ABSTRACT
Minerals, although required in relatively small amounts, play crucial roles in many vital processes of the human body, and their deficiency can cause several serious health issues. Seaweeds and seagrasses, traditionally consumed as vegetables in coastal areas worldwide, are generally known as a rich source of macro- and microminerals in concentrations much higher than in terrestrial plants. Despite their significant nutritional potential, the mineral composition of most of the seaweeds and seagrasses remains unexplored. In the present study, the concentrations of 23 minerals in nine seaweeds and seagrasses from the Philippines were determined using inductively coupled plasma optical emission spectroscopy. The results showed that some of the species are excellent sources of various essential minerals. Actinotrichia fragilis and Mastophora rosea were the highest in calcium content (21,511 and 14,100 mg/100 g DM, respectively), and, together with Anadyomene plicata, they were richest in copper (2.49–3.77 mg/100 g DM), iron (292–480 mg/100 g DM), manganese (22.3–27.2 mg/100 g DM), and nickel (1.49–2.93 mg/100 g DM). Moreover, M. rosea had the highest content of magnesium (2,093 mg/100 g DM). Other species, such as Enhalus acoroides (phosphorus in flowers and seeds, 277 and 282 mg/100 g DM, respectively), Halophila ovalis (silicon, 13.8 mg/100 g DM), Halymenia dilatata (zinc, 5.51 mg/100 g DM), Halymenia maculata (sulphur, 8,268 mg/100 g DM) and Portieria hornemannii (potassium, 8,985 mg/100 g DM; vanadium, 2.90 mg/100 g DM) can be highlighted as good sources of minerals. All these species have a promising potential as mineral-rich foods and can contribute to food and nutrition security.
INTRODUCTION
Minerals are micronutrients that are of fundamental importance to human nutrition and are usually classified according to average daily requirements as macrominerals, with a daily requirement equal to or above 100 mg and crucial for critical functions and maintenance of human body, and microminerals, needed in lower amounts and with an effect strongly dependent on doses (essential in specific amounts but potentially toxic when levels exceed certain thresholds) (Morris & Mohiuddin Citation2022). Although minerals are required at relatively low doses, deficiency can lead to health problems. Securing a sufficient supply of mineral-rich foods for the world growing population is therefore an important goal (Shekhar Citation2013; Quintaes & Diez-Garcia Citation2015). Mineral deficiency has become a major health burden in developing regions including Southeast Asian countries. It is globally the most important risk factor for illness and death particularly affecting hundreds of millions of pregnant women and young children. In the Philippines, iron-deficiency anaemia is the most prevalent micronutrient deficiency affecting a large proportion of infants (40.5%), pregnant women (26.4%), lactating women (16.7%) and elderly males (23.0%), while 25.6% of the population is at risk for insufficient zinc intake (WHO Citation2015; Palanog et al. Citation2019). Calcium deficiency is another problem affecting especially children between 6 months and 10 years of age, with the highest incidence in the poorest group of children (Angeles-Agdeppa et al. Citation2016).
Marine resources are crucial for food production and economic growth. Since civilizations began, the vastness and diversity of the marine ecosystem has been a source of both animal and plant foods. The total world production of wild-collected and cultivated aquatic seaweeds more than tripled between 2000 and 2018, up from 10 million tons to more than 32 million tons, dominated by countries in East and Southeast Asia (FAO Citation2020). Some seaweeds are primarily used as human food, and many others are important sources for production/extraction of alginate, agar and carrageenan used in cosmetic, pharmaceutical or food industries. In Asia and in Pacific Islands, they have traditionally been consumed and used as folk medicines. Irish moss (Chondrus crispus Stackhouse), kombu (Laminaria japonica Areschoug), green laver (Ulva lactuca Linnaeus), nori (Porphyra umbilicalis Kutzing, and other species) and wakame [Undaria pinnatifida (Harvey) Suringar] are just a few common and popular seaweeds consumed worldwide because of their known high nutritional value, represented by abundant amounts of fatty acids, fibre, minerals, proteins and vitamins. In addition to iodine, seaweeds are known to contain significant amounts of calcium, copper, iron, magnesium and potassium (Maehre et al. Citation2014; Shannon & Abu-Ghannam Citation2019; Munoz & Diaz Citation2020; Guiry & Guiry Citation2022). Similarly, as seaweeds, certain species of seagrasses are eaten as vegetables in some coastal communities. Additionally, they are valued for their ecological utility and as feed source and habitat for other marine organisms (Kim et al. Citation2021).
In the Philippines, 949 species of brown (Ochrophyta), green (Chlorophyta) and red algae (Rhodophyta) have been catalogued along its 36,289 km long coastline, having one of the highest seagrass diversities in the world (Ganzon-Fortes Citation2012; Fortes Citation2013; WEPA Citation2022). As one of the leading producers of carrageenan seaweeds, the country has well developed aquacultural and industrial production of main economically important seaweeds, such as spinosum (species of Eucheuma J. Agardh) and cottonii [Kappaphycus alvarezii (Doty) L.M. Liao] (Campbell & Hotchkiss Citation2017; Guiry & Guiry Citation2022). In many regions of the Philippine archipelago, including Mindanao Island, seagrasses and seaweeds are traditionally consumed as food and used for medicinal purposes. However, little is known about their nutritional value and chemical composition, including their mineral content (Saikia et al. Citation2020; Dumilag & Javier Citation2022). With the aim of identifying mineral-rich species of Philippine seaweeds and seagrasses that may appear promising for future food and industrial applications, in the present study we analysed the mineral composition of seven seaweeds and two seagrasses collected in Mindanao Island. The antioxidant activity of all nine species was also evaluated.
MATERIAL AND METHODS
Plant material
In total, nine seaweed and seagrass species were selected for this study: four seaweeds [Halymenia dilatata Zanardini, Halymenia maculata J. Agardh, Hydropuntia eucheumatoides (Harvey) Gurgel & Fredericq and Portieria hornemannii (Lyngbye) P.C. Silva] and two seagrasses [Enhalus acoroides (Linnaeus f.) Royle and Halophila ovalis (R. Brown) Hooker f.] were chosen based on a literature review on their traditional uses as food in the Philippines or in Southeast Asia (Fortes Citation1990; Prud’homme van Reine & Trono Jr. Citation2001; Ganzon-Fortes Citation2012; Vo et al. Citation2015; Pereira Citation2016; Syed et al. Citation2019); three other seaweed species [Actinotrichia fragilis (Forsskål) Børgesen, Anadyomene plicata C. Agardh and Mastophora rosea (C. Agardh) Setchell] were selected for the research because of their abundance in the region. Samples were collected within the intertidal and subtidal coast of Naawan (8°25.5655'N, 124°17.1780'E), Laguindingan (8°37.6487'N, 124°28.3875'E) and Initao (8°32.3782'N, 124°18.8472'E), Misamis Oriental province, in the North of Mindanao, Philippines, during low tide in the month of July and December 2016. The seaweeds and seagrasses were identified by Dr Venus Leopardas, a marine biologist of Mindanao State University at Naawan, Philippines, and the complete scientific names and authorities have been checked in AlgaeBase (Guiry & Guiry Citation2022). The voucher specimens are deposited in the herbarium of the Department of Botany and Plant Physiology of the Faculty of Agrobiology, Food and Natural Resources of the Czech University of Life Sciences, Prague, Czech Republic. The detailed ethnobotanical data on seaweeds and seagrasses are shown in .
Table 1. Ethnobotanical data on Philippine seaweeds and seagrasses.
Sample preparation and dry matter determination
Samples were washed thoroughly with water to remove foreign particles, sand and salt, and then dried in open air for 3 to 4 weeks. The residual moistures of air-dried samples were determined gravimetrically at 130°C for 1 h by a Scaltec SMO 01 analyser (Scaltec Instruments, Gottingen, Germany) according to the Official Methods of Analysis of AOAC International (Citation2012). Prior to the analyses, the air-dried material of each sample was finely ground using a Grindomix apparatus (GM100 Retsch, Haan, Germany). For the antioxidant activity testing, 5 g of ground material of each sample was extracted at room temperature in 150 ml of 80% ethanol using a laboratory orbital shaker (GFL3005, GFL, Burgwedel, Germany) at 200 rpm for 24 h. Each extract was subsequently filtered and concentrated to dryness by means of vacuum rotary evaporator R-200 (Buchi, Flawil, Switzerland) at 40°C. Dry residues were then dissolved in 100% dimethyl sulfoxide to create a stock solution of each extract at a concentration of 51.2 mg ml–1, and stored at –80°C until use.
Mineral analyses
At first, acid digestion of samples as described by Vanek et al. (Citation2010) was carried out, in which 0.5 g of powdered seaweed/seagrass material was individually placed in Teflon vessels (Savillex, Eden Prairie, Minnesota, USA) with 10 ml of 65% HNO3 p.a. (Lach-Ner, Neratovice, Czechia), closed but not sealed, and kept overnight at laboratory temperature. Afterwards, the Teflon vessels were sealed and heated at 120°C on the hot plate for 2 h. The digested solutions were transferred quantitatively to 50-ml volumetric flasks that were then filled to the mark with deionised water (conductivity 18.2 MΩ). Before analysis, the diluted solutions were filtered through 0.45-µm pore Nylon disk filters (Cronus, Sidcup, UK). Content determination of selected elements was accomplished using an inductively coupled plasma optical emission spectrometer (ICP-OES, DUO iCap 7000, Thermo Fisher Scientific, Waltham, Massachusetts, USA) operating at 1.15 kW with nebulizer and auxiliary gas flow rates of 0.5 and 1 litre min–1, respectively. Elemental analysis of 23 minerals was observed with the following spectral lines (wavelength in nm): aluminium (Al, 396.152), antimony (Sb, 206.833), arsenic (As, 189.042), barium (Ba, 493.409), beryllium (Be, 234.861), boron (B, 208.959), cadmium (Cd, 226.502), calcium (Ca, 317.933), cobalt (Co, 237.862), copper (Cu, 224.700), chromium (Cr, 267.716), iron (Fe, 259.940), lead (Pb, 220.353), magnesium (Mg, 279.079), manganese (Mn, 257.610), nickel (Ni, 221.647), phosphorus (P, 177.495), potassium (K, 766.490), silicon (Si, 251.611), sodium (Na, 589.592), sulphur (S, 180.731), vanadium (V, 292.402) and zinc (Zn, 202.548). Standard reference materials (NIST SRM 1575a Pine Needles and NCS DC 73351 Tea) and blanks were used to control the quality of digestion and analysis. The experiments were done in triplicate and the results are expressed as mean and standard deviation (s) in mg/100 g dry matter (DM). The best results were compared with already known seaweeds or foods considered as rich sources of important minerals. Data were calculated from the values available in FoodData Central databases given by United States Department of Agriculture (USDA Citation2022) and were expressed in mg/100 g of DM.
Statistical analysis for comparison of mineral concentrations between species was performed in IBM SPSS Statistics for Windows v28 (IBM Corporation, Armonk, New York, USA), using one-way ANOVA followed by Fisher’s Least Significant Difference test. The differences were considered statistically significant when p < 0.05.
Antioxidant activity evaluation
The method described by Sharma & Bhat (Citation2009) was slightly modified and used to evaluate the ability of extracts to inhibit DPPH radical. Briefly, two-fold serial dilutions of each extract were prepared in methanol (100 μl) in 96-well microtitre plates. Subsequently, 75 μl of methanol and 25 μl of 1 mM DPPH (freshly prepared in methanol) were added to each well in order to start the radical antioxidant reaction. The final concentration range of each extract and Trolox (positive control) in microtitre plate was 0.125–256 µg ml–1 (final volume of 200 μl in each well). Plates were incubated in the dark at room temperature and after 30 min the absorbance was measured spectrophotometrically at 517 nm using a Multiscan Ascent Microplate Reader (Thermo Fisher Scientific). Experiments were performed as three independent tests, each carried out in triplicate. Results were expressed as half maximal inhibitory concentration (IC50) with standard deviation (s) in µg ml–1.
RESULTS
Considering the highest concentrations of individual macrominerals in all species analysed (; Table S1), the most abundant was Ca, especially in Actinotrichia fragilis (21,511 mg/100 g DM) and Mastophora rosea (14,100 mg/100 g DM), followed by Anadyomene plicata, Enhalus acoroides (flowers) and Halophila ovalis (range 2,134–4,346 mg/100 g DM). Among the other essential macrominerals, K in Hydropuntia eucheumatoides and Portieria hornemannii (8,688 and 8,985 mg/100 g DM, respectively), Mg in M. rosea and E. acoroides (flowers; 2,093 and 1,980 mg/100 g DM, respectively), and S in Halymenia maculata (8,268 mg/100 g DM), were the most abundant. For P, higher values were determined for seagrasses (162–282 mg/100 g DM), compared to seaweeds (21.5–167 mg/100 g DM). Sodium content in tested samples showed a wide range of 947 to 10,099 mg/100 g DM, with the lowest concentration for E. acoroides (seeds) and the highest for H. maculata.
Fig. 1. Concentrations of macrominerals in Philippine seaweeds and seagrasses. All the results are presented as a mean value ± standard deviation (s) obtained from three independent experiments and expressed in mg/100 g of dry matter. Statistically significant differences (p < 0.05) of mineral concentrations between species tested are illustrated by letters (A, B, C, D, E, F, G, H, I): for all variables with the same letter, the difference between the means is not statistically significant; if two variables have different letters, they are significantly different.
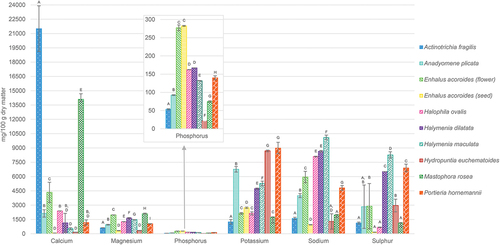
Among essential microminerals (; Table S2), Fe showed the largest concentrations with the highest amounts detected in A. fragilis, A. plicata and M. rosea (292–480 mg/100 g DM). These three species were also highest in the content of Cu (2.49–3.77 mg/100 g DM), Mn (22.3–27.2 mg/100 g DM) and Ni (1.49–2.93 mg/100 g DM). Zinc was the most abundant in H. dilatata (5.51 mg/100 g DM), although the rest of the samples also showed quite high contents of this element (1.11–2.71 mg/100 g DM). Other microminerals have been determined in higher amount especially in A. fragilis (Cr, 0.77 mg/100 g DM), A. plicata (B, Cr and V, 25.3, 1.17 and 2.02 mg/100 g DM, respectively), H. dilatata (Co, 1.75 mg/100 g DM), H. ovalis (B and Si, 33.8 and 13.8 mg/100 g DM, respectively), M. rosea (Cr and Si, 0.93 and 11.1 mg/100 g DM, respectively) and P. hornemannii (B, Si and V, 26.8, 9.68, and 2.90 mg/100 g DM, respectively).
Fig. 2. Concentrations of essential microminerals in Philippine seaweeds and seagrasses. All the results are presented as a mean value ± standard deviation obtained from three independent experiments and expressed in mg/100 g of dry matter. Statistically significant differences (p < 0.05) of mineral concentrations between species tested are illustrated by letters (A, B, C, D, E, F, G, H, I): for all variables with the same letter, the difference between the means is not statistically significant; if two variables have different letters, they are significantly different. *, concentration under detection limit.
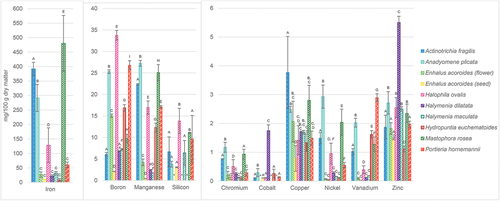
In this study, we also evaluated presence of possibly toxic minerals, namely Al, As, Ba, Be, Cd, Pb and Sb (; Table S2). The highest concentrations were identified in A. plicata (Be, 0.49 mg/100 g DM and Sb, 0.60 mg/100 g DM), H. dilatata (Cd, 0.58 mg/100 g DM), H. maculata (As, 1.91 mg/100 g DM), H. ovalis (Ba, 18.8 mg/100 g DM), M. rosea (Al, 323 mg/100 g DM) and P. hornemannii (Be, 0.49 mg/100 g DM and Pb, 0.39 mg/100 g DM). On the other hand, some of the samples did not contain any detectable concentrations of most of these elements (especially H. dilatata and flowers of E. acoroides).
Fig. 3. Concentrations of possibly toxic minerals in Philippine seaweeds and seagrasses. All the results are presented as a mean value ± standard deviation obtained from three independent experiments and expressed in mg/100 g of dry matter. Statistically significant differences (p < 0.05) of mineral concentrations between species tested are illustrated by letters (A, B, C, D, E): for all variables with the same letter, the difference between the means is not statistically significant; if two variables have different letters, they are significantly different. *, concentration under detection limit.
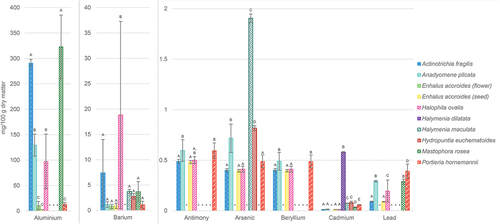
Analysis of variance indicated that there is significant difference (p < 0.05) in the proximate mineral compositions of the seaweeds and seagrasses studied. We also performed Fisher’s Least Significant Difference test to compare means of mineral contents among tested species and the results are presented as a part of .
The antioxidant activity evaluation revealed that only the E. acoroides extract was able to inhibit DPPH free radical. However, values of IC50 were quite high (213 ± 22 µg ml–1 for flowers and 229 ± 16 µg ml–1 for seeds). The rest of the species did not exhibit any antioxidant activity even at the highest concentration tested (256 µg ml–1).
DISCUSSION
The mineral analysis of Philippine seaweeds and seagrasses showed presence of significant amounts of several health beneficial macro- and microminerals, whereas possibly toxic elements were in most cases below health risk levels. To the best of our knowledge, all the seaweeds have been analysed for their mineral content for the first time here, and many of them can be highlighted as excellent sources of certain essential elements. When focusing on macrominerals, A. fragilis, A. plicata and M. rosea, together with seagrasses E. acoroides (flowers) and H. ovalis, had Ca content several times higher than recommended dietary allowance (RDA, 1,000–1,300 mg d–1; WHO/FAO Citation2004) and they are much better sources of this element than dairy products (e.g. Swiss cheese, 1,426 mg/100 g DM) or popular edible seaweeds (e.g. wakame, 750 mg/100 g DM; USDA Citation2022). Such a high content of Ca as shown by A. fragilis is typical for calcified seaweeds [e.g. Lithothamnion calcareum (Pallas) Areschoug, with approximate Ca content of 30% of seaweed thallus], which are used as an alternative natural source of Ca in animal nutrition or in agricultural fertilizers (dos Santos et al. Citation2021). It is well established that sufficient intake of Ca is required throughout a person’s growth process to prevent demineralization of the bones, hypocalcaemia, and severe conditions, such as rickets and osteoporosis (Bueno & Czepielewski Citation2008). The elevated concentrations of Ca, especially in A. fragilis and M. rosea, can significantly contribute to Ca balance in the human body since only about 25%–30% of dietary Ca is effectively absorbed (WHO/FAO Citation2004; Peacock Citation2010). In addition, these two species contained some of the lowest amounts of P, which contributes to better Ca availability, according to the previous demonstration that intestinal Ca absorption decreased with increasing dietary P (Takeda et al. Citation2014). On the other hand, P plays many important roles, especially in the formation of bones and teeth, but its intake is almost double the amount required (RDA 700 mg; Bird & Eskin Citation2021). Our samples showed similar or lower P content in comparison to other types of plant-based foods, e.g. avocado, 194 mg/100 g DM, and spinach, 570 mg/100 g DM (USDA Citation2022). In addition to Ca, seaweeds are generally considered as excellent sources of bioavailable Mg and K. These three elements are critically involved in cardiovascular functions and their high dietary intake and levels circulating in the blood have been reported to lower the risk of cardiovascular disease development (Adebamowo et al. Citation2015; Rosique-Esteban et al. Citation2018; Penalver et al. Citation2018). All tested species contained higher concentrations of Mg and K (except for A. fragilis) compared to RDA for adults (Mg, 200–350 mg d–1, K, 1,600–2,000 mg d–1; Food and Nutrition Board Citation1997) and most of the tested samples showed higher amounts of these two compounds than in popular edible seaweeds rich in K (such as laver, 2,373 mg/100 g DM) or in Mg (e.g. Irish moss, 770 mg/100 g DM) (USDA Citation2022). In human diet, special attention is paid to Na, of which the increased consumption is strongly associated with high blood pressure, with recommended maximum daily intake less than 2.3 g (FDA Citation2021). Several studies confirmed that balance between Na and K is also important, as the increased K intake resulted in increased Na excretion (Yang et al. Citation2011). Moreover, higher amounts of K have been able to block the noxious influences of Na (Langford Citation1991). Thus, seaweeds high in K, such as H. eucheumatoides and P. hornemannii, seem to be a prospective source of this element in daily diet and can provide a strategy for Na elimination in the human body.
Considering essential microminerals, A. fragilis, A. plicata and M. rosea can fully cover RDA for Fe and Cu (8–18 mg d–1 and 0.9 mg d–1, respectively; Institute of Medicine Citation2001). Moreover, they are better sources than common edible seaweeds, such as laver or Irish moss (Fe, 12 and 47 mg/100 g DM, respectively; Cu, 1.76 and 0.8 mg/100 g DM, respectively; USDA Citation2022). It is well known that Fe and Cu closely interact on various levels, for example during their absorption, red cell haemoglobin production, or proper brain function. They also have fundamental relation to antioxidants (as well as Mn, Se and Zn) and their deficit can lead to the development of anaemia (McDowell et al. Citation2007; Collins et al. Citation2013). The above-mentioned seaweed species were also shown to be excellent sources of Mn, involved in the formation of bone, and in amino acid, lipid and carbohydrate metabolism (adequate intake for adults about 2 mg d–1) as well as of Ni, cofactor or structural component of specific metalloenzymes of various functions (Institute of Medicine Citation2001).
Although seaweeds and seagrasses are nowadays often considered as ‘superfoods’ because of their undisputable superior nutritional profile and richness of essential minerals, some of the trace elements they accumulate can be toxic, especially when they are administered in higher doses. These are mainly Al, As, B, Cd, Cr and Pb. On the other hand, in very small amounts they are often considered as possibly essential trace elements, which are nowadays under increasing attention because of some promising physiological or clinical findings connected with the prevention of disease with nutritional roots, or for the enhancement of health and longevity. However, many of their biological functions in the human body are still somewhat unclear and their RDA have not been set (Nielsen Citation2000; Circuncisão et al. Citation2018). Vigilance is required especially for Ba, Be and Sb which have undoubtedly harmful effects on human health (Cooper & Harrison Citation2009a, b; WHO Citation2017). When we considered tolerable daily intake values (Ba, 210 μg kg–1 body weight, Be, 2 μg kg–1 body weight, Sb, 6 μg kg–1 body weight; WHO Citation2017), most of our samples were below the limits and can be considered as safe for consumption, especially H. dilatata for which we did not determine any detectable concentrations of these three elements. The amount of accumulated toxic compounds depends on a variety of factors, such as location, wave exposure, temperature, salinity, light, etc. Moreover, the toxicity of minerals also depends on their physical state (organic or inorganic form). Thus, the presence of potentially toxic elements must be controlled in order to meet the health and quality assurance, and the limits of potentially toxic compounds in seaweeds and seagrasses used for human consumption need to be defined (Kannan et al. Citation2011; Rubio et al. Citation2017; Circuncisão et al. Citation2018).
Although seaweeds analysed in present study have not been previously evaluated for their mineral content, several reports exist for the seagrasses E. acoroides and H. ovalis. Kannan et al. (Citation2011) analysed concentrations of 13 elements in leaves of E. acoroides and found that the most abundant was Mg (73 mg/100 mg DM), followed by Na, K and Ca (69, 24 and 22 mg/100 mg DM, respectively). These four major minerals were also the most abundant in our samples of E. acoroides (together with P and S), but in different order and in much higher concentrations, which may depend on different parts of the seagrass being tested (flowers and seeds vs leaves) and on differences in collection area (the Philippines vs India). In the case of trace elements, our results are more or less similar, and in the case of potentially toxic microminerals our contents are usually lower. In another study, Gatta et al. (Citation2020) determined relatively high levels of Ca, Fe, K and P in seeds and seedpods of E. acoroides, which, especially in the case of Ca and P, fully agree with our findings. Similarly, H. ovalis was evaluated by Wan Hazma et al. (Citation2015) as a rich source of macro- and microminerals and they indicated this seagrass as a nutrient and food source for human utilization. However, all these studies claimed that the mineral content varied greatly with the location.
As far as antioxidant activity of Philippine seaweeds and seagrasses is considered, only E. acoroides showed weak DPPH free radical scavenging effect. However, in previous studies, a strong antioxidant potential of E. acoroides was determined (e.g. Kannan et al. Citation2010; Nindatu et al. Citation2016). This disbalance in the results may perhaps be caused by the different plant parts tested, different locations of the sample collection and variability of antioxidant testing methods.
CONCLUSION
In summary, the results of analyses of Philippine seaweeds and seagrasses revealed that some of them are excellent sources of various essential minerals. Especially seaweeds A. fragilis, A. plicata and M. rosea showed interesting amounts of many of the necessary elements, such as Ca, Cu, Fe, Mg and Ni, and can be considered as potential health-promoting resources for human consumption. Other promising species seem to be E. acoroides, H. dilatata, H. maculata, H. ovalis and P. hornemannii, which are the richest in at least one of the minerals (e.g. K, P, S, Si, V or Zn). Moreover, many of the tested species were often much higher in concentration of minerals than some terrestrial plant food sources and common edible seaweeds. Among all species tested, only E. acoroides showed weak antioxidant activity. All above-mentioned species have a promising potential as mineral rich foods and can contribute to food and nutrition security.
Supplemental Material
Download MS Word (63.3 KB)DISCLOSURE STATEMENT
No potential conflict of interest was reported by the authors.
Supplementary Information
Supplemental data for this article can be accessed online at https://doi.org/10.1080/00318884.2023.2183315
Additional information
Funding
REFERENCES
- Adebamowo S.N., Spiegelman D., Flint A.J., Willett W.C. & Rexrode K.M. 2015. Intakes of magnesium, potassium, and calcium and the risk of stroke among men. International Journal of Stroke 10: 1093–1100.
- Angeles-Agdeppa I., Gironella G.M.P. & Constantino Ma.A.S. 2016. Evaluation of calcium intakes of young children in the Philippines as a result of the 2008 National Nutrition Survey. Philippine Journal of Science 145: 165–174.
- AOAC International (Association of Official Analytical Chemists) 2012. Official methods of analysis of AOAC international, ed. 19. Official Method 925.10. AOAC International, Gaithersburg, Maryland, USA. 3172 pp.
- Bird R.P. & Eskin N.A.M. 2021. The emerging role of phosphorus in human health. Advances in Food and Nutrition Research 96: 27–88.
- Bueno A.L & Czepielewski M.A. 2008. The importance for growth of dietary intake of calcium and vitamin D. Jornal de Pediatria 84: 386–394.
- Campbell R. & Hotchkiss S. 2017. Carrageenan industry market overview. In: Tropical seaweed farming trends, problems and opportunities (Ed. by A. Hurtado, A. Critchley & I. Neish), pp 193–205. Springer International Publishing, Cham, Switzerland. [Developments in Applied Phycology 9.]
- Circuncisão A.R., Catarino M.D., Cardoso S.M. & Silva A.M.S. 2018. Minerals from macroalgae origin: health benefits and risks for consumers. Marine Drugs 16: Article 400.
- Collins J.F., Prohaska J.R. & Knutson M.D. 2013. Metabolic crossroads of iron and copper. Nutrition Reviews 68: 133–147.
- Cooper R.G. & Harrison A.P. 2009a. The exposure to and health effects of antimony. Indian Journal of Occupational & Environmental Medicine 13: 3–10.
- Cooper R.G. & Harrison A.P. 2009b. The uses and adverse effects of beryllium on health. Indian Journal of Occupational & Environmental Medicine 13: 65–76.
- dos Santos L.B.D., Genova J.L., Carvalho P.L.D., Rupolo P.E. & Carvalho S.T. 2021. Calcitic seaweed (Lithothamnion calcareum) as an organic source of calcium in piglet feeding. Animal Production Science 61: 662–672.
- Dumilag R.V. & Javier R.F. 2022. Ethnobotany of medicinal seaweeds of Ilocos Norte, Philippines. Philippine Journal of Science 151: 1135–1156.
- FAO 2020. The state of world fisheries and aquaculture 2020: sustainability in action. Food and Agriculture Organization, Rome, Italy.
- FDA (U.S. Food and Drug Administration) 2021. Sodium in your diet. FDA, Silver Spring, Maryland, USA.
- Food and Nutrition Board 1997. Dietary reference intakes for calcium, phosphorus, magnesium, vitamin D, and fluoride. National Academy Press, Washington DC, USA.
- Fortes M.D. 1990. Seagrasses: a resource unknown in the ASEAN region. International Center for Living Aquatic Resources Management, Manila, Philippines. 46 pp. [ICLARM Education Series 5.]
- Fortes M.D. 2013. A review: biodiversity, distribution and conservation of Philippine seagrasses. Philippine Journal of Science 142: 95–111.
- Ganzon-Fortes E.T. 2012. A historical account of biodiversity studies on Philippine seaweeds (1800–1999). Coastal Marine Science 35: 182–201.
- Gatta R., Nessa N., Jompa J. & Ambo-Rappe R. 2020. Spatial variation of nutritional content in Enhalus acoroides (L.F.) Royle seeds and seed pods. Future of Food: Journal on Food, Agriculture and Society 8: 1–12.
- Guiry M.D. & Guiry G.M. 2022. AlgaeBase. World-wide electronic publication, National University of Ireland, Galway, Ireland. http://www.algaebase.org; searched on 14 June 2022.
- Institute of Medicine 2001. Dietary reference intakes for vitamin A, vitamin K, arsenic, boron, chromium, copper, iodine, iron, manganese, molybdenum, nickel, silicon, vanadium, and zinc. National Academies Press, Washington DC, USA. 800 pp.
- Kannan R.R.R., Arumugam R. & Anatharaman P. 2010. In vitro antioxidant activities of ethanol extract from Enhalus acoroides (L.F.) Royle. Asian Pacific Journal of Tropical Medicine 3: 898–901.
- Kannan R.R.R., Arumugam R. & Anatharaman P. 2011. Chemometric studies of multielemental composition of few seagrasses from Gulf of Mannar, India. Biological Trace Element Research 143: 1149–1158.
- Kim D.H., Mahomoodally M.F., Sadeer N.B., Seok P.G., Zengin G., Palaniveloo K., Khalil A.A., Rauf A. & Rengasamy K.R.R. 2021. Nutritional and bioactive potential of seagrasses: a review. South African Journal of Botany 137: 216–227.
- Langford H.G. 1991. Sodium-potassium interaction in hypertension and hypertensive cardiovascular disease. Hypertension 17: 155–157.
- Maehre H.K., Malde M.K., Eilertsen K.-E. & Elvevoll E.O. 2014. Characterization of protein, lipid and mineral contents in common Norwegian seaweeds and evaluation of their potential as food and feed. Journal of the Science of Food and Agriculture 94: 3281–3290.
- McDowell L.R., Wilkinson N., Madison R. & Felix T. 2007. Vitamins and minerals functioning as antioxidants with supplementation considerations. Proceedings of the Florida Ruminant Nutrition Symposium. Best Western Gateway Grand, Gainesville, Florida, USA.
- Morris A.L. & Mohiuddin S.S. 2022. Biochemistry, nutrients. StatPearls Publishing, Florida, USA.
- Munoz I.L. & Diaz N.F. 2020. Minerals in edible seaweed: health benefits and food safety issues. Critical Reviews in Food Science and Nutrition 62: 1592–1607.
- Nielsen F.H. 2000. Possibly essential trace elements. In: Clinical nutrition of the essential trace elements and minerals (Ed. by J.D. Bogden & L.M. Klevay). Humana Press, Totowa, New Jersey, USA.
- Nindatu M., Noya F., Seimahuira T., Kaya E., Wakano D. & Leasa M. 2016. Potential of Lamun (Enhalus acoroides) seeds from West Seram coastal area as natural antioxidant. Research Journal of Pharmaceutical, Biological and Chemical Sciences 7: 66–71.
- Palanog A.D., Calayugan M.I.C., Descalsota-Empleo G.I., Amparado A., Inabangan-Asilo M.A., Arocena E.C., Cruz P.C.S., Borromeo T.H., Lalusin A., Hernandez J.E. et al. 2019. Zinc and iron nutrition status in the Philippines population and local soils. Frontiers in Nutrition 6: Article 81.
- Peacock M. 2010. Calcium metabolism in health and disease. Clinical Journal of the American Society of Nephrology 5: S23–S30.
- Penalver R., Lorenzo J.M., Ros G., Amarowicz R., Pateiro M. & Nieto G. 2018. Seaweeds as a functional ingredient for a healthy diet. Marine Drugs 18: Article 301.
- Pereira L. 2016. Edible seaweeds of the world. CRC Press, Boca Raton, Florida, USA. 464 pp.
- Prud’homme van Reine W.F. & Trono Jr. G.C. [Eds] 2001. Plant resources of South-East Asia, 15(1). Cryptogams: algae. Backhuys Publishers, Leiden, Netherlands. 318 pp.
- Quintaes K.D. & Diez-Garcia R.W. 2015. The importance of minerals in the human diet. In: Handbook of mineral elements in food (Ed. by M. de la Guardia & S. Garrigues), pp 1–18. Wiley-Blackwell, Hoboken, New Jersey, USA.
- Rosique-Esteban N., Guasch-Ferre M., Hernandez-Alonso P. & Salas-Salvado J. 2018. Dietary magnesium and cardiovascular disease: a review with emphasis in epidemiological studies. Nutrients 10: Article 168.
- Rubio C., Napoleone G., Luis-Gonzalez G., Gutierrez A.J., Gonzalez-Weller D., Hardisson A. & Revert C. 2017. Metals in edible seaweed. Chemosphere 173: 572–579.
- Saikia S., Mahnot N.K., Sahu R.K. & Kalita J. 2020. Edible seaweeds as potential source of nutraceuticals. In: Marine niche: applications in pharmaceutical sciences (Ed. by N.M. Nathani, C. Mootapally, I.R. Gadhvi, B. Maitreya & C.G. Joshi), pp 183–201. Springer Nature, Singapore.
- Shannon E. & Abu-Ghannam N. 2019. Seaweeds as nutraceuticals for health and nutrition. Phycologia 58: 563–577.
- Sharma O.P. & Bhat T.K. 2009. DPPH antioxidant assay revisited. Food Chemistry 113: 1202–1205.
- Shekhar C. 2013. Hidden hunger: addressing micronutrient deficiencies using improved crop varieties. Chemistry & Biology 20: 1305–1306.
- Syed N.N.F., Zakaria M.H., Bujang J.P., Ramaiya S.D. & Hayashizaki K. 2019. Physicochemical properties of starches from seed and rhizome of Enhalus acoroides. Philippine Journal of Natural Sciences 24: 27–33.
- Takeda E., Yamamoto H., Yamanak-Okumura H. & Taketani Y. 2014. Increasing dietary phosphorus intake from food additives: potential for negative impact on bone health. Advances in Nutrition 5: 92–97.
- USDA (United States Department of Agriculture) 2022. FoodData Central. U.S. Dept. of Agriculture – Agricultural Research service, Washington, DC, USA. https://fdc.nal.usda.gov/index.html; searched on 28 June 2022.
- Vanek A., Komarek M., Chrastny V., Becka D., Mihaljevic M., Sebek O., Panuskova G. & Schusterova Z. 2010. Thallium uptake by white mustard (Sinapis alba L.) grown on moderately contaminated soils—Agro-environmental implications. Journal of Hazardous Materials 182: 303–308.
- Vo T.D., Nishihara G.N., Kitamura Y., Shimada S., Kawaguchi S. & Terada R. 2015. The effect of irradiance and temperature on the photosynthesis of Hydropuntia edulis and Hydropuntia eucheumatoides (Gracilariaceae, Rhodophyta) from Vietnam. Phycologia 54: 24–31.
- Wan Hazma W.N., Muta Harah Z., Japar Sidik B. & Natrah F.M.I. 2015. Macro and micro nutrients of tropical seagrasses, Halophila ovalis, H. spinulosa and Halodule uninervis in Johore, Malaysia. Iranian Journal of Fisheries Sciences 14: 246–261.
- WEPA 2022. Water Environment Partnership in Asia. The Ministry of the Environment, Tokyo, Japan. http://www.wepa-db.net/policies/state/philippines/seaareas.html; searched on 14 June 2022.
- WHO 2015. The global prevalence of anaemia in 2011. World Health Organization, Geneve, Switzerland. 48 pp.
- WHO 2017. Guidelines for drinking-water quality, ed. 4, incorporating the 1st addendum. World Health Organization, Geneve, Switzerland. 631 pp.
- WHO/FAO 2004. Vitamin and mineral requirements in human nutrition: report of a joint FAO/WHO expert consultation. World Health Organization, Geneve, Switzerland. 341 pp.
- Yang Q., Liu T., Kuklina E.V., Flanders W.D., Hong Y., Gillespie C., Chang M-H., Gwinnn M., Dowling N., Khoury M.J. & Hu F.B. 2011. Sodium and potassium intake and mortality among US adults: prospective data from the third national health and nutrition examination survey. Archives of Internal Medicine 171: 1183–1191.