In: Seaweed cultivation for renewable resources (Ed. by K.T. Bird & P.H. Benson), pp. 107–146. Elsevier, Amsterdam, Netherlands.
- Brinkhuis B.H., Mariani E.C., Breda V.A. & Brady-Campbell M.M. 1984b. Cultivation of Laminaria saccharina in the New York Marine Biomass Program. In: Eleventh International Seaweed Symposium (Ed. by C.J. Bird & M.A. Ragan), pp. 266–271. Springer, Dordrecht, Netherlands.
- Buck B.H., Krause G. & Rosenthal H. 2004. Extensive open ocean aquaculture development within wind farms in Germany: the prospect of offshore co-management and legal constraints. Ocean and Coastal Management 47: 95–122. DOI:10.1016/j.ocecoaman.2004.04.002.
- Buck E.H. 2007. Fishery, aquaculture, and marine mammal legislation in the 110th Congress. CRS Report to Congress: RL33813. doi:10.1094/PDIS-91-4-0467B
- Capriulo G.M., Smith G., Troy R., Wikfors G., Pellet J. & Yarish C. 2002. The planktonic food web structure of a temperate zone estuary, and its alteration due to eutrophication. Hydrobiologia 475: 263−333.
- Carmona R., Kraemer G.P. & Yarish C. 2006. Exploring northeast American and Asian species of Porphyra for use in an integrated finfish–algal aquaculture system. Aquaculture 252: 54−65. DOI:10.1016/j.aquaculture.2005.11.049.
- Cheney D., B. Rudolph, L.Z. Wang, B. Metz, K. Watson, K. Roberts and I.A. Levine. 1998. Genetic manipulation and strain improvement in commercially valuable red seaweeds. In: New Developments in Marine Biotechnologies. (Ed.) Y. Le Gal & H.O. Halvorson. Plenum Press, New York, New York, USA.
- Chopin T. & Yarish C. 1998. Nutrients or not nutrients? That is the question in seaweed aquaculture … and the answer depends on the type and purpose of the aquaculture system. World Aquaculture 29: 31−33, 60–61.
- Chopin T., Yarish C., Levine I. & Van Patten P. 1998. Seaweed aquaculture. World Aquaculture 29: 17.
- Chopin T., Yarish C., Wilkes R., Belyea E., Lu S. & Mathieson A. 1999. Developing Porphyra/salmon integrated aquaculture for bioremediation and diversification of the aquaculture industry. Journal of Applied Phycology 11: 463–472. DOI:10.1023/A:1008114112852.
- Cicin-Sain B., Knecht R., Rheault R., Bunsick S., DeVoe R., Eichenberg T., Ewart J. & Halvorson H. 2001. Development of a policy framework for offshore marine aquaculture in the 3–200 mile U.S. ocean zone. Report of the Centre for the Study of Marine Policy, University of Delaware, Newark, Delaware, USA. http://udspace.udel.edu/bitstream/handle/19716/2504/sgeez1execsum.pdf?sequence=1; searched on 13 June 2018.
- Concepcion A., DeRosia-Banick K. & Balcom N. 2018. Expanding seaweed cultivation in Connecticut – addressing barriers related to public health. Abstracts of the 38th Annual Milford Aquaculture Seminar. NOAA Fisheries, Milford, Connecticut, USA. p. 21.
- Conitz J., Fagen R. & Stekoll M.S. 2013. Effects of density and substrate type on recruitment and growth of Pyropia torta (Rhodophyta) gametophytes. Botanica Marina 56: 525−533. DOI:10.1515/bot-2013-0067.
- Conitz J.M., Fagen R., Lindstrom S.C., Plumley F.G. & Stekoll M.S. 2001. Growth and pigmentation of juvenile Porphyra torta (Rhodophyta) gametophytes in response to nitrate, salinity and inorganic carbon. Journal of Applied Phycology 13: 423−431. DOI:10.1023/A:1011976431508.
- Cornish M., Critchley A. & Mouritsen O. 2017. Consumption of seaweeds and the human brain. Journal of Applied Phycology 29: 2377−2398. DOI:10.1007/s10811-016-1049-3.
- Day J.P. 2003. Effects of light and ammonium on growth, N uptake and pigmentation of P. umbilicalis Kützing, Porphyra linearis Greville, P. leucosticta Thuret in Le Jolis, Porphyra amplissima Kjellman. M.S dissertation, University of New Hampshire, Durham, New Hampshire, USA.
- DeRosia-Banick K., Carey D., Concepcion A., Balcom N., Getchis T., Greene F., Nicol J., Weeks T. & Applewhite C. 2015. Seaweed production in Connecticut: an interagency effort to establish permitting guidance for seaweed intended for human consumption. In Program and abstracts Northeast Aquaculture Conference & Exposition and the 35th Milford Aquaculture Seminar, Portland, Maine. NOAA Fisheries, Milford, Connecticut, USA. https://cpb-us-w2.wpmucdn.com/wpsites.maine.edu/dist/6/48/files/2015/05/NACE-Program-11jlutp.pdf; searched on 13 June 2018.
- Dombrowski K. 2007. Subsistence livelihood, native identity and internal differentiation in southeast Alaska. Anthropologica 49: 211−229.
- Duff J.A., Getchis T.S. & Hoagland P. 2003. A review of legal and policy constraints to aquaculture in the US Northeast. NRAC Publication No. 03-005. NRAC Project No. WP03-9. University of Maryland, College Park, Maryland, USA.
- Egan B., Garcia-Ezquivel Z., Brinkhuis B.H. & Yarish C. 1990. Genetics of morphology and growth in Laminaria from the North Atlantic Ocean—implications for biogeography. In: Evolutionary biogeography of the marine algae of the North Atlantic (Ed. by D.J. Garbary & G.R. South), pp. 147–171. Springer-Verlag, Berlin, Germany.
- Egan B., Vlasto A. & Yarish C. 1989. Seasonal acclimation to temperature and light in Laminaria longicruris de la Pyl. (Phaeophyta). Journal of Experimental Marine Biology and Ecology 129: 1–6. DOI:10.1016/0022-0981(89)90059-2.
- Egan B. & Yarish C. 1988. The distribution of the genus Laminaria (Phaeophyta) at its Southern limit in the western Atlantic Ocean. Botanica Marina 31: 155−161. DOI:10.1515/botm.1988.31.2.155.
- Egan B. & Yarish C. 1990. Productivity and life history of Laminaria longicruris at its southern limit in the western Atlantic Ocean. Marine Ecology Progress Series 67: 263–273. DOI:10.3354/meps067263.
- Fernández P.A., Leal P.P. & Henríquez L.A. 2019. Co-culture in marine farms: macroalgae can act as chemical refuge for shell-forming molluscs under an ocean acidification scenario. Phycologia 58: 542–551. DOI: 10.1080/00318884.2019.1628576.
- Flavin K., Flavin N. & Flahive B. 2013. Kelp farming manual: a guide to the process, techniques, and equipment for farming kelp in New England waters. Ocean Approved, Portland, Maine, USA. 123 pp. https://static1.squarespace.com/static/52f23e95e4b0a96c7b53ad7c/t/52f78b0de4b0374e6a0a4da8/1391954701750/OceanApproved_KelpManualLowRez.pdf.
- Flowers A.B. & Bird K. 1984. Marine biomass: a long-term methane supply option. Hydrobiologia 116–117: 272–275. DOI:10.1007/BF00027683.
- Food and Agriculture Organization. 2016. The state of world fisheries and aquaculture 2016. Contributing to food security and nutrition for all. Rome, Italy. 200 pp.
- Food and Agriculture Organization. 2018. Global world aquaculture production food and agriculture organization of the United Nations. http://www.fao.org/fishery/en; searched 01 June 2018.
- Gerard V.A. 1997. The role of nitrogen nutrition in high-temperature tolerance of the kelp Laminaria saccharina (Chromophyta). Journal of Phycology 33: 800–810. DOI:10.1111/j.0022-3646.1997.00800.x.
- Getchis T. S., Rose C.M., Carey D., Kelly S., Bellantuono K., & Francis P. 2017. Connecticut Department of Agriculture, Bureau of Aquaculture. http://www.ct.gov/doag/lib/doag/aquaculture/2017/SeaGrant_PermitGuide2017.pdf; searched on 18 June 2019.
- Getchis T.L., Rose C., Bellantuono K., Carey D. & Kelly S. 2012. Improvements to the permitting process for small-scale aquaculture in Long Island sound. In Abstracts of technical papers, Presented at the 32nd annual, Milford aquaculture seminar, Groton, CT; March 12 –14. NOAA Fisheries, Milford, Connecticut, USA. p. 216. DOI:10.1094/PDIS-11-11-0999-PDN
- Getchis T.L. & Rose C.M. 2011. Balancing economic development and conservation of living marine resources and habitats: the role of resource managers. In: Shellfish aquaculture and the environment (Ed. by S.E. Shumway), pp. 425–446. Wiley-Blackwell, Oxford, UK.
- Glenn E., Moore D., Brow J., Tanner R., Fitzsimmons K., Akutigawa M. & Napolean S. 1998. A sustainable culture system for Gracilaria parvispora (Rhodophyta) using sporelings, reef growout and floating cages in Hawaii. Aquaculture 165: 221–232. DOI:10.1016/S0044-8486(98)00263-4.
- Goodyear, D. 2015. A New Leaf: Seaweed could be a miracle food—if we can figure out how to make it taste good. https://www.newyorker.com/magazine/2015/11/02/a-new-leaf; searched on 18 June 2019.
- Guiry M.D. & Guiry G.M. 2012. AlgaeBase. World-wide electronic publication, National University of Ireland, Galway. http://www.algaebase.org; searched on 13 June 2018.
- Hall R. 2011. Multi-trophic millions. Aquaculture North America, Fish Farming in the United States, Canada and Mexico 2(2). March/April.
- Hansen G.I. 1989. Nori farming in Anacortes, Washington: a political dilemma. 13th International Seaweed Symposium (Vancouver, British Columbia) and Fourth Northwest Algal Symposium. Oregon State University Scholars, Rosario Beach, Washinton, USA. http://ir.library.oregonstate.edu/concern/defaults/1v53k318p; March 24–26.
- Harger B.W.W. & Neushul M. 1983. Test-farming of the giant kelp, Macrocystis, as a marine biomass producer. Journal of the World Aquaculture Society 14: 392–403.
- He P. & Yarish C. 2006. The developmental regulation of mass cultures of free-living conchocelis for commercial net seeding of Porphyra leucosticta from Northeast America. Aquaculture 257: 373–381. DOI:10.1016/j.aquaculture.2006.03.017.
- Hopkins D.D., Goldburg R.J. & Marston A. 1997. An environmental critique of government regulations and policies for open ocean aquaculture. Ocean & Coastal Law Journal 2: 235–260.
- Hunter C.J. 1975. Edible seaweeds – a survey of the industry and prospects for farming the Pacific Northwest. Marine Fisheries Review 37: 19–26.
- Hurlburt B. 2016. Noninsured crop disaster assistance program. Abstracts of the 36th Annual Milford Aquaculture Seminar. NOAA Fisheries, Shelton, Connecticut, USA. p. 26.
- Hwang E.K., Ha D.S. & Park C.S. 2017. Strain selection and initiation timing influence the cultivation period of Saccharina japonica and their impact on the abalone feed industry in Korea. Journal of Applied Phycology 29: 2297–2305. DOI:10.1007/s10811-017-1179-2.
- Hwang E.K., Liu F.,.L.K.H., Ha D.S. & Park C.S. 2018. Comparison of the cultivation performance between Korean (Sugwawon No. 301) and Chinese strains (Huangguan No. 1) of kelp Saccharina japonica in an aquaculture farm in Korea. Algae 33: 101–108. DOI:10.4490/algae.2018.33.2.4.
- Hwang E.K., Yotsukura N., Pang S.J., Su L. & Shan T.F. 2019. Seaweed breeding programs and progress in eastern Asian countries. Phycologia 58: 484–495. DOI: 10.1080/00318884.2019.1639436.
- Kim J.K., Kraemer G.P., Neefus C.D., Chung I.K. & Yarish C. 2007. Effects of temperature and ammonium on growth, pigment production and nitrogen uptake by four species of Porphyra (Bangiales, Rhodophyta) native to the New England coast. Journal of Applied Phycology 19: 431−440. DOI:10.1007/s10811-006-9150-7.
- Kim J.K., Kraemer G.P. & Yarish C. 2008. Physiological activity of Porphyra in relation to eulittoral zonation. Journal of Experimental Marine Biology and Ecology 365: 75−85. DOI:10.1016/j.jembe.2008.07.040.
- Kim J.K., Kraemer G.P. & Yarish C. 2014. Field scale evaluation of seaweed aquaculture as a nutrient bioextraction strategy in Long Island Sound and the Bronx River Estuary. Aquaculture 433: 148−156. DOI:10.1016/j.aquaculture.2014.05.034.
- Kim J.K., Kraemer G.P. & Yarish C. 2015. Use of sugar kelp aquaculture in Long Island Sound and the Bronx River Estuary for nutrient extraction. Marine Ecology Progress Series 531: 155–166. DOI:10.3354/meps11331.
- Kim J.K., Yarish C., Hwang E.K., Park M.S. & Kim Y.D. 2017. Seaweed aquaculture: cultivation technologies, challenges and its ecosystem services. Algae 32: 1−13. DOI:10.4490/algae.2017.32.3.3.
- Kraemer G.P., Carmona R., Chopin T., Neefus C.D., Tang X. & Yarish C. 2004. Evaluation of the bioremediatory potential of several species of the red alga Porphyra using short-term measurements of nitrogen uptake as a rapid bioassay. Journal of Applied Phycology 16: 489–497. DOI:10.1007/s10811-004-5511-2.
- Kraemer G.P., Kim J.K. & Yarish C. 2014. Seaweed aquaculture: bioextraction of nutrients to reduce eutrophication. Association of Massachusetts Wetland Scientists Newsletter 89: 16–17.
- Kraemer G.P. & Yarish C. 1999. A preliminary comparison of the mariculture potential of Porphyra purpurea and Porphyra umbilicalis. Journal of Applied Phycology 11: 473–477. DOI:10.1023/A:1008102426752.
- Langan R., Newell R.I.E. McVey J.P., Newell C., Sowles J.W., Rensel J.E.J., Grant J. & Yarish C. 2006. The United States. In: Aquaculture and ecosystems: an integrated coastal and ocean management approach (Ed. by J.P. McVey, C.S. Lee & P.J. O’Bryen), pp. 109–139. World Aquaculture Society, Portland, Maine, USA.
- Levine I. 2006. Case study on Porphyra cultivation in Maine, USA and New Brunswick, Canada. In: Aquaculture Compendium, pp. 1–13. CAB International, Wallingford, UK. https://www.cabi.org/ac; searched on 13 June 2018.
- Levine I., Watson K. & Cheney D. 2001. Marine Agronomy – the introduction of exotic or modified cultivars, is the risk worth the reward? In: Marine aquaculture and the environment: a meeting for stakeholders in the Northeast (Ed. by M.F. Tlusty, D.A. Bengston, H.O. Halvorson, S.D. Oktay, J.B. Pearc & R.B. Rheault), pp. 132–140. Cape Cod Press, Falmouth, Massachusetts, USA.
- Levine I.A. 1997. US Nori farming: an emerging industry. Phycological Newsletter 34: 16–18.
- Levine I.A. 1998. Commercial cultivation of Porphyra (nori) in the United States. World Aquaculture 29: 37–48.
- Levine I.A. & Cheney D. 1998. North American Porphyra cultivation - from molecules to markets. In: New developments in marine biotechnologies (Ed. by Y. Le Gal & H.O. Halvorson), Plenum Press, New York, New York, USA.
- Lewis R.J., Neushul M. & Harger B.W.W. 1986. Interspecific hybridization of the species of Macrocystis in California. Aquaculture 57: 203–210. DOI:10.1016/0044-8486(86)90198-5.
- Li J., Terlizzi D., & Kangas P.. 2012. Development and evaluation of eco-engineered macroalgae and shellfish multi-trophic aquaculture systems in the Chesapeake Bay. https://www.mdsg.umd.edu/research-projects/2012/raq-6. Searched on 18 June 2019
- Lin R., Lindstrom S.C. & Stekoll M.S. 2008. Photosynthesis and respiration of the conchocelis of Alaskan Porphyra (Bangiales, Rhodophyta) species in response to environmental variables. Journal of Phycology 44: 573–583. DOI:10.1111/j.1529-8817.2008.00504.x.
- Lin R. & Stekoll M.S. 2007. Effects of plant growth substances on conchocelis phase of Alaskan Porphyra (Bangiales, Rhodophyta) species in conjunction with environmental variables. Journal of Phycology 43: 1094–1103. DOI:10.1111/j.1529-8817.2007.00388.x.
- Lindell S., Fischell E., Augyte S., Bailey D., Jannink J.-L., Smith B., Wikfors G. & Yarish C. 2018. New tools for selectively improving strains of sugar kelp for food and fuel. Abstracts of the 38th Annual Milford Aquaculture Seminar. NOAA Fisheries, Milford, Shelton, Connecticut, USA. 23 pp.
- Lindell S., Green-Beach E., Bailey D., Beals M., Kim J.K. & Yarish C. 2015. Multi-cropping seaweed Gracilaria tikvahiae with oysters for nutrient bioextraction and sea vegetables in Waquoit Bay, MA. In National Shellfisheries Association 107th Annual Meeting. National Shellfisheries Association, Monterey, California, USA.
- Lindstrom S.C., Conitz J.M., Hall S. & Stekoll M.S. 2008. Induction of conchospore release: ecotypic variation in northeast Pacific species of Porphyra. Journal of Applied Phycology 20: 331–340. DOI:10.1007/s10811-007-9261-9.
- Lopez G., Carey D., Carlton J.T., Cerrato R., Dam H., Digiovanni R., Elphick C., Frisk M., Cobler C., Hice L., et al. 2014. Biology and ecology of Long Island Sound. In: Long Island Sound: prospects for the urban sea (Ed. by J.S. Latimer, M.A. Tedesco, R.L. Swanson, C. Yarish, P.E. Stacey & C. Garza), pp. 285–479. Springer, New York, New York, USA.
- Maine Department of Marine Resources. 2019. https://www.maine.gov/dmr/aquaculture/index.html; searched on 18 June 2019.
- McVey J.P., Stickney R., Yarish C. & Chopin T. 2002. Aquatic polyculture and balanced ecosystem management: new paradigms for seafood production. In: Responsible aquaculture (Ed. by R.R. Stickney & J.P. McVey), pp. 91–104. CAB International, Oxford, UK.
- Merrill J.E. 1981. Nori cultivation: pilot farm planning: final report and summary. Report to Department of Natural Resources, Olympia, Washington, USA. 60 pp.
- Minchin D. 2007. Aquaculture and transport in a changing environment: overlap and links in the spread of alien biota. Marine Pollution Bulletin 55: 302–313. DOI:10.1016/j.marpolbul.2006.11.017.
- Mouritsen O., Dawczynski C., Duelund L., Jahreis G., Vetter W. & Schröder M. 2013. On the human consumption of the red seaweed dulse (Palmaria palmata (L.) Weber & Mohr). Journal of Applied Phycology 25: 1777–1791. DOI:10.1007/s10811-013-0014-7.
- Mumford T.F., Jr. 1987. Commercialization strategy for nori farming culture in Puget Sound, Washington. In: Seaweed cultivation for renewable resources (Ed. by K.T. Bird & P.H. Benson), pp. 351–368. Elsevier, Amsterdam, Netherlands.
- Mumford T.F., Jr. 1990. Nori cultivation in North America: growth of the industry. Hydrobiologia 204/205: 89–98. DOI:10.1007/BF00040219.
- Mumford T.F., Jr. & Hansen L. 1987. A programmatic environmental impact assessment of nori farming in Washington. Department of Natural Resources, Olympia, Washington, USA. 33 pp.
- Mustain, P. 2014. Scientific American. https://blogs.scientificamerican.com/food-matters/move-over-kale-the-new-super-vegetable-comes-from-the-sea-video/; searched 18 June 2019.
- Nagler P.L., Glenn E.P., Nelson S.G. & Napolean S. 2003. Effects of fertilization treatment and stocking density on the growth and production of the economic seaweed Gracilaria parvispora (Rhodophyta) in cage culture at Molokai, Hawaii. Aquaculture 219: 379–391. DOI:10.1016/S0044-8486(02)00529-X.
- National Marine Fisheries Service Office of Science and Technology. 2016. Fisheries of the United States 2015. Department of Commerce, Washington, DC, USA. https://www.st.nmfs.noaa.gov/commercial-fisheries/fus/fus15/index; searched on 13 June 2018.
- National Research Council. 2010. Ecosystem concepts for sustainable bivalve mariculture. Committee on Best Practices for Shellfish Mariculture. The National Academies Press, Washington DC, USA. http://www.nap.edu/catalog/12802.html.
- NCCOS. 2019. National AquaMapper. https://noaa.maps.arcgis.com/apps/webappviewer/index.html?id=4f12cbde0c22488196dda69d495116cc; searched on 18 June 2019.
- Neefus C.D., Mathieson A.C., Bray T.L. & Yarish C. 2008. The distribution, morphology and ecology of three introduced Asiatic species of Porphyra (Bangiales, Rhodophyta) in the northwestern Atlantic. Journal of Phycology 44: 1399–1414. DOI:10.1111/jpy.2008.44.issue-6.
- Neushul M. 1980. Approaches to yield studies and an assessment of foreign macroalgal farming technology. Proceedings of Bio-Energy: ‘80 World Congress and Exposition. Bio-Energy Council Washington, Atlanta, Georgia. pp. 59–75.
- Neushul M. 1981. Domestication and cultivation of Californian macroalgae. Proceedings of International Seaweed Symposium 10: 71–96.
- Neushul M. 1986. Marine Farming: macroalgal production and genetics. Final Technical Report (May 1980 - December 1986). Gas Research Institute, Chicago, Illinois, USA.
- Neushul M. & Harger B.W.W. 1985. Studies of biomass yield from a near-shore macroalgal test farm. Journal of Solar Energy Engineering 107: 93–96. DOI:10.1115/1.3267661.
- Niwa K., Kikuchi N. & Aruga Y. 2005. Morphological and molecular analysis of the endangered species Porphyra tenera (Bangiales, Rhodophyta). Journal of Phycology 41: 294–304. DOI:10.1111/(ISSN)1529-8817.
- NOAA. 2018. https://oceanservice.noaa.gov/facts/eez.html. Searched on 18 June 2019
- North W.J. 1976. Aquacultural techniques for creating and restoring beds of giant kelp, Macrocystis spp. Journal of the Fisheries Research Board of Canada 33: 1015–1023. DOI:10.1139/f76-129.
- North W.J. 1987. Biology of the Macrocystis resource in North America. In: Case studies of seven commercial seaweed resources (Ed. by M.S. Doty, J.F. Caddy & B. Santelices), pp. 265–311. FAO Fisheries Technical Paper, Rome, Italy.
- North W.J. 1994. Review of Macrocystis biology. In: Biology of economic algae (Ed. by I. Akatsuka), pp. 447–527. Academic Publishing, The Hague, Netherlands.
- North W.J., Gerard V. & Kuwabara J. 1982. Farming Macrocystis at coastal and oceanic sites. In: Synthetic and degradative processes in marine macrophytes (Ed. by L.M. Srivastava), pp. 247–262. Walter de Gruyter, Berlin, Germany.
- Paul G. Allen Family Foundation, 2015. https://pgafamilyfoundation.org/news/news-articles/2015-news-items/puget-sound-ocean-acidification-grant. Search on 18 June 2019.
- Pereira R. & Yarish C. 2008. Mass production of marine macroalgae. In: Encyclopedia of ecology. Vol. 3. Ecological engineering (Ed. by S.E. Jørgensen & B.D. Fath), pp. 2236–2247. Elsevier, Oxford, UK.
- Pring-Ham C. & Politano V. 2016. 2015 annual aquatic farm status report. Alaska Department of Fish and Game, Anchorage, Alaska, USA. http://www.adfg.alaska.gov/FedAidPDFs/FMR16-23.pdf; accessed on 13 June 2018.
- Puget Sound Restoration Fund. 2019. https://restorationfund.org/programs/oceanacidification/; searched on 18 June 2019.
- Redmond S. 2013. Effects of increasing temperature and ocean acidification on the microstages of two populations of Saccharina latissima in the northwest Atlantic. MS dissertation, University of Connecticut, Groton, Connecticut, USA. Available at: https://opencommons.uconn.edu/gs_theses/515.
- Redmond S., Green L., Yarish C., Kim J.K. & Neefus C. 2014a. New England seaweed culture handbook. Nursery systems. Connecticut Sea Grant CTSG-14-01, University of Connecticut, Groton, Connecticut, USA. http://nsgl.gso.uri.edu/nhu/nhuh14001.pdf.
- Redmond S., Kim J.K., Yarish C., Pietrak M. & Bricknell I. 2014b. Culture of Sargassum in Korea: techniques and potential for culture in the U.S. Maine Sea Grant College Program, Orono, Maine, USA. http://seagrant.umaine.edu/files/SargassumManual_070914.pdf. 13 pp.
- Reed M. 1907. The economic seaweeds of Hawaii and their food value. Government Printing Office, Washington D.C., USA. 61–88 pp.
- Rose J.M., Bricker S.B., Deonarine S., Ferreira J.G., Getchis T., Grant J., Kim J.K., Krumholz J.S., Kraemer G.P., Stephenson K., et al. 2015. Nutrient bioextraction. In: Encyclopedia of sustainability science and technology (Ed. by R.A. Meyers), pp. 1–33. Springer, New York, New York, USA.
- SBIR. 2010. https://www.sbir.gov/sbirsearch/detail/15535; searched on 18 June 2019.
- Schaller M. 1992. Reckoning with Reagan: America and its president in the 1980s. Oxford University Press, New York, New York, USA. 49–52 pp.
- Smith, B. and Z. Romanoff. 2012. Farming the Urban Sea. National Geographic. https://blog.nationalgeographic.org/2012/10/09/restoring-the-urban-sea-by-farming-it/; searched on 18 June 2019.
- Squires D.F. & McKay L.B. 1983. Marine biomass: New York State species and site studies, annual report. December 1, 1981-November 30, 1982 (No. NYSERDA-83-18). New York State Energy Research and Development Authority, Albany, New York, USA.
- Stahl L. 2018. Seaweed farming and its surprising benefits. CBS News. https://www.cbsnews.com/news/seaweed-farming-and-its-surprising-benefits/?ftag=CNM-00-10aab7d&linkId=51105266; searched on 18 June 2019
- Stekoll M.S. 1989. Mariculture of the kelp, Macrocystis, in southeast Alaska. Northwest Environmental Journal 5: 141–143.
- Stekoll M.S. 1999. Marine plant mariculture in Alaska: status and research. World Aquaculture Magazine 30: 52–54, 67.
- Stekoll M.S. 2006. The seaweed resources of Alaska. In: Seaweed Resources of the World (Ed. by M. Ohno & A. Critchley), pp. 258–265. Japan International Cooperation Agency, Tokyo, Japan.
- Stekoll M.S. & Else P.V. 1990. Cultivation of Macrocystis integrifolia (Laminariales, Phaeophyta) in southeastern Alaskan waters. Hydrobiologia 204/205: 445–451. DOI:10.1007/BF00040269.
- Stekoll M.S. & Else P.V. 1992. The feasibility of Macrocystis mariculture in Southeast Alaska. State of Alaska and the Japan Overseas Fisheries Cooperation Foundation, Tokyo, Japan. 71 pp.
- Stekoll M.S., Lin R. & Lindstrom S.C. 1999. Porphyra cultivation in Alaska: conchocelis growth of three indigenous species. Hydrobiologia 398/399: 291–297. DOI:10.1023/A:1017043813609.
- Tedesco M.A., Swanson R.L., Stacey P.E., Latimer J.S., Yarish C. & Garza C. 2014. Synthesis for management. In: Long Island Sound: prospects for the Urban Sea (Ed. by J.S. By, M.A. Latimer, R.L. Tedesco, C.Y. Swanson, P.E. Stacey & C. Garza), pp. 481–539. Springer, New York, New York, USA.
- The Scott’s Company. 2019. https://www.plantersplace.com/osmocote-plant-food/osmocote-story/; searched on 18 June 2019.
- Tiller R., Gentry R. & Richards R. 2013. Stakeholder driven future scenarios as an element of interdisciplinary management tools; the case of future offshore aquaculture development and the potential effects on fishermen in Santa Barbara, California. Ocean and Coastal Management 73: 127−135. DOI:10.1016/j.ocecoaman.2012.12.011.
- Tompkins A. 1982. 1982 Gas Research Institute Marine Biomass Program Annual Report. Gas Research Institute, Chicago, Illinois, USA.
- USDA. 2014. https://www.fsa.usda.gov/Internet/FSA_Federal_Notices/nap_irm_2014.pdf; searched on 18 June 2019.
- US Environmental Protection Agency. 2013. National water program best practices and end of year performance report (Fiscal Year 2012), United States Environmental Protection Agency, Washington. http://water.epa.gov/resource_performance/performance/upload/OW_End_of_Year_BPFY2012_Report.pdf.
- Varekamp J.C., McElroy A.E., Mullaney J.R. & Breslin V.T. 2014. Metals, organic compounds, and nutrients in Long Island Sound: sources, magnitudes, trends, and impacts. In: Long Island Sound: prospects for the Urban Sea (Ed. by J.S. Latimer, M.A. Tedesco, R.L. Swanson, C. Yarish, P.E. Stacey & C. Garza), pp. 203−283. Springer, New York, New York, USA.
- Waaland J.R. 2004. Integrating intensive aquaculture of the red seaweed. Chondracanthus Exasperates. Bulletin of Fisheries Research Agency: Supplement 1: 91–100.
- Walker T. 2018. Selective breeding the next step for kelp culture. Hatchery International. May/June pp. 20–21. http://magazine.hatcheryinternational.com/publication/?i=486811&p=16#{“page”:20,”issue_id”:486811}
- Watson K., Levine I. & Cheney D.P. 2000. Biomonitoring of an aquacultured introduced seaweed, Porphyra yezoensis (Rhodophyta, Bangiophycidae) in Cobscook Bay, Maine, USA. In: Marine Bioinvasions: Proceedings of the First National Conference, MIT Sea Grant College Program, Cambridge, Massachusetts, USA. pp. 260–264.
- West A.L., Mathieson A.C., Klein A.S., Neefus C.D. & Bray T.L. 2005. Molecular ecological studies of New England species of Porphyra (Rhodophyta, Bangiales). Nova Hedwigia 80: 1–24. DOI:10.1127/0029-5035/2005/0080-0001.
- Wheeler W.N., Neushul M. & Harger B.W.W. 1981. Development of a coastal marine farm and its association problems. In: Proceedings of the Tenth International Seaweed Symposium (Ed. by T. Levring), pp. 631–636. Walter de Gruyter, Berlin, Germany.
- Witman J.D. & Lamb R.W. 2018. Persistent differences between coastal and offshore kelp forest communities in a warming Gulf of Maine. PloS ONE 13: e0189388. DOI:10.1371/journal.pone.0189388.
- Würsig B. & Gailey G.A. 2002. Marine mammals and aquaculture: conflicts and potential resolutions. In: Responsible marine aquaculture (Ed. by R.R. Stickney & J.P. McVay), pp. 45–59. CAP International Press, New York, New York, USA.
- Yarish C., Brinkhuis B.H., Egan B. & Garcia-Ezquivel Z. 1990a. Morphological and physiological bases for Laminaria selection protocols in Long Island sound. In: Economically important marine plants of the Atlantic: their biology and cultivation (Ed. by C. Yarish, C.A. Penniman & M. van Patten), pp. 53–94. The Connecticut Sea Grant College, Groton, Connecticut, USA.
- Yarish C., Chopin T., Wilkes R., Mathieson A.C., Fei X.G. & Lu S. 1999. Domestication of nori for northeast America: the Asian experience. World Aquaculture 99: 11–17.
- Yarish C. & Egan P.F. 1987. Biological studies on Laminaria longicruris and its aquaculture potential in Long Island Sound. In: Columbia University seminars on pollution and aquatic resources Vol. 20 (Ed. by G.J. Halasi-Kun), pp. 193–220. Columbia University Press, New York, New York, USA.
- Yarish C. & Egan P.F. 1989. The biology of Laminaria longicruris and its potential for mariculture in Long Island Sound. In: The second conference on the aquatic environment: problems and perspectives (Ed. by K. Kanungo, K. Brousseau, R. Singletary & L. Liscek), pp. 55–89. Western Connecticut State University, Danbury, Connecticut, USA.
- Yarish C., Frankenstein G., Sperr A.E., Fei X.G., Mathieson A.C. & Levine I. 1997. Domestication of Porphyra (nori) for Northeast America. Journal of Shellfish Research 16: 296–297.
- Yarish C., Kim J.K. & Curtis J. 2015. Seaweed aquaculture for bioextraction of nutrients from Long Island Sound. Connecticut Sea Grant College Program Interim Report, University of Connecticut, Storrs, Connecticut USA.
- Yarish C., Kim J.K. & Kraemer G.P. 2014. Nutrient bioextraction in Long Island Sound (CT, NY). National Fish and Wildlife Foundation Project, University of Connecticut, Storrs, USA. http://www.nfwf.org/whatwedo/grants/search/pages/grant-profile.aspx?EGID=33050.
- Yarish C., Kim J.K., Lindell S. & Kite-Powell H. 2017. Developing an environmentally and economically sustainable sugar kelp aquaculture industry in southern New England: from seed to market. EEB Articles 38. Available at http://opencommons.uconn.edu/marine_sci/4.
- Yarish C., Penniman C.A. & Egan B. 1990b. Growth and reproductive responses of Laminaria longicruris de la Pyl. to nutrient enrichment. Proceedings of the Thirteenth International Seaweed Symposium. Vancouver, Canada, August 13–18, 1989. Springer, Dordrecht, Germany. pp. 505–511.
- Yarish C., Wilkes R., Chopin T., Fei X.G., Mathieson A.C., Klein A.S., Friel D., Neefus C.D., Mitman G.G. & Levine I. 1998. Domesticating indigenous Porphyra (nori) species for commercial cultivation in northeast America. Journal of the World Aquaculture Society 29: 26–29, 55.
- Zhang J., Liu T., Bian D.P., Zhang L., Li X.B., Liu D.T., Liu C., Cui J.J. & Xiao L.Y. 2016. Breeding and genetic stability evaluation of the new Saccharina variety ‘Ailunwan’ with high yield. Journal of Applied Phycology 28: 3413–3421. DOI:10.1007/s10811-016-0810-y.
- Zhang J., Liu T., Feng R., Liu C., Jin Y., Jin Z. & Song H. 2018. Breeding of the new Saccharina variety “Sanhai” with high-yield. Aquaculture 485: 59–65. DOI:10.1016/j.aquaculture.2017.11.015.
- Zollmann M., Robin A., Prabhu M., Polikovsky M., Gillis A., Greiserman P.A. & Golberg A. 2019. Green technology in green macroalgal biorefineries. Phycologia 58: 516–534. DOI: 10.1080/00318884.2019.1640516.