ABSTRACT
Neisseria meningitidis is a common cause of bacterial meningitis, often leading to permanent sequelae or death. N. meningitidis is classified into serogroups based on the composition of the bacterial capsular polysaccharide; the 6 major disease-causing serogroups are designated A, B, C, W, X, and Y. Four of the 6 disease-causing serogroups (A, C, Y, and W) can be effectively prevented with available quadrivalent capsular polysaccharide protein conjugate vaccines; however, capsular polysaccharide conjugate vaccines are not effective against meningococcal serogroup B (MnB). There is no vaccine available for serogroup X. The public health need for an effective serogroup B vaccine is evident, as MnB is the most common cause of meningococcal disease in the United States and is responsible for almost half of all cases in persons aged 17 to 22 years. In fact, serogroup B meningococci were responsible for the recent meningococcal disease outbreaks on college campuses. However, development of a suitable serogroup B vaccine has been challenging, as serogroup B polysaccharide-based vaccines were found to be poorly immunogenic. Vaccine development for MnB focused on identifying potential outer membrane protein targets that elicit broadly protective immune responses across strains from the vast number of proteins that exist on the bacterial surface. Human factor H binding protein (fHBP; also known as LP2086), a conserved surface-exposed bacterial lipoprotein, was identified as a promising vaccine candidate. Two recombinant protein-based serogroup B vaccines that contain fHBP have been successfully developed and licensed in the United States under an accelerated approval process: bivalent rLP2086 (MenB-FHbp; Trumenba®) and 4CMenB (MenB-4 C; Bexsero®). This review will focus on bivalent rLP2086 only, including vaccine components, mechanism of action, and potential coverage across serogroup B strains in the United States.
Introduction
The bacteria Neisseria meningitidis is a human pathogen that is transmitted through contact with respiratory droplets. Although transmission and colonization typically result in asymptomatic carriage of the bacterium in the upper respiratory tract of otherwise healthy individuals [Citation1–Citation6], N. meningitidis is capable of invading the human host, leading to bacteremia that can quickly become life-threatening invasive meningococcal disease (IMD) [Citation6,Citation7]. IMD most often presents as meningitis and/or septicemia, and less commonly pneumonia, septic arthritis, otitis media, and epiglottitis [Citation5,Citation8].
Meningococcal disease is potentially fatal within 24 h of onset, with an average case fatality rate ranging from 10% to 20%; mortality rates can be as high as 40% in patients with septicemia [Citation3,Citation9,Citation10]. Moreover, almost 20% of survivors will experience permanent significant clinical sequelae, including neurologic impairment, hearing loss, and limb amputation [Citation3,Citation5,Citation11–Citation15]. In the United States, meningococcal disease is most common among infants and children <5 years of age, adolescents and young adults aged 16–21 years, and adults aged ≥65 years [Citation2]. The rapid progression of disease and the long-term sequelae that result from infection emphasize the need for safe and effective preventive strategies.
Twelve serogroups of N. meningitidis have been identified based on the composition of the bacterial capsular polysaccharide [Citation3,Citation5], with serogroups A, B, C, W, X, and Y responsible for almost all of the cases of life-threatening, sporadic, and endemic meningococcal disease worldwide [Citation5,Citation8,Citation16–Citation18]. Until recently, approved meningococcal vaccines only protected against meningococcal strains caused by four of the six major disease-causing serogroups of N. meningitidis. Specifically, infection with serogroups A, C, W, and Y can be prevented using purified capsular polysaccharide or capsular polysaccharide protein conjugate vaccines [Citation2]. Routine immunization with a meningococcal ACWY conjugate vaccine is recommended in the United States for all persons aged 11–18 years and for persons considered at increased risk for exposure to N. meningitidis [Citation2].
In the United States, serogroups B, C, and Y are the most prevalent serogroups [Citation2,Citation19]. However, even prior to implementing ACWY recommendations in the United States, meningococcal disease caused by all serogroups had started to decline [Citation20]. At this time, the majority of outbreaks were related to serogroup C (i.e. 72 serogroup C outbreaks, versus nine serogroup Y and 17 serogroup B outbreaks, occurred from 1980 to 2002); after the availability of the ACWY vaccine, the majority of outbreaks in the United States were related to serogroup B (i.e. nine serogroup B outbreaks, versus five serogroup C outbreaks, occurred from 2008 to 2015) [Citation2,Citation19,Citation21–Citation30]. In fact, serogroup B (MnB) was the cause of recent meningococcal outbreaks observed at several US university campuses [Citation31]. Thus, MnB remains the most common cause of meningococcal disease in the United States [Citation32], accounting for approximately half of all meningococcal cases among persons aged 17–22 years [Citation29], emphasizing the public health need for an effective MnB vaccine in this population.
Utilizing the capsular polysaccharide as a vaccine target for serogroups A, C, W, and Y made the development of a vaccine against these serogroups relatively straightforward. Unfortunately, the MnB polysaccharide is composed of polysialic acid repeating units that are structurally similar to molecules found on human neuronal cells, and thus can be considered a human self-antigen. To overcome the poor functional immunogenicity of MnB capsular polysaccharide (and the potential safety concerns of autoimmunity), MnB vaccine development switched focus to surface-exposed proteins () [Citation7,Citation33]. Researchers would need to discover a MnB surface protein that is present in a majority of circulating MnB disease strains, have limited immunologic variability across diverse MnB isolates, and elicit the appropriate immune response (i.e. a bactericidal antibody response, as discussed in more detail below) ().
Figure 1. Serogroup B vaccine development. Serogroup B vaccine development has focused on surface proteins that elicit a sufficient immune response, are present in a majority of MnB disease strains, and have limited immunologic variability across diverse serogroup B strains. A conserved surface-exposed bacterial lipoprotein and human complement fHBP, also known as LP2086, was identified as a promising vaccine target.
fHBP: factor H binding protein; MnB: meningococcal serogroup B.
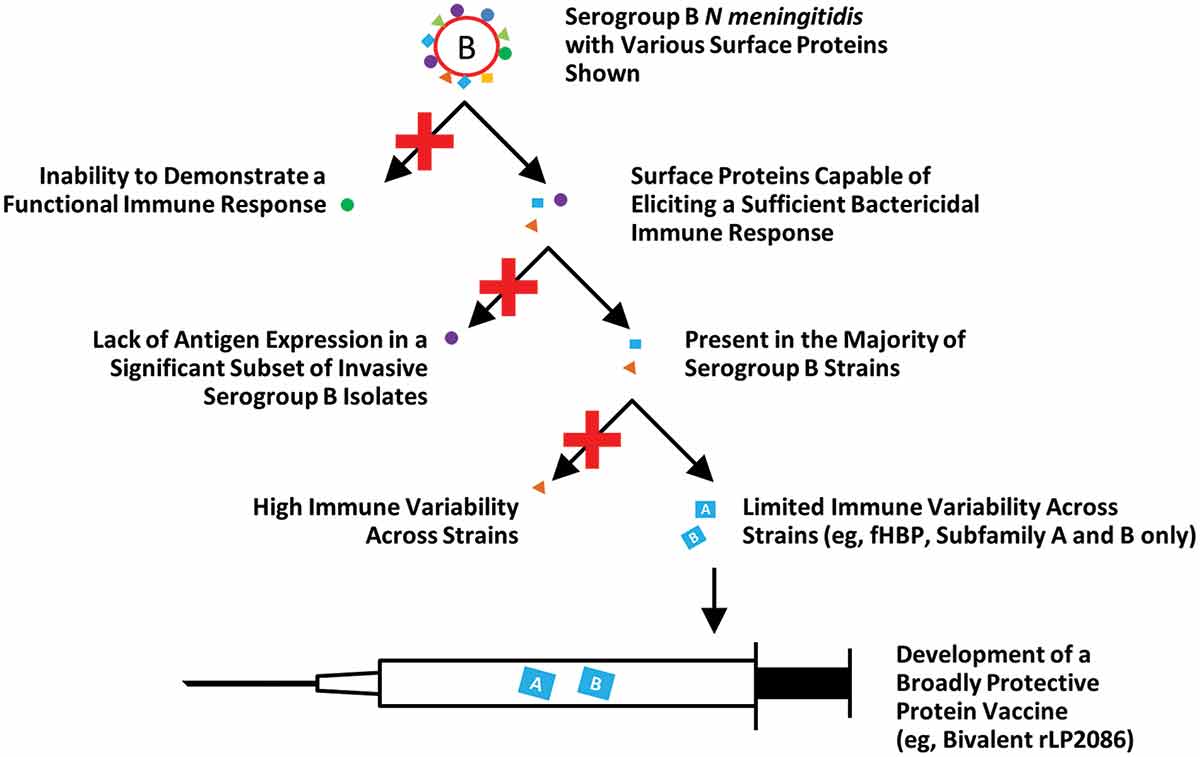
Protein-based outer membrane vesicle (OMV) MnB vaccines have been effectively used in response to outbreaks caused by specific epidemic MnB strains in Cuba, Norway, and New Zealand [Citation34]; these vaccines, produced from the specific strain causing the epidemic or outbreak, are composed of proteins that are shed by the bacterium in blebs from the outer membrane. The immune response to OMV vaccines is largely directed against the Porin A (PorA) protein expressed by the targeted MnB strain [Citation35]. Because there is extensive diversity in the PorA proteins expressed by different MnB strains, the ability of an OMV vaccine to protect against MnB is restricted to strains that express the same PorA protein variant [Citation35].
To counter the extensive variability of PorA between strains, candidate vaccines with multiple PorA variants have been pursued [Citation36]; however, even a vaccine with up to six PorA variants would likely provide limited coverage due to the diversity of PorA between MnB strains [Citation18]. Other surface proteins identified as potential vaccine targets include neisserial heparin-binding antigen (NHBA) and neisserial adhesin A (NadA) [Citation37,Citation38]. However, NHBA, although present in virtually all strains, is not always highly expressed, is genetically diverse, and functional immune responses as measured in serum bactericidal assay using human complement (hSBA), as described below, are hard to demonstrate [Citation39–Citation42]. The gene encoding NadA is not found in all strains, and when nadA is present, antigen expression is lacking in a significant subset of invasive isolates [Citation41,Citation43,Citation44].
A surface-exposed protein, known as factor H binding protein (fHBP), on the capsular polysaccharide was identified as a promising vaccine target by two different research groups [Citation45–Citation47]. fHBP is an ideal target because it is expressed on >97% of MnB strains. Furthermore, fHBP can be categorized into two immunologically distinct subfamilies, A and B [Citation45] (). Other groups also categorized fHBP into three major variants (i.e. 1, 2, and 3) [Citation47] or nine modular groups (i.e. I–IX) () [Citation48]. A publicly available database (http://pubmlst.org/neisseria/fHbp/) that allows all nomenclatures to be cross-referenced was also developed [Citation49]. Importantly for vaccine development, it should be noted that subfamily A and variant 2 (modular group III and VI) and 3 (modular groups II, V, VIII, and IX) are immunologically related, as they elicit protective antibodies across these groups, but do not cross-protect against subfamily B or variant 1 (modular groups I, IV, and VII) strains, and vice versa (). In addition, fHBP surface expression levels also vary between MnB strains and have been found to influence susceptibility in hSBA [Citation50,Citation51].
Figure 2. Meningococcal serogroup B factor H binding protein. MnB strains contain 1 of 2 distinct protein subfamilies of fHBP: subfamily A and subfamily B. Other groups also categorize fHBP into three variants (i.e. 1, 2, and 3) or 9 modular groups (i.e. I to IX).
fHBP: factor H binding protein; MnB: meningococcal serogroup B.
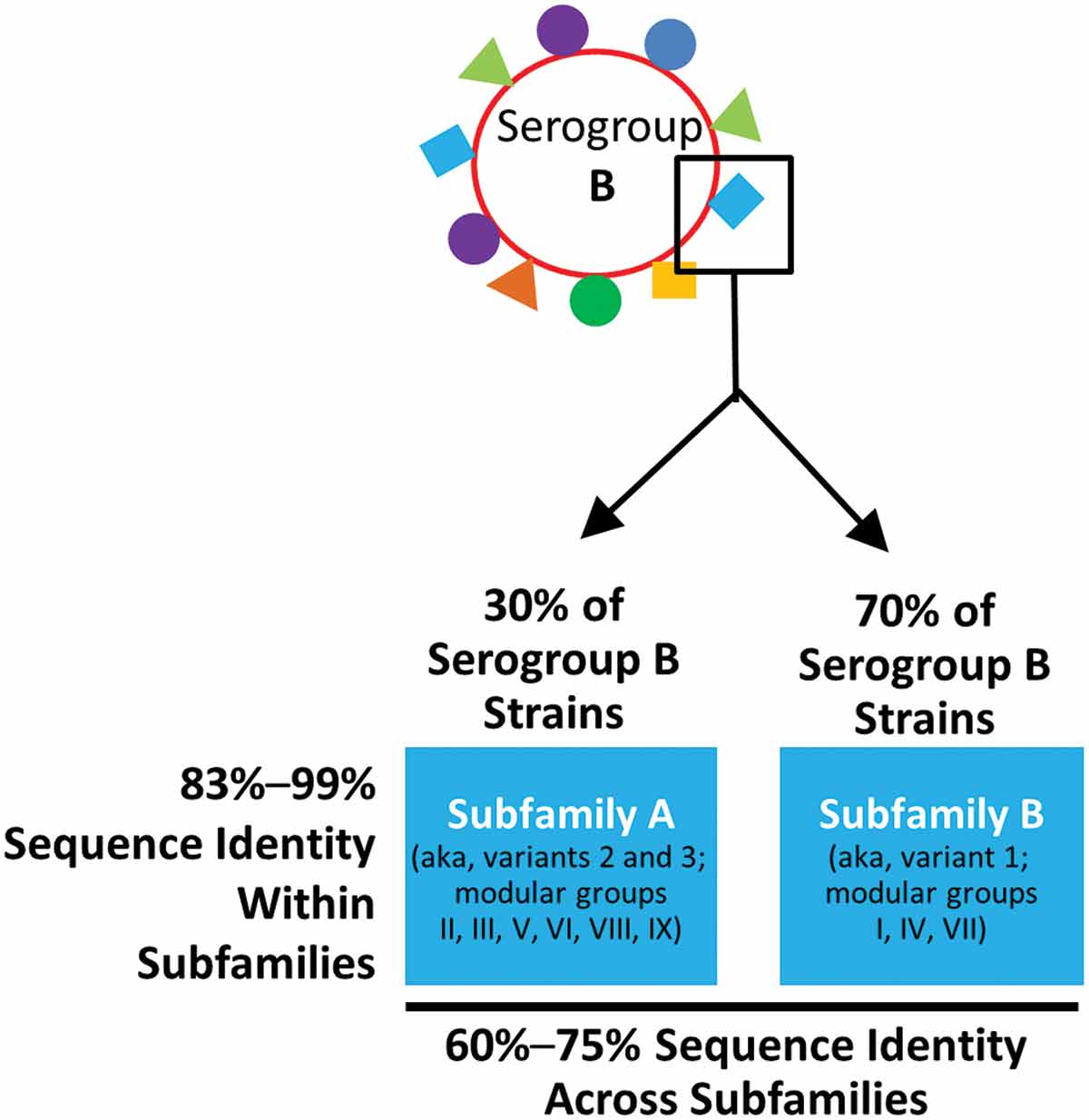
Two protein-based serogroup B vaccines that contain fHBP were developed: bivalent rLP2086 (Trumenba®; MenB-FHbp) and 4CMenB (Bexsero®; MenB-4C). These vaccines differ in their composition and approach to providing protection across diverse MnB strains. Specifically, bivalent rLP2086 is composed of an fHBP variant from each of the two distinct fHBP subfamilies (subfamily A and subfamily B): variant A05 and variant B01 (), discussed in more detail below. 4CMenB includes four unrelated meningococcal antigenic protein components (two as fusion proteins, GNA2091 fused to subfamily B fHBP and NHBA fused to GNA1030; NadA; and an OMV); none of these vaccine components cover the full antigenic diversity of the protein family from which they are derived. Through an accelerated regulatory process, bivalent rLP2086 was approved in the United States for the prevention of MnB disease in individuals 10 through 25 years of age [Citation52]. This was followed several months later by accelerated approval of 4CMenB, which is also licensed in Europe, Canada, Australia, and Brazil [Citation53–Citation57]. Due to the increased risk of MnB disease in adolescents, the Advisory Committee on Immunization Practices (ACIP) recommends that a MnB vaccine series of either bivalent rLP2086 or 4CMenB be considered for all adolescents and young adults aged 16–23 years to provide short-term protection against most strains causing serogroup B meningococcal disease; the preferred age for vaccination is 16–18 years [Citation58]. This recommendation is designed to protect adolescents and young adults during the period of increased risk (incidence of disease in the United States in adolescents/young adults peaks between 18 and 22 years of age). The ACIP also recommends vaccination with an MnB vaccine for high-risk persons aged 10 years and older [Citation31]. The Affordable Care Act and Vaccines for Children Program, which offers vaccines to children whose parents or guardians may not be able to afford them, cover both recommendations, as these programs ensure coverage for all vaccines administered in accordance with ACIP recommendations [Citation59,Citation60].
The goal of this review is to provide a general overview of bivalent rLP2086 for US physicians, and thus will focus primarily on bivalent rLP2086, with an emphasis on vaccine components, mechanism of action, and coverage across various serogroup B strains in the United States.
Vaccine components
MnB vaccine development focused on identifying a suitable antigen from outer membrane protein targets that exist on the bacterial surface (). The goal was to find a protein that elicited broad protective immune responses and was expressed by the majority of MnB disease causing strains. Once identified, these protein antigens were used as antigenic targets in recombinant protein vaccines.
A recombinant protein vaccine consists of purified protein antigen(s) that have been produced in large quantities using a heterologous expression system (i.e. bacteria or yeast genetically engineered to express high concentrations of a specific protein). The strategy of using recombinant proteins for vaccination is not new, as a recombinant protein vaccine using a yeast expression system targeting hepatitis B infection has been available in the United States since 1983 (Recombivax HB®, Merck & Co., Inc.) [Citation61]. More recently, another recombinant vaccine also using a yeast expression system was developed for protection against human papillomavirus (Gardasil®, Merck & Co., Inc) and is recommended for adolescents and young adults for the prevention of several diseases, including cervical, vulvar, vaginal, and anal cancers [Citation62,Citation63].
Bivalent rLP2086 is composed of two recombinant fHBP proteins. fHBP was identified as a potential vaccine target due to its ability to stimulate a strong antibody response capable of killing diverse serogroup B strains [Citation45]. The fHBP protein is utilized by meningococci to evade complement-mediated bactericidal activity [Citation64–Citation67] and is expressed in essentially all N. meningitidis serogroup B isolates [Citation46,Citation50,Citation68]. fHBP can be immunologically categorized into two distinct protein subfamilies, A and B, with 83–99% sequence identity within subfamilies and only 60–75% sequence identity between subfamilies () [Citation68]. Approximately 70% of invasive MnB isolates from the United States, Europe, New Zealand, and South Africa express fHBP subfamily B variants and 30% express subfamily A variants ().
The high sequence identity within subfamilies suggested that antibodies generated against any fHBP subfamily A protein variant would be effective against any serogroup B strain with a subfamily A fHBP; a similar response would be expected for antibodies elicited against subfamily B variants and strains expressing subfamily B fHBP. Preclinical studies confirmed that antibodies directed to fHBP are predominantly subfamily specific, and therefore supported a vaccine formulation composed of a protein from each fHBP subfamily. Data from early clinical studies further supported bivalent rLP2086 as broadly effective against MnB strains causing invasive disease [Citation45,Citation50,Citation69–Citation78].
Although the recombinant protein vaccine approach is not new, their use in preventing meningococcal disease is novel. Bivalent rLP2086 is a recombinant protein-based vaccine consisting of purified proteins separately produced by Escherichia coli genetically engineered to express high concentrations of subfamily A or B fHBP. With the exception of 4CMenB, protein expression using E coli has not been previously used for other recombinant protein vaccines. These recombinant proteins are then purified, stabilized [Citation51], and formulated for intramuscular injection [Citation52]. The recombinant fHBPs in bivalent rLP2086 contain a lipid tail, as does the native protein, which appears to increase immunogenicity [Citation45]. This is in contrast to the fHBP contained within 4CMenB, which is not lipidated. Importantly, bivalent rLP2086 shows consistency of immunologic response and safety and reactogenicity profile between studies [Citation45,Citation50,Citation69–Citation79] and is generally consistent with that observed for other meningococcal vaccines in adolescents [Citation80,Citation81].
Mechanism of action
One of the major functions of the innate immune system, which serves as the body’s first non-adaptive defense mechanism after infection, is activation of the complement cascade. Functioning mainly to eliminate pathogens, the complement cascade is composed of three main pathways (i.e. the common [or mannose-binding lectin] pathway, the classical pathway, and the alternative pathway) composed of more than 20 proteins. The importance of the complement cascade in clearance of meningococci from an infected individual is emphasized by the observation that individuals with defects in any of the complement activation pathways are at increased risk for IMD [Citation67,Citation82].
These three pathways are regulated by a series of proteins that activate or inactivate them in several places. For example, human factor H (hFH) is a regulatory protein that prevents activation of the alternative complement pathway to prevent unintended host damage. Many pathogens take advantage of these host regulators with some bacteria evolving methods to evade killing by binding proteins that inhibit the complement cascade to the bacterial surface [Citation83]. In the case of N. meningitidis, fHBP on the bacterial surface binds hFH thereby allowing the bacterium to evade the host’s innate immune system ()) [Citation64–Citation67]. Antibodies elicited by a vaccine such as bivalent rLP2086 block binding of hFH to fHBP; the bacterium will be rendered more susceptible to complement-mediated lysis ()) [Citation64]. As discussed in more detail below, bactericidal killing is the accepted surrogate of protection against meningococcal disease. Antibodies directed against fHBP potentially enhance bactericidal killing by simultaneously mediating killing through the classical pathway and amplifying the alternative pathway [Citation65]. Bound antibodies may also serve to target the bacterium for opsonization by phagocytes ()). However, it should be mentioned that some MnB isolates can recruit FH independent of fHBP [Citation84], which may allow these isolates to resist anti-fHBP complement-mediated bacteriolysis; further studies are necessary to confirm the implications of these variants on vaccine efficacy.
Figure 3. Role of factor H binding protein in immune response. (a) Meningococci recruit hFH to the cell surface; hFH binds fHBP, preventing complement from binding and allowing the bacterium to escape destruction by the innate immune system. (b) fHBP-specific antibodies, such as bivalent rLP2086 meningococcal B vaccine, prevent hFH from binding to fHBP, allowing complement to be deposited on the surface of the bacterium via direct binding to the pathogen (alternative pathway) or fixed to antibodies on the pathogen surface (classical pathway). Antibodies targeting fHBP can kill serogroup B meningococci via direct lysis or through phagocytosis.
fH: factor H; fHBP: factor H binding protein; hFH: human factor H.
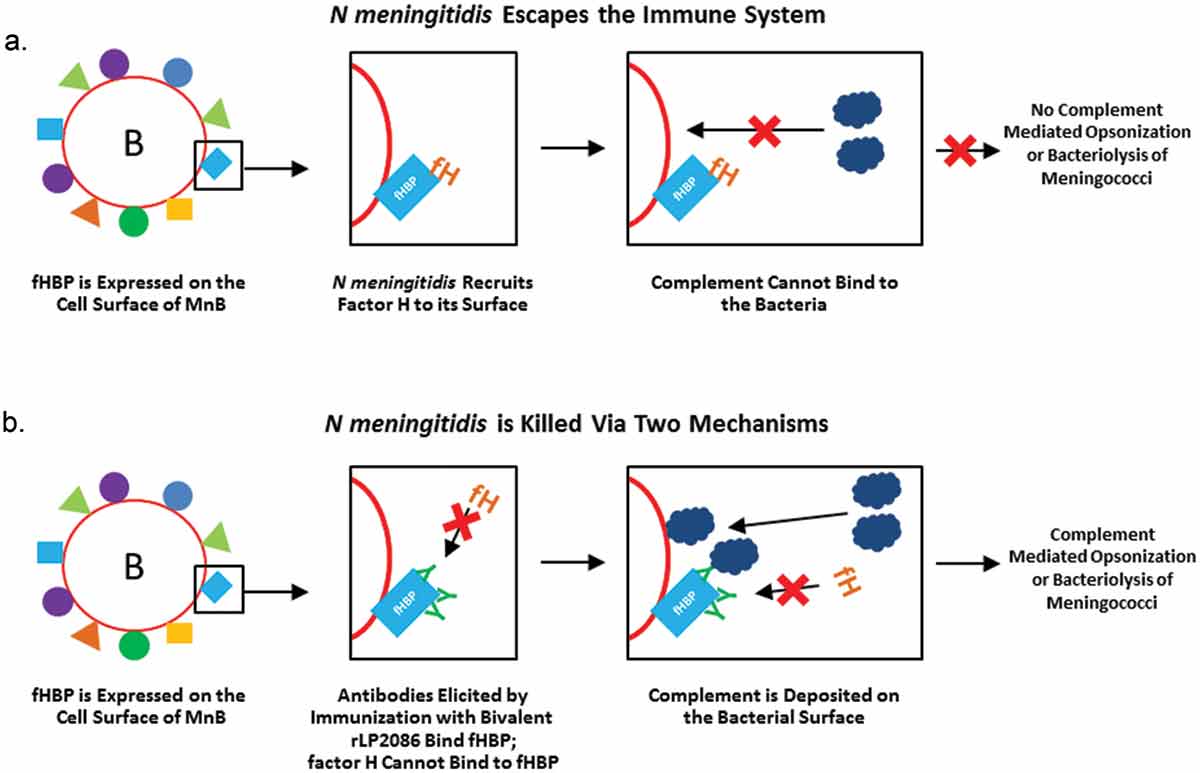
Coverage
Due to the relatively low incidence of meningococcal infection, largescale studies to directly determine the efficacy of a meningococcal vaccine would be logistically challenging; therefore, alternate end points that correlate with efficacy must be used to determine the potential of a MnB vaccine in a population [Citation85]. Because protection against IMD is achieved through the humoral immune system via the production of serum bactericidal antibodies to meningococcal capsular polysaccharides or to protein antigens, the activity of these bactericidal antibodies generated by the host to meningococcal vaccine antigens is used as a surrogate for efficacy. Complement-dependent bactericidal activity can be measured using hSBA () [Citation2,Citation86]. hSBA titer for a specific test strain is calculated by determining the highest serum dilution that kills ≥50% of the target bacteria in the assay.
Figure 4. Serum bactericidal assay with 4 different test strains of serogroup B N meningitidis used in bivalent rLP2086 studies.
fHBP: factor H binding protein; MnB: meningococcal serogroup B.
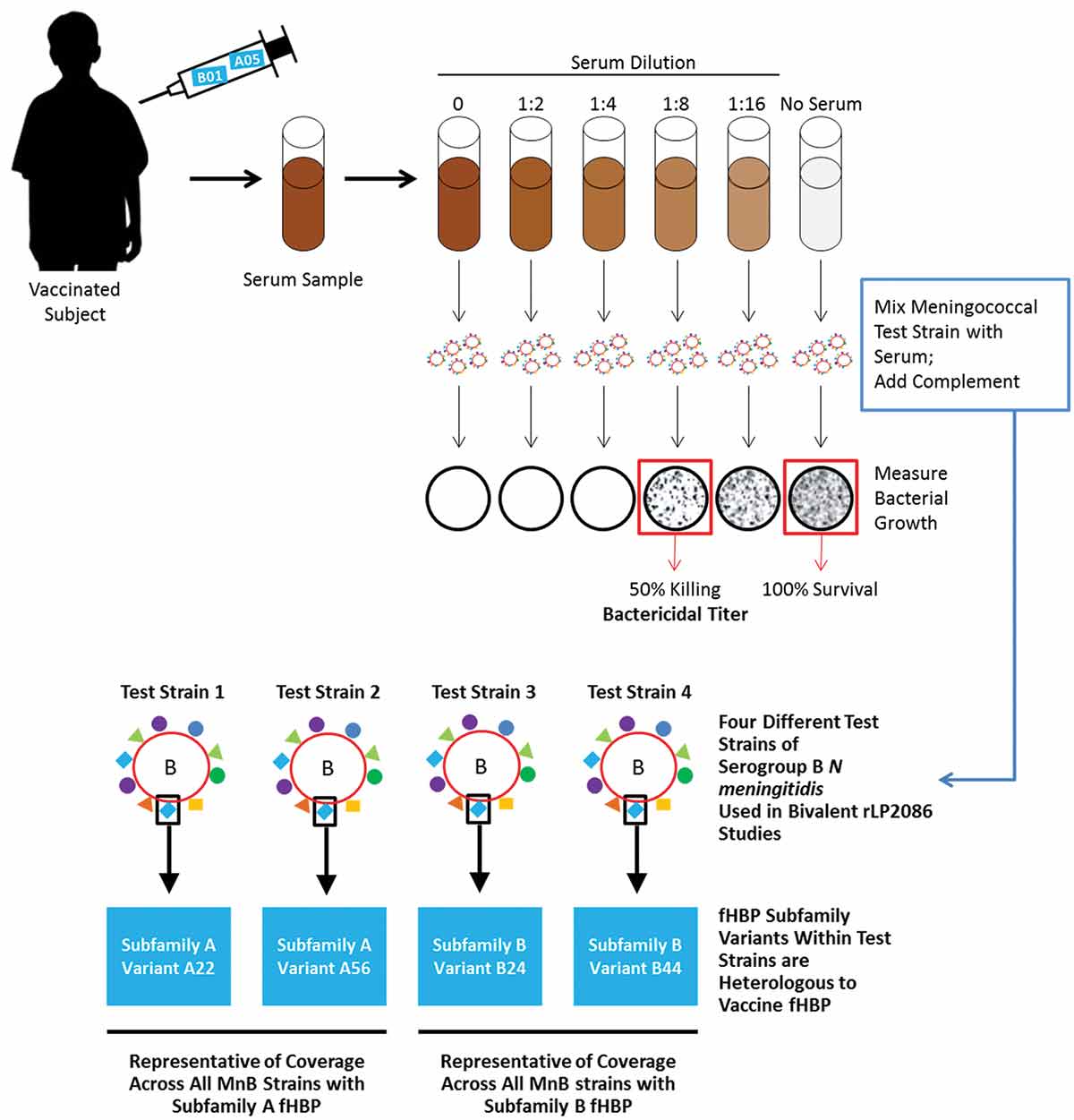
Based on seminal work completed by Goldschneider and colleagues in the late 1960s [Citation87], an hSBA titer of ≥1:4 is the accepted correlate of protection against meningococcal disease () [Citation87–Citation90]. Importantly, serogroup C conjugate vaccines were licensed on the basis of this surrogate end point, which correlated with effectiveness in postlicensure surveillance studies [Citation91]. SBA titers are currently accepted and used to demonstrate protection across meningococcal serogroups [Citation86,Citation89,Citation92]. Notably, licensure of bivalent rLP2086 was based on higher, more stringent hSBA titers of ≥1:8 or ≥1:16 for the test strains used, meaning even greater immune responses were required to meet study endpoints than the 1:4 titer that is accepted as the correlate of protection. As noted above, an hSBA assay can be also used preclinically to identify potential vaccine antigens, as those antigens that do not elicit a functional antibody response in hSBAs are unlikely to be an effective vaccine target.
For vaccines targeting the capsular polysaccharide, which have limited immunologic variability across strains within a specific serogroup, broad coverage can be assessed using a single test strain in SBA assays to represent all strains within that serogroup. However, evaluating broad coverage of a protein-based vaccine can be difficult because there are variations in the amino acid sequence of proteins expressed by MnB strains [Citation93,Citation94]. Importantly, no standardized hSBAs were available in support of a broadly protective MnB vaccine based on surface protein antigens that show sequence variability.
Thus, it was imperative to identify appropriate hSBA test strains for use in bivalent rLP2086 immunogenicity studies to accurately reflect vaccine coverage across the diverse MnB strains. Considering the limitations for the number of strains that could be tested in hSBA assays, 4 serogroup B strains representative of prevalent strains in the United States and elsewhere and reflecting the epidemiology of fHBPs expressed by MnB strains were selected for bivalent rLP2086 studies using an unbiased approach () [Citation51]. Each of the test strains expresses an fHBP variant that is heterologous (or different) from the fHBP variants in the vaccine and are representative of both rLP2086 subfamilies, A and B, and show low hSBA responses before vaccination (). As noted above, fHBP expression level is an important component in determining hSBA susceptibility. Thus, it should be noted that only strains within a low to medium level of fHBP surface expression were selected as this was representative of the strain pool collected; strains below a threshold were not predictably susceptible to killing in hSBAs [Citation50,Citation51]. Importantly, the vast majority of strains examined to date are above this expression threshold and are susceptible to killing in hSBAs [Citation51].
These test strains were used in hSBAs to demonstrate the generation of bactericidal antibodies against prevalent disease-causing MnB strains and representative of the diversity of fHBP. This approach supported the accelerated approval of bivalent rLP2086 in the United States for the prevention of MnB disease in individuals aged 10 through 25 years () [Citation31]. To supplement the immunogenicity data obtained from the four primary test strains and further support broad coverage of bivalent rLP2086, 10 additional test strains were selected and hSBAs developed to evaluate immune responses in two of the phase 3 clinical studies in adolescents and young adults [Citation78]. Data from these phase 3 studies confirmed those from earlier phase 1 and phase 2 () studies suggesting that antibodies generated by bivalent rLP2086 recognize strains across the diversity of fHBP subfamilies A and B [Citation45,Citation50,Citation69–Citation78]. Although surrogate methods may help predict the potential coverage elicited by vaccination with bivalent rLP2086, actual effectiveness cannot be determined until large-scale post-licensure surveillance data are available.
Conclusions and future considerations
MnB remains the predominant cause of meningococcal infection and disease burden in many regions, including the United States. While there are several conjugate polysaccharide vaccines available for the prevention of disease-causing serogroups A, C, W, and Y, the development of an effective MnB vaccine has been challenging. However, the discovery of LP2086 as a vaccine target, followed by the development of bivalent rLP2086, has provided the potential to extend prevention of IMD to include serogroup B.
As recommendations for the use of serogroup B vaccines are emerging, it is important to understand the potential benefits of vaccination in adolescents to determine the overall public health impact of vaccination. Importantly, adolescents are the primary carriers of meningococci [Citation1]. Because carriage is a prerequisite of transmission, prevention of carriage in adolescents through vaccination has the potential to result in prevention in both vaccinated (i.e. direct protection) and unvaccinated (i.e. indirect or herd protection) individuals. A phase 3, randomized study examining the effect of 4CMenB on carriage identified no significant difference between carriage of controls versus 4CMenB-vaccinated subjects for all disease-causing MnB isolates identified by PCR [Citation95]. Meningococcal carriage after dose 1 of bivalent rLP2086 was also evaluated in conjunction with its use to control an outbreak at a Rhode Island College in February 2015. Although this study suggested that bivalent rLP2086 vaccination had no apparent immediate effect on MnB carriage prevalence or acquisition 2 months after the first dose [Citation96], further data on bivalent rLP2086’s impact on carriage after 2 and 3 doses, as the vaccine is currently indicated, is needed. More importantly, whether either MnB vaccine can prevent carriage and acquisition in the context of a vaccination program, thereby decreasing transmission of serogroup B meningococci if broadly used, remains to be determined.
Although infants have some of the highest incidence rates of IMD in the United States, with the majority of cases being caused by MnB [Citation2,Citation97], routine vaccination of infants who are not at increased risk of IMD is not part of the national immunization program in the United States for any meningococcal serogroup. Moreover, neither bivalent rLP2086 nor 4CMenB are currently licensed for use in infants in the United States. Although it is beyond the scope of this review, it is important to acknowledge that 4CMenB is licensed for use in infants and has recently been included as part of the national immunization program in the United Kingdom [Citation98].
Education of those at risk for this disease, which includes adolescents/young adults as age is a risk factor, and their parents, physicians, policy makers, and the general public about the potential benefits of MnB vaccination is imperative, with the aim of improving vaccination coverage and decreasing meningococcal disease burden.
In the United States, serogroups B, C, and Y each account for about one-third of the IMD burden [Citation19]. Current recommendations in adolescents include recommendations of vaccines covering all three of these serogroups (i.e. ACIP recommends routine immunization with a meningococcal ACWY conjugate vaccine for all persons aged 11–18 years [Citation2] and that a MnB vaccine series be considered for all adolescents and young adults aged 16–23 years [Citation58]). By following these recommendations, we have the potential to maximize the prevention of both sporadic cases and outbreaks of meningococcal disease burden in the United States.
Table 1. Summary of phase 2 studies of bivalent rLP2086 meningococcal B vaccine in adolescents.
Declaration of interest
Editorial support has been provided by Nicole Gudleski O’Regan, PhD, of Complete Healthcare Communications, LLC, funded by Pfizer. A Gandhi, L York and P Balmer are employed by Pfizer Medical Development & Scientific Clinical Affairs. The authors have no other relevant affiliations or financial involvement with any organization or entity with a financial interest in or financial conflict with the subject matter or materials discussed in the manuscript apart from those disclosed.
Acknowledgments
The authors would like to thank Annaliesa Anderson, PhD, and Gregg C. Sylvester, MD, MPH, (Pfizer Inc) for critical reading of the manuscript.
References
- MacNeil JR, Cohn C. Chapter 8: meningococcal disease. In: VPD surveillance manual. 5th ed. Atlanta (GA): Centers for Disease Control and Prevention; 2011.
- Cohn AC, MacNeil JR, Clark TA, et al. Prevention and control of meningococcal disease: recommendations of the advisory committee on immunization practices (ACIP). MMWR Recomm Rep. 2013;62:1–28.
- Chang Q, Tzeng Y-L, Stephens DS. Meningococcal disease: changes in epidemiology and prevention. Clin Epidemiol. 2012;4:237–245.
- Burrage M, Robinson A, Borrow R, et al. Effect of vaccination with carrier protein on response to meningococcal C conjugate vaccines and value of different immunoassays as predictors of protection. Infect Immun. 2002;70:4946–4954.
- Rosenstein NE, Perkins BA, Stephens DS, et al. Meningococcal disease. N Engl J Med. 2001;344:1378–1388.
- Read RC. Neisseria meningitidis; clones, carriage, and disease. Clin Microbiol Infect. 2014;20:391–395.
- McIntosh ED, Carey V, Toneatto D, et al. Prevention of rare diseases: how revolutionary techniques can help vulnerable individuals-the example of serogroup B meningococcal infection. Ther Adv Vaccines. 2015;3:13–23.
- Stephens DS, Greenwood B, Brandtzaeg P. Epidemic meningitis, meningococcaemia, and Neisseria meningitidis. Lancet. 2007;369:2196–2210.
- Thompson MJ, Ninis N, Perera R, et al. Clinical recognition of meningococcal disease in children and adolescents. Lancet. 2006;367:397–403.
- Cohn AC, MacNeil JR, Harrison LH, et al. Changes in Neisseria meningitidis disease epidemiology in the United States, 1998–2007: implications for prevention of meningococcal disease. Clin Infect Dis. 2010;50:184–191.
- Kirsch EA, Barton RP, Kitchen L, et al. Pathophysiology, treatment and outcome of meningococcemia: a review and recent experience. Pediatr Infect Dis J. 1996;15:967–978; quiz 979.
- Centers for Disease Control and Prevention. Meningococcal disease: technical & clinical information [cited 2015 Jul 13]. Available from: http://www.cdc.gov/meningococcal/clinical-info.html
- Edwards MS, Baker CJ. Complications and sequelae of meningococcal infections in children. J Pediatr. 1981;99:540–545.
- Viner RM, Booy R, Johnson H, et al. Outcomes of invasive meningococcal serogroup B disease in children and adolescents (MOSAIC): a case-control study. Lancet Neurol. 2012;11:774–783.
- Roed C, Omland LH, Skinhoj P, et al. Educational achievement and economic self-sufficiency in adults after childhood bacterial meningitis. JAMA. 2013;309:1714–1721.
- Rouphael NG, Stephens DS. Neisseria meningitidis: biology, microbiology, and epidemiology. Methods Mol Biol. 2012;799:1–20.
- Halperin SA, Bettinger JA, Greenwood B, et al. The changing and dynamic epidemiology of meningococcal disease. Vaccine. 2012;30(suppl 2):B26–B36.
- Anderson AS, Jansen KU, Eiden J. New frontiers in meningococcal vaccines. Expert Rev Vaccines. 2011;10:617–634.
- Baccarini C, Ternouth A, Wieffer H, et al. The changing epidemiology of meningococcal disease in North America 1945–2010. Hum Vaccin Immunother. 2013;9:162–171.
- Centers for Disease Control and Prevention. Surveillance reports: Neisseria meningitidis (1993–2013) [cited 2016 Mar 17]. Available from: http://www.cdc.gov/abcs/reports-findings/surv-reports.html
- MacNeil J Epidemiology of serogroup B meningococcal disease, United States [cited 2015 Mar 27]. Available from: http://www.cdc.gov/vaccines/acip/meetings/downloads/slides-2014-10/mening-02-MacNeil.pdf
- Brooks R, Woods CW, Benjamin DK Jr., et al. Increased case-fatality rate associated with outbreaks of Neisseria meningitidis infection, compared with sporadic meningococcal disease, in the United States, 1994–2002. Clin Infect Dis. 2006;43:49–54.
- Weiss D, Antwi M, Del Rosso P, et al. Serogroup C invasive meningococcal disease among men who have sex with men — New York City, 2010–2012. MMWR Morb Mortal Wkly Rep. 2013;61:1033–1052.
- California Department of Public Health. Meningococcal vaccine recommendations for men who have sex with men [cited 2015 Mar 23]. Available from: http://www.cdph.ca.gov/HealthInfo/discond/Documents/MSM_meningococcal_vaccine_health_advisory_April15_2014.pdf
- Chicago Health Alert Network. Meningococcal vaccine recommendations for HIV-positive individuals [cited 2015 Sep]. Available from: https://www.chicagohan.org/mening;jsessionid=26A202153B93BCF32DCEBE782223277B
- Mandal S, Wu HM, MacNeil JR, et al. Prolonged university outbreak of meningococcal disease associated with a serogroup B strain rarely seen in the United States. Clin Infect Dis. 2013;57:344–348.
- Patel M. Serogroup B meningococcal disease in the US. Presented at: IDWeek; 2014 Oct 8–12; Philadelphia, PA.
- Oregon Health Authority. 2015 meningococcal update [cited 2015 Jun 9]. Available from: https://public.health.oregon.gov/DiseasesConditions/DiseasesAZ/MeningococcalDisease/Pages/Meningococcal-Update.aspx
- Soeters HM, McNamara LA, Whaley M, et al. Serogroup B meningococcal disease outbreak and carriage evaluation at a college – Rhode Island, 2015. MMWR Morb Mortal Wkly Rep. 2015;64:606–607.
- Schaffner W, Baker CJ, Bozof L, et al. Addressing the challenges of serogroup B meningococcal disease outbreaks on campuses: a report by the National Foundation for Infectious Diseases. Bethesda (MD): National Foundation for Infectious Diseases; May 2014.
- Folaranmi T, Rubin L, Martin SW, et al. Use of serogroup B meningococcal vaccines in persons aged ≥10 years at increased risk for serogroup B meningococcal disease: recommendations of the advisory committee on immunization practices, 2015. MMWR Morb Mortal Wkly Rep. 2015;64:608–612.
- Centers for Disease Control and Prevention. Active bacterial core surveillance (ABCs) report, emerging infections program network, Neisseria meningitidis, 2012 [cited 2016 March 31]. Available from: http://www.cdc.gov/abcs/reports-findings/survreports/mening12.pdf
- Anderson AS, Hao L, Jiang Q, et al. Potential impact of the bivalent rLP2086 vaccine on Neisseria meningitidis carriage and invasive serogroup B disease. Hum Vaccin Immunother. 2013;9:471–479.
- Granoff DM. Review of meningococcal group B vaccines. Clin Infect Dis. 2010;50(Suppl 2):S54–S65.
- Holst J, Martin D, Arnold R, et al. Properties and clinical performance of vaccines containing outer membrane vesicles from Neisseria meningitidis. Vaccine. 2009;27(Suppl 2):B3–B12.
- Cartwright K, Morris R, Rümke H, et al. Immunogenicity and reactogenicity in UK infants of a novel meningococcal vesicle vaccine containing multiple class 1 (PorA) outer membrane proteins. Vaccine. 1999;17:2612–2619.
- Comanducci M, Bambini S, Brunelli B, et al. NadA, a novel vaccine candidate of Neisseria meningitidis. J Exp Med. 2002;195:1445–1454.
- Welsch JA, Moe GR, Rossi R, et al. Antibody to genome-derived neisserial antigen 2132, a Neisseria meningitidis candidate vaccine, confers protection against bacteremia in the absence of complement-mediated bactericidal activity. J Infect Dis. 2003;188:1730–1740.
- Donnelly J, Medini D, Boccadifuoco G, et al. Qualitative and quantitative assessment of meningococcal antigens to evaluate the potential strain coverage of protein-based vaccines. Proc Natl Acad Sci U S A. 2010;107:19490–19495.
- Jacobsson S, Hedberg ST, Mölling P, et al. Prevalence and sequence variations of the genes encoding the five antigens included in the novel 5CVMB vaccine covering group B meningococcal disease. Vaccine. 2009;27:1579–1584.
- Rossi R, Beernink PT, Giuntini S, et al. Susceptibility of meningococcal strains responsible for two serogroup B outbreaks on U.S. university campuses to serum bactericidal activity elicited by the MenB-4C vaccine. Clin Vaccine Immunol. 2015 Dec;22(12):1227–1234.
- Jacobsson S, Thulin S, Mölling P, et al. Sequence constancies and variations in genes encoding three new meningococcal vaccine candidate antigens. Vaccine. 2006;24:2161–2168.
- Wang X, Cohn A, Comanducci M, et al. Prevalence and genetic diversity of candidate vaccine antigens among invasive Neisseria meningitidis isolates in the United States. Vaccine. 2011;29:4739–4744.
- Comanducci M, Bambini S, Caugant D, et al. NadA diversity and carriage in Neisseria meningitidis. Infect Immun. 2004;72:4217–4223.
- Fletcher LD, Bernfield L, Barniak V, et al. Vaccine potential of the Neisseria meningitidis 2086 lipoprotein. Infect Immun. 2004;72:2088–2100.
- McNeil LK, Murphy E, Zhao XJ, et al. Detection of LP2086 on the cell surface of Neisseria meningitidis and its accessibility in the presence of serogroup B capsular polysaccharide. Vaccine. 2009;27:3417–3421.
- Masignani V, Comanducci M, Giuliani MM, et al. Vaccination against Neisseria meningitidis using three variants of the lipoprotein GNA1870. J Exp Med. 2003;197:789–799.
- Pajon R, Beernink PT, Harrison LH, et al. Frequency of factor H-binding protein modular groups and susceptibility to cross-reactive bactericidal activity in invasive meningococcal isolates. Vaccine. 2010;28:2122–2129.
- Brehony C, Wilson DJ, Maiden MC. Variation of the factor H-binding protein of Neisseria meningitidis. Microbiology. 2009;155:4155–4169.
- Jiang H-Q, Hoiseth SK, Harris SL, et al. Broad vaccine coverage predicted for a bivalent recombinant factor H binding protein based vaccine to prevent serogroup B meningococcal disease. Vaccine. 2010;28:6086–6093.
- Zlotnick GW, Jones TR, Liberator P, et al. The discovery and development of a novel vaccine to protect against Neisseria meningitidis serogroup B disease. Hum Vaccin Immunother. 2015;11(1):5–13.
- TRUMENBA®. Full prescribing information [package insert]. Collegeville (PA): Pfizer, Inc.; 2014.
- Bexsero. Health Canada [cited 2014 Aug 19]. Available from: http://www.hc-sc.gc.ca/dhp-mps/prodpharma/sbd-smd/drug-med/sbd_smd_2014_bexsero_147275-eng.php
- Bexsero®. Summary of product characteristics. 4CMenB. Basel (Switzerland): Novartis; 2013.
- US Food and Drug Administration (FDA). Approval letter – Bexsero [cited 2015 Jun 8]. Available from: http://www.fda.gov/BiologicsBloodVaccines/Vaccines/ApprovedProducts/ucm431446.htm
- Australian Government Department of Health. Australian public assessment report for multi-component meningococcal B vaccine [cited 2014 Aug 19]. Available from: http://www.tga.gov.au/pdf/auspar/auspar-meningococcal-131031.pdf
- Leroy O, Giradie P, Yazdanpanah Y, et al. Hospital-acquired pneumonia: microbiological data and potential adequacy of antimicrobial regimens. Eur Respir J. 2002;20:432–439.
- MacNeil JR, Rubin L, Folaranmi T, et al. Use of serogroup B meningococcal vaccines in adolescents and young adults: recommendations of the advisory committee on immunization practices, 2015. MMWR Morb Mortal Wkly Rep. 2015;64:1171–1176.
- Centers for Disease Control and Prevention. Charter of the advisory committee on immunization practices [cited 2015 Nov 24]. Available from: http://www.cdc.gov/vaccines/acip/committee/charter.pdf
- Centers for Disease Control and Prevention. Vaccines for children (VFC) program [cited 2015 Nov 24]. Available from: http://www.cdc.gov/vaccines/programs/vfc/index.html
- Recombivax HB (Hepatitis B Vaccine, recombinant). Full prescribing information [package insert]. White House Station (NJ): Merck & Co., Inc.; 2014.
- Markowitz LE, Dunne EF, Chesson HW, et al. Human papillomavirus vaccination: recommendations of the advisory committee on immunization practices (ACIP). Morb Mortal Wkly Rep. 2014;63:1–30.
- Gardasil (Human Papillomavirus Quadrivalent [types 6, 11, 16, and 18] Vaccine, recombinant). Full prescribing information [package insert]. White House Station (NJ): Merck & Co., Inc.; 2011.
- Madico G, Welsch JA, Lewis LA, et al. The meningococcal vaccine candidate GNA1870 binds the complement regulatory protein factor H and enhances serum resistance. J Immunol. 2006;177:501–510.
- Giuntini S, Reason DC, Granoff DM. Complement-mediated bactericidal activity of anti-factor H binding protein monoclonal antibodies against the meningococcus relies upon blocking factor H binding. Infect Immun. 2011;79:3751–3759.
- Schneider MC, Exley RM, Chan H, et al. Functional significance of factor H binding to Neisseria meningitidis. J Immunol. 2006;176:7566–7575.
- Wright V, Hibberd M, Levin M. Genetic polymorphisms in host response to meningococcal infection: the role of susceptibility and severity genes. Vaccine. 2009;27:B90–B102.
- Murphy E, Andrew L, Lee K-L, et al. Sequence diversity of the factor H binding protein vaccine candidate in epidemiologically relevant strains of serogroup B Neisseria meningitidis. J Infect Dis. 2009;200:379–389.
- Marshall HS, Richmond PC, Nissen MD, et al. A phase 2 open-label safety and immunogenicity study of a meningococcal B bivalent rLP2086 vaccine in healthy adults. Vaccine. 2013;31:1569–1575.
- Nissen MD, Marshall HS, Richmond PC, et al. A randomized, controlled, phase 1/2 trial of a Neisseria meningitidis serogroup B bivalent rLP2086 vaccine in healthy children and adolescents. Pediatr Infect Dis J. 2012;32:364–371.
- Richmond PC, Marshall HS, Nissen MD, et al. Safety, immunogenicity, and tolerability of meningococcal serogroup B bivalent recombinant lipoprotein 2086 vaccine in healthy adolescents: a randomised, single-blind, placebo-controlled, phase 2 trial. Lancet Infect Dis. 2012;12:597–607.
- Richmond PC, Nissen MD, Marshall HS, et al. A bivalent Neisseria meningitidis recombinant lipidated factor H binding protein vaccine in young adults: results of a randomised, controlled, dose-escalation phase 1 trial. Vaccine. 2012;30:6163–6174.
- Marshall HS, Richmond PC, Beeslaar J, et al. Persistence of meningococcal serogroup B–specific responses following vaccination with bivalent rLP2086. Presented at: Meningitis Research Foundation (MRF) Conference; 2015 Nov 4–5; London, UK.
- Muse D, Christensen S, Bhuyan P, et al. A phase 2, randomized, active-controlled, observer-blinded study to assess the immunogenicity, tolerability, and safety of bivalent rLP2086, a meningococcal serogroup B vaccine, coadministered with tetanus, diphtheria and acellular pertussis vaccine and serogroup A, C, Y and W-135 meningococcal conjugate vaccine in healthy US adolescents. Pediatr Infect Dis J. 2016 Jun;35(6):673–682.
- Senders S, Bhuyan P, Jiang Q, et al. Immunogenicity, tolerability, and safety in adolescents of bivalent rLP2086, a meningococcal serogroup B vaccine, coadministered with quadrivalent human papilloma virus vaccine. Pediatr Infect Dis J. 2016;35:548–554.
- Vesikari T, Wysocki J, Beeslaar J, et al. Immunogenicity, safety, and tolerability of bivalent rLP2086 meningococcal group B vaccine administered concomitantly with diphtheria, tetanus, and acellular pertussis and inactivated poliomyelitis vaccines to healthy adolescents. J Pediatric Infect Dis Soc. 2016 Jun;5(2):180–187.
- Vesikari T, Østergaard L, Diez-Domingo J, et al. Meningococcal serogroup B bivalent rLP2086 vaccine elicits broad and robust serum bactericidal responses in healthy adolescents. J Pediatric Infect Dis Soc. 2016 Jun;5(2):152–160.
- Vesikari T, Senders S, Absalon J, et al. Immunogenicity and safety of bivalent rLP2086, a meningococcal serogroup B vaccine, in adolescents and young adults in 2 pivotal phase 3 trials. Presented at: Meningitis Research Foundation; 2015 Nov 4–5; London, UK.
- Ostergaard L, Lucksinger GH, Absalon J, et al. A phase 3, randomized, active-controlled study to assess the safety and tolerability of meningococcal serogroup B vaccine bivalent rLP2086 in healthy adolescents and young adults. Vaccine. 2016;34:1465–1471.
- O’Ryan M, Stoddard J, Toneatto D, et al. A multi-component meningococcal serogroup B vaccine (4CMenB): the clinical development program. Drugs. 2014;74:15–30.
- Halperin SA, Baine Y, Domachowske JB, et al. Comparison of the safety and immunogenicity of a novel quadrivalent meningococcal ACWY-tetanus toxoid conjugate vaccine and a marketed quadrivalent meningococcal ACWY-diphtheria toxoid conjugate vaccine in healthy individuals 10–25 years of age. J Pediatric Infect Dis Soc. 2014;3:33–42.
- Emonts M, Hazelzet JA, de Groot R, et al. Host genetic determinants of Neisseria meningitidis infections. Lancet Infect Dis. 2003;3:565–577.
- Lambris JD, Ricklin D, Geisbrecht BV. Complement evasion by human pathogens. Nat Rev Microbio. 2008;6:132–142.
- Giuntini S, Pajon R, Ram S, et al. Binding of complement factor H to PorB3 and NspA enhances resistance of Neisseria meningitidis to anti-factor H binding protein bactericidal activity. Infect Immun. 2015;83:1536–1545.
- Plotkin SA, Gilbert PB. Nomenclature for immune correlates of protection after vaccination. Clin Infect Dis. 2012;54:1615–1617.
- Frasch CE, Borrow R, Donnelly J. Bactericidal antibody is the immunologic surrogate of protection against meningococcal disease. Vaccine. 2009;27(suppl 2):B112–B116.
- Goldschneider I, Gotschlich EC, Artenstein MS. Human immunity to the meningococcus. I. The role of humoral antibodies. J Exp Med. 1969;129:1307–1326.
- Andrews N, Borrow R, Miller E. Validation of serological correlate of protection for meningococcal C conjugate vaccine by using efficacy estimates from postlicensure surveillance in England. Clin Diagn Lab Immunol. 2003;10:780–786.
- Borrow R, Balmer P, Miller E. Meningococcal surrogates of protection–serum bactericidal antibody activity. Vaccine. 2005;23:2222–2227.
- Ramsay M, Kaczmarski E, Rush M, et al. Changing patterns of case ascertainment and trends in meningococcal disease in England and Wales. Commun Dis Rep CDR Rev. 1997;7:R49–R54.
- Ramsay ME, Andrews N, Kaczmarski EB, et al. Efficacy of meningococcal serogroup C conjugate vaccine in teenagers and toddlers in England. Lancet. 2001;357:195–196.
- Borrow R, Carlone GM, Rosenstein N, et al. Neisseria meningitidis group B correlates of protection and assay standardization–international meeting report Emory University, Atlanta, Georgia, United States, 16–17 March 2005. Vaccine. 2006;24:5093–5107.
- Sacchi CT, Whitney AM, Popovic T, et al. Diversity and prevalence of PorA types in Neisseria meningitidis serogroup B in the United States, 1992–1998. J Infect Dis. 2000;182:1169–1176.
- Vipond C, Care R, Feavers IM. History of meningococcal vaccines and their serological correlates of protection. Vaccine. 2012;30(Suppl 2):B10–B17.
- Read RC, Baxter D, Chadwick DR, et al. Effect of a quadrivalent meningococcal ACWY glycoconjugate or a serogroup B meningococcal vaccine on meningococcal carriage: an observer-blind, phase 3 randomised clinical trial. Lancet. 2014;384(9960):2123–2131.
- Soeters HM, McNamara L, Whaley M, et al. Meningococcal carriage evaluation in response to a serogroup B meningococcal disease outbreak and mass vaccination campaign at a college – Rhode Island, 2015. Presented at: IDWeek; 2015 Oct 7–11; San Diego, CA.
- Centers for Disease Control and Prevention. Infant meningococcal vaccination: Advisory Committee on Immunization Practices (ACIP) recommendations and rationale. MMWR Morb Mortal Wkly Rep. 2013;62:52–54.
- Public Health England. Introduction of MenB immunisation for infants [cited 2016 May 31]. Available from: https://www.gov.uk/government/uploads/system/uploads/attachment_data/file/437906/150622_MenB_bipartite_letter.pdf