Abstract
As parental ages at birth continue to rise, concerns about the effects of fertility postponement on offspring are increasing. Due to reproductive ageing, advanced parental ages have been associated with negative health outcomes for offspring, including decreased longevity. The literature, however, has neglected to examine the potential benefits of being born at a later date. Secular declines in mortality mean that later birth cohorts are living longer. We analyse mortality over ages 30–74 among 1.9 million Swedish men and women born 1938–60, and use a sibling comparison design that accounts for all time-invariant factors shared by the siblings. When incorporating cohort improvements in mortality, we find that those born to older mothers do not suffer any significant mortality disadvantage, and that those born to older fathers have lower mortality. These findings are likely to be explained by secular declines in mortality counterbalancing the negative effects of reproductive ageing.
Introduction
In recent decades, the postponement of parenthood has been one of the most prominent demographic trends across high-income countries (United Nations Citation2014). As (a) shows, there has been a remarkable increase in the mean age at childbearing across the world's most advanced economies since the 1970s. In 2014, the mean maternal age at childbearing was above 30 in Austria, Finland, France, Germany, Italy, Japan, the Netherlands, Norway, Spain, Switzerland, Sweden, and the UK, with the United States not far behind (Human Fertility Database Citation2016). The trends are similar for paternal age. Another of the most remarkable demographic developments of the past century has been the secular increase in longevity, illustrated in (b). Studies have documented clear and steady improvements in both period and cohort mortality, with life expectancy at birth continuing to increase across most of the world (Oeppen and Vaupel Citation2002; Wilmoth Citation2005; Rau et al. Citation2008; Shkolnikov et al. Citation2011). In this study we evaluate whether these two striking demographic developments interact with one another.
Figure 1 (a) Mean age at childbearing for women and (b) life expectancy at birth for men and women combined, in eight developed countries, 1900–2014
Note: Life expectancy at birth in Germany refers to West Germany. Exact years included are dependent on data availability.
Sources: Human Fertility Database and Human Mortality Database.
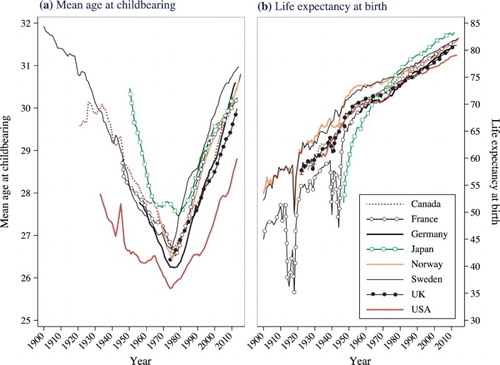
Previous research has indicated that, compared with younger maternal ages, advanced maternal age at the time of birth is associated with worse birth outcomes (Cnattingius et al. Citation1992; Andersen et al. Citation2000), and may also be associated with worse long-term health, including higher mortality during adulthood (Kemkes-Grottenthaler Citation2004; Smith et al. Citation2009). Although older parents typically have greater socio-economic resources, an increase in the mean age at childbearing combined with the negative effects of reproductive ageing could have important consequences for population health. On the other hand, increasing parental age naturally parallels the linear passage of chronological time. For any particular woman or man, having a child at a later age also means that the birth will occur in a later calendar year. Given the remarkable declines in mortality for succeeding cohorts, this later timing of birth is likely to lead to longer lives for such children. In this study we use Swedish population data to consider explicitly how secular improvements in longevity may counterbalance or even outweigh any potential negative effects of reproductive ageing. We expect that secular declines in mortality will counterbalance the negative effects of reproductive ageing, with children born to older mothers and fathers living longer, or at least not suffering any disadvantage from the biological age of their parents.
Parental age and offspring longevity: counterbalancing processes
There are at least three important dimensions to consider when explaining the relationship between parental age at childbearing on offspring health and longevity: physiological effects, socio-economic status, and macro-level trends in mortality. We discuss each of these in turn.
The first dimension is the physiological effect of parental age on offspring health, which is attributable to reproductive ageing (Hassold and Hunt Citation2001; Tatone et al. Citation2008; Kong et al. Citation2012). Human reproductive systems deteriorate with increasing age. Increasing maternal age is associated with the accumulation of DNA damage in the germ cells and decreasing embryo viability, leading to lower fecundity (Abdalla et al. Citation1997). Studies have shown that the likelihood of spontaneous abortion, stillbirth, and Down syndrome, as well as the risk of poor perinatal outcomes such as pre-term birth and low birth weight, increase exponentially for potential mothers from around age 25 (Cnattingius et al. Citation1992; Andersen et al. Citation2000). The offspring of older mothers are also at a greater risk of developing childhood cancers (Yip et al. Citation2006). The age of the father also matters. Increasing paternal age is an important determinant of de novo mutations in the male germ cells (Kong et al. Citation2012), and later paternal age has been found to be associated with increased risks of schizophrenia and autism (Sipos et al. Citation2004; Hultman et al. Citation2011). Studies have also indicated that advanced parental age may be associated with long-term consequences for health: the offspring of older parents experience higher mortality and lower reproductive fitness in adulthood (Kemkes-Grottenthaler Citation2004; Smith et al. Citation2009; Gavrilov and Gavrilova Citation2012; Gillespie et al. Citation2013), and the children of older mothers are particularly less likely to survive to be centenarians (Gavrilov and Gavrilova Citation2014, Citation2015; Jarry et al. Citation2014). Furthermore, those born to older parents are more likely to develop Alzheimer's disease or schizophrenia in adulthood (Rocca et al. Citation1991; Sipos et al. Citation2004). The negative consequences of advanced parental age are primarily concentrated among offspring born to parents aged 35 or older.
Although the weight of evidence strongly suggests that reproductive ageing is associated with worse health outcomes for offspring, recent research has also suggested that having an older father could have beneficial effects for offspring longevity, due to variation in telomere length by paternal age. Telomeres are repeating DNA sequences at the ends of chromosomes that preserve genetic integrity as cells divide (Eisenberg et al. Citation2012). Telomere length decreases as a consequence of cell division and DNA replication, as well as from oxidative stress. As a result, telomere length generally decreases with increasing age (Watson Citation1972; Richter and von Zglinicki Citation2007). Shorter telomeres are associated with a host of negative health outcomes, including an increased risk of cardiovascular disease (Benetos et al. Citation2001), diabetes (Salpea et al. Citation2010), and all-cause mortality (Cawthon et al. Citation2003). However, there is an exception to this general rule: telomere length actually increases with paternal age in human sperm (Allsopp et al. Citation1992). As a consequence, the children of older fathers have longer telomeres and this effect is cumulative across generations. That is, having an older grandfather is associated with greater telomere length, even net of the age of the father at the time of birth (Eisenberg et al. Citation2012). As a result, the negative effect on health of an increase in de novo mutations in the male germ line with increasing paternal age may be counterbalanced by the beneficial effect on health of increasing telomere length. Telomere length among offspring does not vary by age of mother, because women are born with the full pool of primary oocytes that they will carry over the life course.
The second factor influencing the relationship between parental age at childbearing and offspring longevity is parental socio-economic status. Compared with younger mothers of the same cohort, older mothers typically have higher levels of education (Lappegård Citation2000), greater incomes, and higher occupational status (McLanahan Citation2004; Powell et al. Citation2006). Due to assortative mating they tend to be partnered with men who also have high socio-economic status (McPherson et al. Citation2001; Mare Citation2016). Studies also show that older parents are more likely to be in stable relationships (Thomson et al. Citation2014) and, relative to younger couples, they are happier after they have children (Margolis and Myrskylä Citation2011; Myrskylä and Margolis Citation2014). Childbearing in the teenage years or in early adulthood is most common among men and women from less advantaged socio-economic backgrounds (Hoffman et al. Citation1993), and even when this is not the case, childbearing at relatively early ages tends to disrupt educational and occupational trajectories, leading to lower socio-economic attainment and worse health for those parents (Klepinger et al. Citation1995; Barclay et al. Citation2016). As a consequence, the children of older parents are generally the beneficiaries of greater resources and higher parenting quality (Powell et al. Citation2006; Kalil et al. Citation2012). This disparity in the resources available to children of parents with high vs. low socio-economic statuses has been growing since at least the 1970s (McLanahan Citation2004). Given the strong relationship between socio-economic status and health (Mackenbach et al. Citation1997; Torssander and Erikson Citation2010), parental socio-economic status may offset or counterbalance the negative effects of reproductive ageing documented by previous research.
A third factor that is of critical importance in explaining the longevity of children by parental age at the time of birth is macro-level trends in mortality. For any prospective parent, delaying parenthood means that their child will be born at a later date. Until recently the conceptual importance of this factor has been ignored by those researching this topic. Recent research that has explicitly considered the importance of cohort improvements over time has shown that on average the children of older mothers have a higher IQ, attain more education, and are taller (Myrskylä et al. Citation2013; Barclay and Myrskylä Citation2016a). These advantages are attributable to increasing IQ scores at the population level (Flynn Citation1987), educational expansion (Breen Citation2010), and steady improvements in population stature (Komlos and Lauderdale Citation2007). Given secular declines in mortality, this can also be expected to apply to offspring longevity, with those born to older parents benefitting from placement in a later birth cohort.
A handful of previous studies have examined the association between parental age and offspring mortality without controlling for mortality improvements over time, using data from Canada and the United States, and have shown that later maternal age is associated with a lower likelihood of surviving to age 100 or older (Gavrilov and Gavrilova Citation2014, Citation2015; Jarry et al. Citation2014). However, while these studies do provide an important insight into the potential effects of parental age on extreme longevity, the focus on survival to a specific age (e.g., 100 or 104) among a small sample rather than examining mortality across adulthood in a full population means that it is not possible to draw definitive conclusions from those findings. Indeed, on the contrary, we contend that the secular improvements in mortality over the past century should counterbalance the negative physiological effects of advanced parental age.
Mortality trends in Sweden
Human life expectancy has increased by more than two years per decade for over a century (Oeppen and Vaupel Citation2002), and Sweden has experienced a similar pattern of improvement (see (b)). Life expectancy at birth in Sweden in 1900 was 53.6 for women and 50.8 for men, but by 2014 it was 84.1 for women and 80.4 for men. In the early 1970s, Sweden was the world leader in life expectancy at birth, but it has since lost ground to countries like France and Japan (Drefahl et al. Citation2014). Akin to the pattern in other developed countries, gains in period life expectancy at birth earlier in the twentieth century are primarily attributable to declines in infant and child mortality, while improvements since the 1950s are primarily attributable to declines at older ages (Christensen et al. Citation2009). Although mortality decline among centenarians has stagnated (Drefahl et al. Citation2012), there have still been very substantial reductions in the rates of mortality attributable to neoplasms and cardiovascular diseases among pre-centenarians in Sweden (Björck et al. Citation2009; La Vecchia et al. Citation2010; Modig et al. Citation2013).
In this study we use Swedish population data to study the relationship between parental age at the time of birth and offspring mortality in adulthood over the period 1990–2012. shows age-adjusted mortality trends for 1990–2012 for all-cause mortality, and for mortality attributable to neoplasms and cardiovascular diseases separately. Men are shown in (a) and women in (b). The declines in all-cause mortality were large for both men and women during this period. In 1990, the all-cause mortality rate was 1,543 per 100,000 for men and 989 per 100,000 for women, and by 2012 had declined to 1,071 for men and 782 for women (Socialstyrelsen Citation2007, Citation2013). Declines in mortality attributable to cardiovascular diseases and neoplasms were also substantial for both men and women, but particularly so for men.
Figure 2 Age-adjusted period mortality rates for major causes of death for (a) men and (b) women, Sweden 1990–2012
Note: A log scale is used.
Sources: Socialstyrelsen [Swedish National Board of Health and Welfare] (Citation2007, Citation2013).
![Figure 2 Age-adjusted period mortality rates for major causes of death for (a) men and (b) women, Sweden 1990–2012Note: A log scale is used.Sources: Socialstyrelsen [Swedish National Board of Health and Welfare] (Citation2007, Citation2013).](/cms/asset/3270cf5d-0dd8-494d-976c-2893f29e8555/rpst_a_1411969_f0002_c.jpg)
Postponing parenthood, or continuing childbearing at older ages, means placing any such children into a later birth cohort. As a result of the declines in age-specific mortality in Sweden, clearly illustrated in and , the members of these later-born cohorts are likely to live longer than if they had been born in an earlier time period. In this study we investigate whether secular trends of increasing longevity counterbalance or outweigh the negative effects of reproductive ageing on offspring longevity, using Swedish population data to study adult mortality between ages 30 and 74.
Data and methods
Data
In this study, we use Swedish administrative register data to examine individuals born in Sweden between 1938 and 1960 inclusive. In Sweden, each individual is given a unique personal identification number (PIN). This PIN makes it possible to link an individual's records across the various administrative registers. One particularly important register for this study is the Swedish Multigenerational Register. This contains information on the PIN of each individual, as well as the PINs of the individual's parents (Statistics Sweden Citation2011). This information allows us to identify the biological mother and father of each individual and, in turn, to identify any other biological kin relations. The main family members of interest in this study are the mother, father, and siblings of the index person. We use information on the biological mother and father to determine the maternal and paternal ages at the time of birth and to identify the sibling group. The earliest birth cohort for which it is possible to link individuals to their siblings in the Swedish Multigenerational Register is 1932. However, we use cohorts born from 1938 to 1960, as previous studies have suggested that birth order may influence long-term health and mortality (Barclay and Myrskylä Citation2014; Barclay and Kolk Citation2015). Since we can only link individuals to their kin from 1932 onwards, everybody born in 1932 appears in the data as a ‘firstborn’. For a more accurate measure of birth order, we start from cohorts born in 1938.
Although we describe our methodological strategy in more detail in the following subsection, that strategy also affects the analytical sample that we use. Between 1938 and 1960 there were 2,491,059 live births in Sweden. After excluding individuals who are missing information on the PIN of the mother or father, sibling groups where any child is born outside Sweden, and sibling groups with multiple births, we are left with 1,899,314 observations. In our main analyses we use within-family sibling comparison analyses, comparing siblings who share a biological mother and father. These sibling comparison analyses rely on variance in the sibling group to estimate the importance of parental age at the time of birth. That means that our sibling fixed effect analyses are based on sibling groups where at least two siblings are observed and where at least one sibling has died. This results in an analytical sample of 319,749, of whom 117,169 have died. Although within-family sibling comparisons have a high degree of internal validity, there are limitations to generalizability when such a large proportion of the population is excluded from the analyses. To address this, we also use between-family comparisons that do not exclude one-child sibling groups or sibling groups where none of the siblings have died. The analytical sample for those analyses is 1,899,314, of whom 157,328 have died. We provide more detail on our statistical models in the following subsection.
To study mortality, we use the Swedish Mortality Register that covers the period 1960–2012 and provides information on timing of death by year and month. Although the Swedish Mortality Register contains data for the period 1960–2012, the multigenerational registers that allow family members to be linked to one another are incomplete before the 1990s (Statistics Sweden Citation2011). As a consequence, studying mortality before the early 1990s for individuals who can be linked to their kin is, in effect, still conditioning on survival to the early 1990s for members of that population. This is particularly important, because a central component of our analysis is the application of a within-family sibling comparison approach, detailed in the following subsection. We therefore choose to study mortality over the period January 1990 to December 2012. Although we could also study cohorts born later than 1960, we focus on these cohorts born 1938–60 to somewhat limit the degree of cohort and period heterogeneity in mortality patterns. Furthermore, mortality in early adulthood in Sweden is uncommon, meaning that few deaths are observed among cohorts born after 1960 in the period up to 2012, the latest year for which we have data on mortality.
Statistical analyses
To estimate the relationship between parental age and offspring mortality we use four different models. To conduct these analyses, we use the ‘st’ suite of analytical tools for survival analysis in Stata 13. Models 1 and 2 use the full cohorts of individuals born 1938–60 and use Cox proportional hazard regressions (Cox Citation1972) to examine the relationship between parental age at time of birth and offspring mortality in a between-family comparison. Models 3 and 4 use stratified Cox models, which we refer to as within-family comparison analyses, on the subset of sibling groups where at least one of the siblings has died. The stratified Cox models are analogous to sibling fixed effects models (Allison Citation2009, chapter 5), and were implemented using the ‘strata()’ option for Cox survival analysis in Stata 13. Analyses were stratified by a shared sibling group identity number (ID). The sibling group ID was defined by a shared biological mother and biological father.
The standard Cox proportional hazards model is expressed as:where h(t | X1, … , Xk) is the hazard rate for individuals with characteristics X1, … , Xk at time t, h0(t) is the baseline hazard at time t, and βj, j = 1, … , k are the estimated coefficients. Since the failure event in our analysis is the death of the individual, the baseline hazard of our model, h0(t), is age. Since we study mortality between 1990 and 2012, the age from which we begin to follow up individuals in our analysis is the age of the index person in 1990. For individuals born in 1938 that is 52, whereas for individuals born in 1960 that is age 30. We observe individuals born in 1938 between ages 52 and 74, and individuals born in 1960 between ages 30 and 52. Individuals are censored on first migration out of Sweden, at death, or in 2012; whichever comes first.
Model 1 is a descriptive model that examines the associations between maternal and paternal ages and mortality using a standard Cox model, and interacts sex of offspring with maternal and paternal age at the time of birth. Model 2 is a standard Cox model where we also use the full cohort of men and women born in Sweden between 1938 and 1960 to examine how parental age at the time of birth is related to offspring mortality. In Model 2 we control for offspring sex, offspring birth order, sibling group size, length of the birth interval preceding the birth of the index person, and highest levels of education achieved by the mother and by the father. We also include time-varying covariates for the death and migration status of the mother and father. We control for offspring sex, as some studies have documented variation in the sex ratio at birth with increasing maternal age, and sex is a key predictor of mortality in adulthood (James Citation1987). Previous research has also shown a relationship between birth order and health (Barclay and Myrskylä Citation2014; Barclay and Kolk Citation2015), and a relationship between sibling group size and health (Hart and Davey-Smith Citation2003), both of which are associated with parental age at childbearing. The length of the preceding birth interval is also associated with perinatal outcomes (Conde-Agudelo et al. Citation2006), which are in turn associated with long-term mortality (Osler et al. Citation2003). Parental education is also strongly associated with offspring health (Hayward and Gorman Citation2004), and more highly educated parents tend to have children at later ages (McLanahan Citation2004). We control for the death and migration status of the mother and father, as previous research has suggested that there is a relationship between parent–child lifespan overlap and offspring longevity (Myrskylä and Fenelon Citation2012), and this might explain the relationship between parental age at the time of birth and offspring longevity (Myrskylä et al. Citation2014). The four categories for these parental death/migration variables are: (1) alive and in Sweden; (2) emigrated and no mortality observed; (3) has died; and (4) a small number of cases where we have data on death following emigration. The length of the preceding birth interval is set to zero for firstborn siblings.
In a variant of Model 2, we interact parental birth cohort with parental age at the time of birth to examine how delaying childbearing to older ages, or continuing childbearing at older ages, is related to offspring mortality for parents born in different cohorts. In these analyses we examine the mortality of children born to parents of three different cohorts: those born in 1885–1919, 1920–29, and 1930–46.
Models 3 and 4 estimate the association between parental age and offspring longevity using a stratified Cox model, which is equivalent to a within-family sibling comparison for the subset of sibling groups. The stratified Cox model takes the following form, where the hazard for an individual from stratum s is:where h0s(t) is the baseline hazard for stratum s, s = 1, … , S. Each stratum, s, is a sibling group. Membership of a sibling group is defined by sharing a biological mother and a biological father. In the standard Cox proportional hazard regression shown earlier, the baseline hazard h0 is common to all individuals in the analysis. In the stratified Cox model, we allow the baseline hazard to differ between strata, based on the assumption that there are unobserved factors particular to each sibling group that may confound the relationship between parental age at the time of birth and offspring mortality in adulthood (Allison Citation2009, chapter 5). Such confounding factors could include genetics, parental health and health behaviours, and unmeasured aspects of parental socio-economic status that are inadequately captured by variables for educational attainment or social class. To the extent that such factors are shared by siblings, they are controlled for in the stratified Cox models.
Models 3 and 4 both include control variables for offspring sex and birth order. Model 4 also includes a control variable for birth year, using individual-year dummy variables, while Model 3 does not. The purpose of Model 3 is to investigate what we would describe as the ‘total effect’ of parental age on offspring longevity. From the perspective of any individual mother or father, giving birth at a later age also means giving birth into a later calendar year. We argue that the relationship between parental age at the time of birth and offspring mortality that is actually experienced by mothers and fathers is one that incorporates the effect of both parental age and calendar year. That is to say, parental age does not exist in a vacuum, as the children born to an older mother or father benefit from being born in a birth cohort that experiences lower mortality than if that same woman, or man, had had the children at a younger age. Model 3 captures this critical aspect of the parental age effect by: (1) comparing children born to the same parents (so that being born at an older parental age really does mean being born in a later calendar year); and (2) excluding the control variable for birth year. As a result, the estimated coefficients for parental age at the time of birth capture both parental age itself and the secular declines in mortality experienced by later-born birth cohorts.
As already stated, Model 4 is the same as Model 3, except that it includes an additional control variable for birth year, using one-year dummies. We describe this as the ‘net effect’ of parental age on offspring mortality. We present the results from Model 4 for the sake of completeness, but must mention several important qualifications. Within a sibling group, a one-year increase in parental age is exactly the same as a one-year increase in birth year. This introduces an age–period–cohort dependency into our models. Since age, period, and cohort are linearly dependent on one another, there are infinitely many solutions to any attempt to separate them. Therefore, in a within-family comparison, it is not possible to identify the effect of parental age net of the effect of birth year. It would be possible to circumvent this problem if there were significant variation in the mortality trend over time, for example, if mortality decline had been observed for some birth cohorts, but not others. Other analyses have shown that the effects of parental age on outcomes such as educational attainment and child mortality can be isolated by exploiting heterogeneity in the secular trend (Barclay and Myrskylä Citation2016b, Citation2017). Unfortunately, however, this is not a characteristic of our data. As the rising life expectancy in (b) illustrates, mortality has been declining steadily for the entire period over which we have data.
Results
Descriptive statistics
shows summary statistics for the two analytical populations used in our analyses: the full cohorts born 1938–60 and the sibling population. It can be seen that in the full population the mortality rate is lowest among those born to mothers aged 25–29, and is higher among those born to younger and older mothers. With regards to paternal age, we can see that the mortality rates are lowest among those born to fathers aged 20–39. Slightly higher mortality rates are found among those born to teenage fathers, and to fathers aged 40 or above. In the sibling population the pattern is quite different, with those born to older mothers and fathers having lower mortality rates than those born to younger parents. However, this is a consequence of conditioning the selection of the sibling population on the death of at least one of the siblings. Those deaths will be concentrated among older siblings, who will be born in earlier cohorts, and will typically be born to younger mothers and fathers. The descriptive patterns among the other variables in show that mortality is substantially lower among women than men in both the full population and in the sibling population. In the full population, mortality rates are higher following the death of either the mother or father. Since these numbers are descriptive, the death of the mother or father is also correlated with the age of the index person. Finally, it is also clear for both populations that mortality rates are substantially lower among those born in later birth cohorts.
Table 1 Descriptive statistics for all-cause mortality for Swedish men and women born 1938–60: full cohort population and sibling population
Survival analyses
and show the results from Models 1, 2, 3, and 4, which are survival analyses of the relationship between maternal and paternal age at the time of birth and offspring all-cause mortality in Sweden between 1990 and 2012, for cohorts born 1938–60. Models 1 and 2 are Cox regressions on the full population, while Models 3 and 4 are stratified Cox regressions based on the population of sibling groups where at least one sibling died during the observation window.
Figure 3 All-cause mortality by maternal age and paternal age at the time of birth, for Swedish men and women born 1938–60
Notes: Model 1 is a Cox regression adjusting for sex only. Model 2 is a fully adjusted Cox regression. Model 3 is a stratified Cox regression adjusting for sex and birth order. Model 4 is a stratified Cox regression adjusting for sex, birth order, and birth year. Error bars show 95 per cent confidence intervals.
Source: Swedish register data, authors’ own calculations.
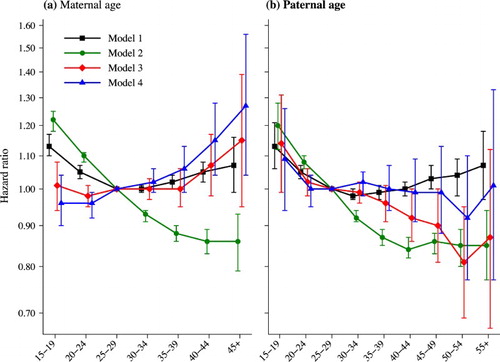
Table 2 Survival analyses of Swedish men and women born 1938–60: all-cause mortality over the period 1990–2012
Model 1 examines the relationship between parental age and offspring mortality, adjusting only for sex, while Model 2 introduces additional control variables for birth order, sibling group size, the length of the preceding birth interval, maternal and paternal educational levels, and the death and migration status of the mother and father. As can be seen from Model 1 (), individuals born to mothers and fathers aged 25–29 experience lower mortality than individuals born to younger or older parents. Those born to teenage mothers or fathers have a hazard 13 per cent higher, while those born to mothers aged 45 or older, or fathers aged 55 or older have a hazard that is 7 per cent higher, but these estimates are not very precise, probably because of small cell sizes. The results from Model 2 () show that after adjusting for various confounding factors, those born to teenage mothers or teenage fathers experience the highest mortality, and that the hazard of mortality generally decreases monotonically with increasing maternal and paternal age. We have also considered whether these results could be driven by parental cohort differences. However, in models stratified by parental cohort (shown in the supplementary material; Figure S1), the results are largely the same.
While the estimates from Model 2 are adjusted for a number of potentially confounding variables, it is very possible that there are unobserved factors that vary within sibling groups that could confound the relationship between parental age at the time of birth and offspring mortality in adulthood. This could explain why Model 2 suggests that those born to older mothers experience substantially lower mortality than those born to younger mothers. To attempt to minimize this residual confounding, we estimate sibling comparison models, which adjust for all time-invariant factors that are shared by siblings. Model 3 adjusts for birth order, giving what we describe as the ‘total effect’ of parental age at the time of birth, while Model 4 adjusts for birth year as well as birth order, giving what we describe as the ‘net effect’ of parental age.
The results from Model 3 show that the relationship between maternal age at the time of birth and mortality is completely flat up to age 35–39, by which age the vast majority of births have taken place. This indicates that maternal age at the time of birth does not have any impact at all on offspring mortality in adulthood for the majority of the population born 1938–60. The results in do show that individuals born to mothers aged 40–44 experience mortality that is 7 per cent higher, and individuals born to mothers aged 45 or older have mortality that is 15 per cent higher than individuals born to mothers aged 25–29, though these estimates are not statistically significant. An increase in mortality of 7 per cent is approximately equivalent to a life expectancy at age 30 (e30) that is eight months shorter than for offspring born to women aged 25–29, when applied to a Swedish life table from 2007. This difference in remaining e30 is slightly larger than the seasonal differences in mortality observed in Austria by Doblhammer and Vaupel (Citation2001). Although the increase in mortality associated with being born to a mother aged 45 or older is larger, at 15 per cent, this risk is only experienced by a very small fraction of the population.
The results for paternal age from Model 3 show that being born to a teenage father is associated with a 14 per cent increase in the hazard of mortality, although the confidence interval is wide. Increasing paternal age is associated with a monotonic decrease in offspring mortality up to age 50–54, at which point there is a small increase. For the most part this decline in mortality is statistically significant. These results indicate that delaying childbearing to older ages has few, if any, negative consequences for the children of the vast majority of women, and that when men delay childbearing to older ages, this actually decreases mortality among their children.
The results from Model 4 are also shown in and . Model 4 includes control variables for the index person's birth year using individual-year dummy variables. As stated in the ‘Statistical analyses’ subsection, we advise caution in the interpretation of these results. Within a sibling group, a one-year increase in maternal or paternal age is exactly the same as a one-year increase in birth year. As a consequence, it should not be possible to pick apart the difference in the effect of maternal age and birth year. Nevertheless, these estimates do suggest what would be expected theoretically: that when controlling for the benefit of being born in a later birth year, being born to older parents is associated with worse mortality outcomes. The results show an increase in mortality with increasing maternal age at the time of birth, and this increase is generally statistically significant. Relative to individuals born to mothers aged 25–29, those born to women aged 40–44 experience a 15 per cent higher hazard of mortality. The results for paternal age do not show any statistically significant results for all-cause mortality: the point estimates are flat between ages 20–24 and 45–49, and being born at a later time point is not associated with significantly lower mortality as it is in Model 3.
The reader will note that all our analyses are based on a pooled analysis of men and women. We also tested for an interaction between sex and parental age at the time of birth, and found that there was no statistically significant interaction. The estimates plotted from these models are shown in the supplementary material (Figure S2).
We also conducted additional analyses to examine the effect of heterogeneity by cause of death. The three specific causes examined were cardiovascular diseases, neoplasms, and all other remaining causes. We took account of the fact that Sweden switched from version nine to version ten of the International Classification of Diseases (ICD) in 1996 (Janssen and Kunst Citation2004). The diagnostic categories for cardiovascular diseases were 390–459 in ICD-9 and I00–I99 in ICD-10. For neoplasms the diagnostic categories were 140–239 in ICD-9 and C00–D48 in ICD-10. The results from these analyses are shown in the supplementary material (Tables S1–S3 and Figures S3–S5). The results for the two major causes of death—neoplasms and cardiovascular diseases—were generally very consistent with the results that we observe for all-cause mortality.
Discussion
This study has shown that in twentieth-century Sweden, after taking into account the benefits of being born in a later birth year with lower mortality rates, postponing fatherhood increased longevity for the offspring, and postponing motherhood had no negative effect on offspring longevity. Although previous research has examined the relationship between parental age at childbearing and offspring mortality (Kemkes-Grottenthaler Citation2004; Smith et al. Citation2009; Gavrilov and Gavrilova Citation2012; Gillespie et al. Citation2013), that body of research has generally focused on isolating the effect of parental age net of potential confounding factors. Although that is undoubtedly an important exercise, we believed that research on this topic would be positively informed by a careful consideration of the role of cohort and period trends for offspring outcomes rather than treating them as a nuisance factor to be adjusted for. This study has advanced the literature by highlighting the often-overlooked point that prospective parents are choosing not just whether to have a child at age 25 or at age 35, but whether to have a child at age 25 this year or at age 35 a decade from now. This approach provides additional nuance to the debate regarding how childbearing at advanced ages affects the offspring, particularly when considering the many positive secular trends over past decades, which are not limited to improvements in longevity.
We argue that our approach towards understanding the relationship between parental age and offspring mortality represents the most accurate portrayal of that experience from the perspective of any individual mother or father. That is, mothers who continue childbearing at older ages do not increase the mortality of their children, and fathers who continue childbearing at older ages increase the longevity of their children. While certain risks, such as involuntary childlessness, increase with fertility postponement, our results indicate that the children of older fathers in high-income Sweden live longer. Although we focus on mortality across ages 30–74, the low levels of infant, child, and early adult mortality in Sweden mean that the potential impact of mortality selection before age 30 is unlikely to be driving our results. Nevertheless, it is important to acknowledge that the children who survived to enter our analysis are necessarily those who survived to reach adult ages, and were born to parents who were able to conceive successfully and have children. The children born to parents of advanced reproductive ages are therefore necessarily drawn from a relatively healthy proportion of the population.
As shown in (b), life expectancy at birth has been increasing consistently since at least 1900 across a wide range of countries that are today classified as developed. As a consequence, we argue it is very plausible to generalize the results that we observe in this study beyond Sweden, to other countries where life expectancy has been increasing. It should be added that an increase in the mean age at childbearing at the national level is not needed for our findings to be generalizable to other contexts, only that life expectancy must have been increasing. When life expectancy is increasing, children born in later calendar years will on average live longer; as a result, parents who give birth at older ages are likely to benefit their children in this regard. Although advanced maternal age is associated with involuntary childlessness, greater risk of spontaneous abortion, and an increased risk of poor perinatal outcomes (Cnattingius et al. Citation1992; Andersen et al. Citation2000), our study suggests that it is not associated with increased mortality in adulthood. We suggest that these results may also be generalizable to other high-income countries that have experienced steady increases in longevity.
Considering the consequences of these findings, it may be noted that they have implications for inequality in society more broadly. Indeed, it is possible that these secular improvements in health and longevity are contributing further to the diverging destinies of children with parents of high vs. low socio-economic status (McLanahan Citation2004). On average, higher maternal age is associated with higher socio-economic status. If we take two women born in 1950, one of whom comes from a low socio-economic status background and gives birth at age 20, and the other of whom comes from a high socio-economic status background and gives birth at age 40, even net of the difference in the socio-economic resources of these two women, the child of the mother with higher socio-economic status would be expected to live longer. In turn, that means that from a parental cohort perspective, the socio-economic disparities in the health and longevity of children are likely to be even greater than has previously been documented. Furthermore, since there are intergenerational correlations in the timing of childbearing (Kahn and Anderson Citation1992; Dahlberg Citation2013), this diverging pattern is likely to be cumulative over generations. Indeed, a particularly notable dimension of our results is the double burden of teenage childbearing. While teenage childbearing is associated with a wide range of negative outcomes for offspring (Brooks-Gunn and Furstenberg Citation1986), this is particularly true when taking a life course perspective and considering macro-level trends. Not only are the children of teenage parents disadvantaged for all the reasons well detailed in the previous literature, teenage parents are also giving birth at the point in their lives where their children are least likely to gain from secular improvements in longevity.
This study itself is not without limitations. Due to the nature of the Swedish Multigenerational Register and the Swedish Mortality Register, we study mortality over the period 1990–2012 for cohorts born 1938–60. This means that we observe mortality over different ages for different birth cohorts, and we do not observe mortality before age 30 or after age 74. Our results apply to this specific combination of birth cohorts and ages in this time period. While we would argue that the declines in age-adjusted all-cause and cause-specific mortality () make it clear that our findings are not limited to the cohorts and ages that we study, we are not able to evaluate our research question empirically outside the specific birth cohort, age, and period combination defined by our data structure. Most deaths occur after age 74 in contemporary Sweden, and so the relationship between parental age and offspring longevity may be found to differ once it is possible to study these birth cohorts to extinction. Indeed, previous research on survival to age 100 or older, where birth year was not controlled, has suggested that being born to a mother of advanced maternal age decreases the likelihood of becoming a centenarian, and being born to a teenage mother increases the likelihood of becoming a centenarian (Gavrilov and Gavrilova Citation2014, Citation2015; Jarry et al. Citation2014); however, that research was conducted using data on different cohorts and in different countries from the current study, and examined survival to different ages, so those findings should not necessarily be considered to contradict our results. In fact, most of the improvement in life expectancy in Sweden over the past few decades has been attributed to mortality improvements at older ages (Björck et al. Citation2009; La Vecchia et al. Citation2010; Modig et al. Citation2013), suggesting that it is at least plausible that the patterns we observe will also persist when studied at older ages.
A further important point is that many of our analyses are based on within-family sibling comparisons. The key strength of these stratified Cox models is that they adjust for all factors that are shared by siblings, including those that are unobserved and may otherwise be very difficult to measure, such as shared genetics or shared parenting style. However, as we describe in the ‘Data’ subsection, these models require the selection of families where at least one sibling died in the observation window that we use in our study (1990–2012). This in turn means that the size of our analytical sample for those sibling comparison models is much lower than that of the full population for those birth cohorts. Since mortality is generally low at adult ages in Sweden, this means that we likely select on a portion of the population that has worse health or has been exposed to greater environmental stressors, and it is not technically possible to generalize those results to the rest of the population. However, it is quite credible that this more vulnerable or frail portion of the population could be more susceptible than average to the negative effects of reproductive ageing associated with advanced parental age, and yet we still see that advanced paternal age is associated with lower mortality in this sibling population, and that advanced maternal age is not associated with significantly elevated mortality. Furthermore, when also conducting our analyses using data on the full population for the selected birth cohorts, we find qualitatively very similar results: delaying childbearing to older ages is associated with lower offspring mortality. Indeed, the results are even clearer in the analyses using the full cohort data.
Whether the positive effects of postponement on longevity will continue to be observed in the future depends on whether mortality continues to decline. While the pace of increase in the highest period life expectancies has slowed down, it continues to be positive (Vallin and Meslé Citation2009). Moreover, cohort life expectancies have increased even more rapidly than period-based measures (Shkolnikov et al. Citation2011) and the most recent trends show that period life expectancy continues to increase year-on-year (Mathers et al. Citation2015). This suggests that our finding that postponing childbearing increases longevity for offspring not only applies to the cohorts that we examine in this study, but also to cohorts born after 1960, including those born today. Although increases in life expectancy do not always translate directly into increases in healthy life expectancy, the latter has also been improving over time (Salomon et al. Citation2012), suggesting that delaying childbearing to older ages translates into real improvements in the life conditions of the offspring. Nevertheless, it is important to be clear that although mortality improvements in high-income countries are predicted for the foreseeable future, we cannot anticipate them with complete certainty.
Although we have considered primarily the benefits associated with childbearing at older ages, it is also important to reflect on potential disadvantages. From the perspective of parents, delaying childbearing to older ages will mean that lifespan overlap with their children will be shorter on average. While a desire to convey as much advantage as possible to children would be natural, this must also be counterbalanced with wanting to spend more of one's lifetime with one's children. Furthermore, as already mentioned, childbearing at older ages is associated with an increased risk of involuntary childlessness, higher rates of miscarriage, and an increased risk of poor birth outcomes (Cnattingius et al. Citation1992; Andersen et al. Citation2000). A further important point is that not all secular trends are positive. Although longevity has been increasing consistently over the past century, there have also been increases in inequality (Piketty Citation2014), which could negatively affect opportunities and life chances. Nevertheless, similar population-level improvements over time have also been observed in other domains, including education and cognitive ability (Myrskylä et al. Citation2013; Barclay and Myrskylä Citation2016a). Future research on advancing parental age would benefit from combining the traditional micro-perspective with the macro-perspective outlined in this study that acknowledges the potential benefits of being born at a later date.
Supplementary Material
Download PDF (217.6 KB)Notes
1 Please direct all correspondence to Kieron Barclay, Max Planck Institute for Demographic Research, Konrad-Zuse-Straße 1, 18057 Rostock, Germany; or by E-mail: [email protected]
2 Kieron Barclay and Mikko Myrskylä were supported by a European Research Council Starting Grant awarded to Mikko Myrskylä (COSTPOST: 336475).
References
- Abdalla, H., M. Wren, A. Thomas, and L. Korea. 1997. Age of the uterus does not affect pregnancy or implantation rates; a study of egg donation in women of different ages sharing oocytes from the same donor, Human Reproduction 12(4): 827–829. doi: 10.1093/humrep/12.4.827
- Allison, P. D. 2009. Fixed Effects Regression Models. Vol. 160. Thousand Oaks, CA: SAGE Publications.
- Allsopp, R. C., H. Vaziri, C. Patterson, S. Goldstein, E. V. Younglai, A. B. Futcher, C. W. Greider, and C. B. Harley. 1992. Telomere length predicts replicative capacity of human fibroblasts, Proceedings of the National Academy of Sciences 89(21): 10114–10118. doi: 10.1073/pnas.89.21.10114
- Andersen, A.-M. N., J. Wohlfahrt, P. Christens, J. Olsen, and M. Melbye. 2000. Maternal age and fetal loss: population based register linkage study, BMJ 320(7251): 1708–1712. doi: 10.1136/bmj.320.7251.1708
- Barclay, K. and M. Kolk. 2015. Birth order and mortality: a population-based cohort study, Demography 52(2): 613–639. doi: 10.1007/s13524-015-0377-2
- Barclay, K. and M. Myrskylä. 2014. Birth order and physical fitness in early adulthood: evidence from Swedish military conscription data, Social Science & Medicine 123: 141–148. doi: 10.1016/j.socscimed.2014.11.007
- Barclay, K. and M. Myrskylä. 2016a. Advanced maternal age and offspring outcomes: reproductive aging and counterbalancing period trends, Population and Development Review 42(1): 69–94. doi: 10.1111/j.1728-4457.2016.00105.x
- Barclay, K. and M. Myrskylä. 2016b. Linear effects of maternal age and period trends cannot be distinguished – Reply from Barclay and Myrskylä, Population and Development Review 42(4): 711. doi: 10.1111/padr.12025
- Barclay, K. and M. Myrskylä. 2017. Fertility postponement could reduce child mortality: evidence from 228 Demographic and Health Surveys covering 77 developing countries, MPIDR Working Paper WP–2017–005: 1–39.
- Barclay, K., K. Keenan, E. Grundy, M. Kolk, and M. Myrskylä. 2016. Reproductive history and post-reproductive mortality: a sibling comparison analysis using Swedish register data, Social Science & Medicine 155: 82–92. doi: 10.1016/j.socscimed.2016.02.043
- Benetos, A., K. Okuda, M. Lajemi, M. Kimura, F. Thomas, J. Skurnick, C. Labat, K. Bean, and A. Aviv. 2001. Telomere length as an indicator of biological aging: the gender effect and relation with pulse pressure and pulse wave velocity, Hypertension 37(2): 381–385. doi: 10.1161/01.HYP.37.2.381
- Björck, L., A. Rosengren, K. Bennett, G. Lappas, and S. Capewell. 2009. Modelling the decreasing coronary heart disease mortality in Sweden between 1986 and 2002, European Heart Journal 30: 1046–1056. doi: 10.1093/eurheartj/ehn554
- Breen, R. 2010. Educational expansion and social mobility in the 20th century, Social Forces 89(2): 365–388. doi: 10.1353/sof.2010.0076
- Brooks-Gunn, J. and F. F. Furstenberg Jr. 1986. The children of adolescent mothers: Physical, academic, and psychological outcomes, Developmental Review 6(3): 224–251. doi: 10.1016/0273-2297(86)90013-4
- Cawthon, R. M., K. R. Smith, E. O’Brien, A. Sivatchenko, and R. A. Kerber. 2003. Association between telomere length in blood and mortality in people aged 60 years or older, The Lancet 361(9355): 393–395. doi: 10.1016/S0140-6736(03)12384-7
- Christensen, K., G. Doblhammer, R. Rau, and J. W. Vaupel. 2009. Ageing populations: the challenges ahead, The Lancet 374(9696): 1196–1208. doi: 10.1016/S0140-6736(09)61460-4
- Cnattingius, S., M. R. Forman, H. W. Berendes, and L. Isotalo. 1992. Delayed childbearing and risk of adverse perinatal outcome: a population-based study, Journal of the American Medical Association 268(7): 886–890. doi: 10.1001/jama.1992.03490070068044
- Conde-Agudelo, A., A. Rosas-Bermúdez, and A. C. Kafury-Goeta. 2006. Birth spacing and risk of adverse perinatal outcomes: a meta-analysis, Journal of the American Medical Association 295(15): 1809–1823. doi: 10.1001/jama.295.15.1809
- Cox, D. R. 1972. Regression models and life-tables, Journal of the Royal Statistical Society, Series B 34(2): 187–220.
- Dahlberg, J. 2013. Family influence in fertility: a longitudinal analysis of sibling correlations in first birth risk and completed fertility among Swedish men and women, Demographic Research 29: 233–246. doi: 10.4054/DemRes.2013.29.9
- Doblhammer, G. and J. W. Vaupel. 2001. Lifespan depends on month of birth, Proceedings of the National Academy of Sciences 98(5): 2934–2939. doi: 10.1073/pnas.041431898
- Drefahl, S., A. Ahlbom, K. Modig, and K. A. O'Connor. 2014. Losing ground - Swedish life expectancy in a comparative perspective, PLOS ONE 9(2): e88357. doi: 10.1371/journal.pone.0088357
- Drefahl, S., H. Lundström, K. Modig, and A. Ahlbom. 2012. The era of centenarians: mortality of the oldest old in Sweden, Journal of Internal Medicine 272(1): 100–102. doi: 10.1111/j.1365-2796.2012.02518.x
- Eisenberg, D. T., M. G. Hayes, and C. W. Kuzawa. 2012. Delayed paternal age of reproduction in humans is associated with longer telomeres across two generations of descendants, Proceedings of the National Academy of Sciences 109(26): 10251–10256. doi: 10.1073/pnas.1202092109
- Flynn, J. R. 1987. Massive IQ gains in 14 nations: what IQ tests really measure, Psychological Bulletin 101(2): 171–191. doi: 10.1037/0033-2909.101.2.171
- Gavrilov, L. A. and N. S. Gavrilova. 2012. Biodemography of exceptional longevity: early-life and mid-life predictors of human longevity, Biodemography and Social Biology 58(1): 14–39. doi: 10.1080/19485565.2012.666121
- Gavrilov, L. A. and N. S. Gavrilova. 2014. Determinants of exceptional human longevity: new ideas and findings, Vienna Yearbook of Population Research 11: 295–323. doi: 10.1553/populationyearbook2013s295
- Gavrilov, L. A. and N. S. Gavrilova. 2015. New developments in the biodemography of aging and longevity, Gerontology 61(4): 364–371. doi: 10.1159/000369011
- Gillespie, D. O., A. F. Russell, and V. Lummaa. 2013. The effect of maternal age and reproductive history on offspring survival and lifetime reproduction in preindustrial humans, Evolution 67(7): 1964–1974. doi: 10.1111/evo.12078
- Hart, C. L. and G. Davey-Smith. 2003. Relation between number of siblings and adult mortality and stroke risk: 25 year follow up of men in the collaborative study, Journal of Epidemiology and Community Health 57: 385–391. doi: 10.1136/jech.57.5.385
- Hassold, T. and P. Hunt. 2001. To err (meiotically) is human: the genesis of human aneuploidy, Nature Reviews Genetics 2(4): 280–291. doi: 10.1038/35066065
- Hayward, M. D. and B. K. Gorman. 2004. The long arm of childhood: the influence of early-life social conditions on men’s mortality, Demography 41(1): 87–107. doi: 10.1353/dem.2004.0005
- Hoffman, S. D., E. M. Foster, and F. F. Furstenberg Jr. 1993. Re-evaluating the costs of teenage childbearing, Demography 30(1): 1–13. doi: 10.2307/2061859
- Hultman, C., S. Sandin, S. Levine, P. Lichtenstein, and A. Reichenberg. 2011. Advancing paternal age and risk of autism: new evidence from a population-based study and a meta-analysis of epidemiological studies, Molecular Psychiatry 16(12): 1203–1212. doi: 10.1038/mp.2010.121
- Human Fertility Database. Max Planck Institute for Demographic Research (Germany) and Vienna Institute of Demography (Austria). Available: www.humanfertility.org (data downloaded April 2016).
- Human Mortality Database. University of California, Berkeley (USA), and Max Planck Institute for Demographic Research (Germany). Available: www.mortality.org or www.humanmortality.de (data downloaded April 2016).
- James, W. H. 1987. The human sex ratio. Part 1: a review of the literature, Human Biology 59(5): 721–752.
- Janssen, F. and A. E. Kunst. 2004. ICD coding changes and discontinuities in trends in cause-specific mortality in six European countries, 1950–99, Bulletin of the World Health Organization 82(12): 904–913.
- Jarry, V., A. Gagnon, and R. Bourbeau. 2014. Maternal age, birth order and other early-life factors: a family-level approach to exploring exceptional survival, Vienna Yearbook of Population Research 11: 267–294. doi: 10.1553/populationyearbook2013s267
- Kahn, J. R. and K. E. Anderson. 1992. Intergenerational patterns of teenage fertility, Demography 29(1): 39–57. doi: 10.2307/2061362
- Kalil, A., R. Ryan, and M. Corey. 2012. Diverging destinies: maternal education and the developmental gradient in time with children, Demography 49(4): 1361–1383. doi: 10.1007/s13524-012-0129-5
- Kemkes-Grottenthaler, A. 2004. Parental effects on offspring longevity—evidence from 17th to 19th century reproductive histories, Annals of Human Biology 31(2): 139–158. doi: 10.1080/03014460410001663407
- Klepinger, D. H., S. Lundberg, and R. D. Plotnick. 1995. Adolescent fertility and the educational attainment of young women, Family Planning Perspectives 27: 23–28. doi: 10.2307/2135973
- Komlos, J. and B. E. Lauderdale. 2007. The mysterious trend in American heights in the 20th century, Annals of Human Biology 34(2): 206–215. doi: 10.1080/03014460601116803
- Kong, A., M. L. Frigge, G. Masson, S. Besenbacher, P. Sulem, G. Magnusson, S. A. Gudjonsson, A. Sigurdsson, A. Jonasdottir, A. Jonasdottir, W. S. W. Wong, G. Sigurdsson, G. B. Walters, S. Steinberg, H. Helgason, G. Thorleifsson, D. F. Gudbjartsson, A. Helgason, O. Th. Magnusson, U. Thorsteinsdottir, and K. Stefansson. 2012. Rate of de novo mutations and the importance of father’s age to disease risk, Nature 488(7412): 471–475. doi: 10.1038/nature11396
- La Vecchia, C., C. Bosetti, F. Lucchini, P. Bertuccio, E. Negri, P. Boyle, and F. Levi. 2010. Cancer mortality in Europe, 2000–2004, and an overview of trends since 1975, Annals of Oncology 21: 1323–1360. doi: 10.1093/annonc/mdp530
- Lappegård, T. 2000. New fertility trends in Norway, Demographic Research 2, 3. Available: https://www.demographic-research.org/volumes/vol2/3/default.htm doi: 10.4054/DemRes.2000.2.3
- Mackenbach, J. P., A. E. Kunst, A. E. J. M. Cavelaars, F. Groenhof, J. J. M. Geurts, and EU Working Group on Socioeconomic Inequalities in Health. 1997. Socioeconomic inequalities in morbidity and mortality in Western Europe, The Lancet 349: 1655–1659. doi: 10.1016/S0140-6736(96)07226-1
- Mare, R. D. 2016. Educational homogamy in two gilded ages: evidence from intergenerational social mobility data, The ANNALS of the American Academy of Political and Social Science 663(1): 117–139. doi: 10.1177/0002716215596967
- Margolis, R. and M. Myrskylä. 2011. A global perspective on happiness and fertility, Population and Development Review 37(1): 29–56. doi: 10.1111/j.1728-4457.2011.00389.x
- Mathers, C. D., G. A. Stevens, T. Boerma, R. A. White, and M. I. Tobias. 2015. Causes of international increases in older age life expectancy, The Lancet 385(9967): 540–548. doi: 10.1016/S0140-6736(14)60569-9
- McLanahan, S. 2004. Diverging destinies: how children are faring under the second demographic transition, Demography 41(4): 607–627. doi: 10.1353/dem.2004.0033
- McPherson, M., L. Smith-Lovin, and J. M. Cook. 2001. Birds of a feather: homophily in social networks, Annual Review of Sociology 27: 415–444. doi: 10.1146/annurev.soc.27.1.415
- Modig, K., T. Andersson, S. Drefahl, A. Ahlbom, and P. Whittaker. 2013. Age-specific trends in morbidity, mortality and case-fatality from cardiovascular disease, myocardial infarction and stroke in advanced age: evaluation in the Swedish population, PLOS ONE 8(5): e64928. doi: 10.1371/journal.pone.0064928
- Myrskylä, M. and A. Fenelon. 2012. Maternal age and offspring adult health: evidence from the health and retirement study, Demography 49(4): 1231–1257. doi: 10.1007/s13524-012-0132-x
- Myrskylä, M. and R. Margolis. 2014. Happiness: before and after the kids, Demography 51(5): 1843–1866. doi: 10.1007/s13524-014-0321-x
- Myrskylä, M., I. T. Elo, I. V. Kohler, and P. Martikainen. 2014. The association between advanced maternal and paternal ages and increased adult mortality is explained by early parental loss, Social Science & Medicine 119: 215–223. doi: 10.1016/j.socscimed.2014.06.008
- Myrskylä, M., K. Silventoinen, P. Tynelius, and F. Rasmussen. 2013. Is later better or worse? Association of advanced parental age with offspring cognitive ability among half a million young Swedish men, American Journal of Epidemiology 177(7): 649–655. doi: 10.1093/aje/kws237
- Oeppen, J. and J. W. Vaupel. 2002. Demography: enhanced: broken limits to life expectancy, Science 296(5570): 1029–1031. doi: 10.1126/science.1069675
- Osler, M., A. N. Andersen, P. Due, R. Lund, M. T. Damsgaard, and B. E. Holstein. 2003. Socioeconomic position in early life, birth weight, childhood cognitive function, and adult mortality. A longitudinal study of Danish men born in 1953, Journal of Epidemiology and Community Health 57(9): 681–686. doi: 10.1136/jech.57.9.681
- Piketty, T. 2014. Capital in The Twenty-First Century. Cambridge, MA: Harvard University Press.
- Powell, B., L. C. Steelman, and R. M. Carini. 2006. Advancing age, advantaged youth: parental age and the transmission of resources to children, Social Forces 84(3): 1359–1390. doi: 10.1353/sof.2006.0064
- Rau, R., E. Soroko, D. Jasilionis, and J. W. Vaupel. 2008. Continued reductions in mortality at advanced ages, Population and Development Review 34(4): 747–768. doi: 10.1111/j.1728-4457.2008.00249.x
- Richter, T., and T. von Zglinicki. 2007. A continuous correlation between oxidative stress and telomere shortening in fibroblasts, Experimental Gerontology 42(11): 1039–1042. doi: 10.1016/j.exger.2007.08.005
- Rocca, W., C. Van Duijn, D. Clayton, V. Chandra, L. Fratiglioni, A. Graves, A. Heyman, A. Jorm, E. Kokmen, K. Kondo, J. A. Mortimer, S. L. Shalat, H. Soininen, and A. Hofman for the Eurodem Risk Factors Research Group. 1991. Maternal age and Alzheimer’s Disease: a collaborative re-analysis of case-control studies, International Journal of Epidemiology 20(Supplement 2): S21–S27. doi: 10.1093/ije/20.Supplement_2.S21
- Salomon, J. A., H. Wang, M. K. Freeman, T. Vos, A. D. Flaxman, A. D. Lopez, and C. J. Murray. 2012. Healthy life expectancy for 187 countries, 1990–2010: a systematic analysis for the global burden disease study 2010, The Lancet 380(9859): 2144–2162. doi: 10.1016/S0140-6736(12)61690-0
- Salpea, K. D., P. J. Talmud, J. A. Cooper, C. G. Maubaret, J. W. Stephens, K. Abelak, and S. E. Humphries. 2010. Association of telomere length with type 2 diabetes, oxidative stress and UCP2 gene variation, Atherosclerosis 209(1): 42–50. doi: 10.1016/j.atherosclerosis.2009.09.070
- Shkolnikov, V. M., D. A. Jdanov, E. M. Andreev, and J. W. Vaupel. 2011. Steep increase in best-practice cohort life expectancy, Population and Development Review 37(3): 419–434. doi: 10.1111/j.1728-4457.2011.00428.x
- Sipos, A., F. Rasmussen, G. Harrison, P. Tynelius, G. Lewis, D. A. Leon, and D. Gunnell. 2004. Paternal age and schizophrenia: a population based cohort study, BMJ 329(7474): 1070. doi: 10.1136/bmj.38243.672396.55
- Smith, K. R., G. P. Mineau, G. Garibotti, and R. Kerber. 2009. Effects of childhood and middle-adulthood family conditions on later-life mortality: evidence from the Utah Population Database, 1850–2002, Social Science and Medicine 68: 1649–1658. doi: 10.1016/j.socscimed.2009.02.010
- Socialstyrelsen. 2007. Dödsorsaker 2005 [Causes of Death 2005]. Stockholm, Sweden: Socialstyrelsen.
- Socialstyrelsen. 2013. Dödsorsaker 2013 [Causes of Death 2013]. Stockholm, Sweden: Socialstyrelsen.
- Statistics Sweden. 2011. Multigeneration Register 2010: A Description of Contents and Quality. Stockholm: Statistics Sweden.
- Tatone, C., F. Amicarelli, M. C. Carbone, P. Monteleone, D. Caserta, R. Marci, P. G. Artini, P. Piomboni, and R. Focarelli. 2008. Cellular and molecular aspects of ovarian follicle ageing, Human Reproduction Update 14(2): 131–142. doi: 10.1093/humupd/dmm048
- Thomson, E., T. Lappegård, M. Carlson, A. Evans, and E. Gray. 2014. Childbearing across partnerships in Australia, the United States, Norway, and Sweden, Demography 51(2): 485–508. doi: 10.1007/s13524-013-0273-6
- Torssander, J. and R. Erikson. 2010. Stratification and mortality—a comparison of education, class, status, and income, European Sociological Review 26(4): 465–474. doi: 10.1093/esr/jcp034
- United Nations. 2014. World Fertility Report 2013: Fertility at the Extremes. New York, NY: United Nations, Department of Economic and Social Affairs, Population Division.
- Vallin, J. and F. Meslé. 2009. The segmented trend line of highest life expectancies, Population and Development Review 35(1): 159–187. doi: 10.1111/j.1728-4457.2009.00264.x
- Watson, J. D. 1972. Origin of concatemeric T7DNA, Nature New Biology 239(94): 197–201. doi: 10.1038/newbio239197a0
- Wilmoth, J. R. 2005. On the relationship between period and cohort mortality, Demographic Research 13: 231–280. doi: 10.4054/DemRes.2005.13.11
- Yip, B. H., Y. Pawitan, and K. Czene. 2006. Parental age and risk of childhood cancers: a population-based cohort study from Sweden, International Journal of Epidemiology 35(6): 1495–1503. doi: 10.1093/ije/dyl177