Abstract
Amyloidosis is a disease characterized by the deposition of protein fibrils. Cardiac involvement is a significant factor in determining prognosis. This study aimed to examine the clinical profile, outcomes, and long-term mortality rates in patients with transthyretin (ATTR) and amyloid light-chain (AL) amyloidosis. The retrospective cohort study included 94 patients with amyloidosis (69 with AL and 25 with ATTR amyloidosis) diagnosed between 2010 and 2022. The study involved multimodality imaging (ECG, echocardiography and cardiac magnetic resonance (CMR) data and survival analyses. Patients with ATTR amyloidosis were older and had a higher proportion of males compared to those with AL amyloidosis. Cardiac involvement was more prevalent in the ATTR group, including atrial fibrillation (AF), while pleural and pericardial effusion were more frequent in the AL group. Biomarkers such as NT-proBNP and troponin T were significantly elevated in both groups and were associated with all-cause mortality only in univariate analyses. CMR data, especially typical late gadolinium enhancement (LGE) was not associated with increased mortality, while pleural effusion and left atrial dilatation on echocardiography were identified as powerful predictors of mortality. In conclusion, both AL and ATTR amyloidosis exhibited poor outcomes. Cardiac involvement, particularly dilated left atrium and pleural effusion on echocardiography were associated with an increased risk of mortality, while typical LGE on CMR was not.
Introduction
Amyloidosis is a rare disease characterized by the extracellular deposition of misfolded proteins, which aggregate as fibrils, with the potential to affect multiple organ systems, including the heart [Citation1]. Mature fibrils have binding sites for Congo red, making biopsy material with positive amyloid visible in the microscope with polarized light after staining [Citation2,Citation3]. Amyloidosis is classified based on targeted organs, i.e. systemic or localized, and may be either acquired or hereditary. Furthermore, the determination of aggregating fibril proteins and precursor proteins is also mandatory for classification. Two major types of amyloidosis are AL (amyloid light chain), derived from immunoglobulins, and ATTR (amyloid transthyretin). Both forms can occur sporadically or be hereditary, although only a few AL patients are described as hereditary in the literature [Citation2]. ATTR amyloidosis was previously described as a very rare diagnosis. Recent research, however, shows that this form of amyloidosis, which often causes heart failure, is probably underdiagnosed. New studies indicate that up to 20% of patients over 80 years with heart failure may have ATTR amyloidosis, and autopsy studies have shown that up to 25% of the elderly have amyloid deposition in the heart [Citation4].
AL amyloidosis is a systemic disease and may affect all organs, most commonly the kidneys, followed by the heart, where up to 50–70% of AL amyloidosis patients have cardiac involvement [Citation4–6]. The ATTR type is more organ-specific, and the heart is one of the main targets, almost always affected, regardless of whether it is a hereditary or sporadic variant [Citation4,Citation7,Citation8]. Due to the pathophysiology of cardiac amyloidosis, this phenotype is classified as a restrictive/infiltrative cardiomyopathy and is one of the hypertrophic cardiomyopathies. Dilatation of the heart occurs in the late/advanced stages of the disease [Citation8].
Amyloidosis is diagnosed differently based on subgroups and organ involvement. Multiple organs can be affected at the same time and the heart and kidneys are often involved regardless subtype [Citation1]. Electrocardiogram (ECG) might show typical patterns of low-voltage QRS complexes, left ventricular (LV) hypertrophy [Citation9] or LV strain. When amyloidosis is clinically suspected, imaging modalities and biopsy are often used to verify and classify the disease [Citation4]. Cardiac amyloidosis can be diagnosed with a positive endomyocardial biopsy alone, often considered the gold standard. However, echocardiography and cardiac magnetic resonance (CMR) imaging are the initial imaging modalities that raise suspicion of cardiac amyloidosis [Citation10]. The use of endomyocardial biopsy is limited due to the invasive nature of the procedure, risks for complications, and the need for specialized training centers to perform it. Recently, radionuclide cardiac imaging and positron emission tomography (PET) have been increasingly used and are relatively reliable methods for diagnosing ATTR amyloidosis. However, new research has demonstrated promising results regarding PET detection of AL as well when it comes to cardiac involvement [Citation11,Citation12].
The prognosis for amyloidosis depends mainly on the subtype, how early the diagnosis is made, and whether the heart is affected, as heart involvement is one of the most important factors for poor outcomes [Citation13,Citation14]. Although overall awareness of amyloidosis has increased substantially, larger contemporary studies on clinical outcomes are scarce, particularly in Scandinavian countries. In this article, we describe the clinical profile, clinical outcomes, and long-term mortality rates in patients with ATTR and AL amyloidosis from a single center over a period of 13 years.
Methods
Patient diagnosis and selection criteria
This retrospective descriptive cohort study from Bergen enrolled patients from western Norway (the Western Norway Regional Health Authority and related health trusts) catchment area. We investigated patients diagnosed from 2010 to 2022. A total of 120 patients diagnosed with either AL or ATTR amyloidosis by the ICD-10 codes (E 85.X) in their medical records were identified. After carefully reviewing their clinical records and imaging modalities used, as well as a thorough review of the blood tests, a total of 26 patients did not meet inclusion criteria and were excluded ().
The diagnosis of both AL and ATTR amyloidosis was made after standardized recognized guidelines [Citation15]. Testing for wild type or hereditary type of ATTR was not performed as standard part of diagnostic work up. The primary date of diagnosis (study entry), demographics, clinical variables, and laboratory values were obtained and confirmed by the same investigator (AI) in the immediate vicinity of the date of diagnosis or during the same hospital stay. All ECGs and echocardiograms (SS) and CMR images (THL) were carefully assessed by two independent imaging specialists. Typical CMR findings are presented in .
Figure 2. CMR images of an 83-year-old male diagnosed with AL amyloidosis. The left image (Panel A – 4-chamber view) displays the typical distribution of Late Gadolinium Enhancement (LGE), with a prominent subendocardial involvement in the left ventricle and encompassing the walls of all cardiac chambers, including the interatrial septum. In the right image (Panel B), native T1 mapping acquisition (transverse plane) reveals an elevated measurement within the interventricular septum, registering at 1448 milliseconds. This value exceeds the recommended threshold, which should ideally be below 1371 milliseconds, their upper normal value is defined as mean + 2 standard deviations (SD).
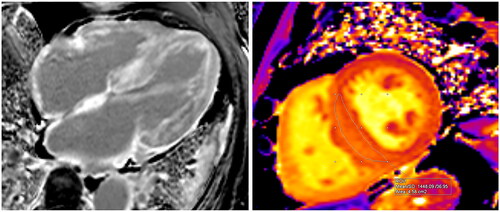
Standard 12-lead ECGs acquired in the supine position were carefully assessed with regard to voltage criteria and defined as either normal or low-voltage, both in frontal and limb leads (). Low voltage was defined as QRS voltage amplitude ≤5 mm (≤0.5 mV) in all limb leads, including both negative and positive components, and ≤1 mV in all precordial leads.
Figure 3. Electrocardiographic and echocardiography (apical 4-chamber view) images showing low voltage QRS (A) and huge left ventricular hypertrophy (B), arrow pointing towards interventricular septum) in a study participant with amyloidosis.
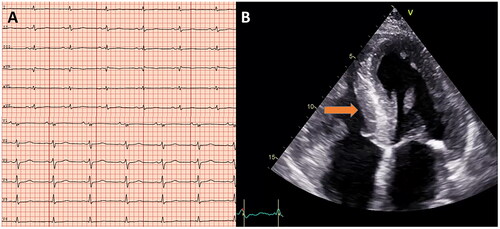
All echocardiograms were obtained with commercially available ultrasound machines following the same standard echocardiography protocol. Echocardiograms were retrospectively analysed on an Echopac workstation. LV structure (wall thickness and chamber dimensions) and function (LV ejection fraction and global longitudinal strain), and left atrium size (enlarged if left atrial volume index (LAVi) was ≥34 ml/m2) were measured. Right ventricular hypertrophy was defined as right ventricular free wall thickness >5 mm and LV hypertrophy as LV mass index >95 g/m2 in women and >115 g/m2 in men. Relative wall thickness was defined as 2× LV posterior wall thickness/LV internal diameter at end-diastole and considered high if >0.42 [Citation16]. Coronary artery disease (CAD) was defined as previously known myocardial infarction, percutaneous coronary intervention (PCI), coronary artery bypass graft (CABG), or angiographic evidence of significant obstructive disease in the epicardial coronary arteries.
Statistical analysis
IMB SPSS statistic, version 28 was used for all statistic. All continuous variables were tested for normal distribution (Shapiro–Wilk test) and presented as mean with standard deviation (SD) or median with interquartile range (IQR). Variables that are not normally distributed was log transformed to its natural logarithm (Ln) before cox regressions analysis. The independent t-test, if the data were normally distributed in each group was used to compare means, or its nonparametric equivalent (Mann-Whitney U test) was used to compare the distribution of two groups. Categorical variables are expressed as n (%) and were compared by Pearson Chi-squared and Fisher′s Exact tests when applicable. The survival analyses were performed both in the ATTR and AL patients. Kaplan-Meier curves were generated to calculate event-free survival rates in patients with and without atrial dilatation and atrial fibrillation, and the difference between groups was tested by Log-Rank test. Hazard ratios, both crude and adjusted for potential confounders, were estimated using Cox proportional hazards models. A two-sided p-value of <0.05 was considered statistically significant for all tests.
Endpoint of interest was all-cause mortality with censoring date 19 January 2023. Follow-up time was calculated from diagnosing point to death.
Approvement
The Regional Committee for Medical and Health Research (REK) classified the study as quality assurance project, without further need for ethical assessment (case number 327818/REK vest). The hospital data protection officer approved the study.
Results
Patients’ characteristics
The cohort included 94 patients (mean age 68.4 years, 69% males) with AL (n = 69, 73%) and ATTR (n = 25, 23%) amyloidosis. Patient characteristics are presented in . The clinical profiles of both phenotypes (AL vs. ATTR) amyloidosis were compared. Median age was significantly higher in the ATTR group compared with the AL group (79.4 years versus 64.4 years, p < 0.001) (). Systolic blood pressure was significantly higher in the same group as well (median 122 mmHg versus 138 mmHg, p < 0.001) (). In both groups, there was a greater proportion of men than women, respectively 64% versus 84% (p = 0.61). Cardiac involvement was observed in all patients with ATTR (100%), while this proportion was 68% in the AL group (p = 0.001). ECG findings were not significant between the groups (low voltage in 62% of AL and 60% of ATTR group, p = 0.838), while the prevalence of AF was nearly two-fold higher in the ATTR group (ATTR 68% versus AL 38%, p = 0.009). and Citation5 show correlations of interest from CMR, laboratory, and echocardiography data. The correlations are as expected, and most of them reached significance. Higher age at diagnosis correlates with higher levels of cardiac biomarkers in serum, and cardiac measurements are more deviated from reference values.
Figure 5. Correlation matrix. The figure give the correlation between the different parameters investigated, the values are given as Pearson correlation coefficient (PCC) and corresponding p-values. Negative PCC indicates a negative correlation, and positive PCC indicates positive correlation. Abbreviations: EF: LV ejection fraction (%) on CMR. GLS: global longitudinal strain on echocardiography.
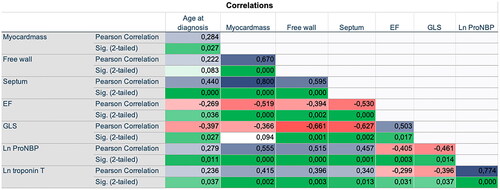
Table 1. Baseline characteristics and laboratory data.
Laboratory data
The main blood test data are presented in . Albumin was significantly different in the groups (AL 33.0 ± 8.6 g/L versus ATTR 40.1 ± 5.2 g/L, p < 0.001.) Also, total protein (g/L) showed the same findings with higher levels in the ATTR group. NT-pro-BNP was equally elevated in both groups. Among the immunoglobulins, the concentration of IgG was two-fold higher in the ATTR group compared with AL (AL 5.6 g/L versus ATTR 11.5 g/L, p= <0.001).
CMR findings
38 (55%) of the AL group and 24 (96%) of the ATTR group underwent a CMR. Typical LGE was present in 92% of ATTR patients and only about half of the AL group (p < 0.001). By contrast, atypical LGE was found in none of the ATTR patients but was evident in 11% of the AL patients (p = 0.287). Interventricular septum thickness, LV mass, LV mass index, and end-systolic volume were all significantly higher in the ATTR group than in AL (all p < 0.001) (). LV ejection fraction was significantly lower in the ATTR population (median 46.9 ± 10.1% versus 57.9 ± 11.1%, p < 0.001).
Table 2. Echocardiographic and cardiac magnetic resonance (CMR) imaging characteristics.
Echocardiographic findings
Echocardiographic findings of the patient population are presented in . 93 out of 94 (98.9%) patients had an echocardiogram performed. A higher proportion of the ATTR group had typical echocardiographic features of amyloidosis: biventricular hypertrophy (or LV hypertrophy), reduced global longitudinal strain with apical sparing "cherry on the top" pattern, representing preserved apical strain (21 [84%] versus 40 [62%], p = 0.041) than in the AL group. Left atrial dilatation tended to be more common in the ATTR compared with the AL group (80% versus 59% in the AL group, p = 0.056). LV end-diastolic dimension was indifferent between the two groups. Intraventricular septum thickness (mm) was significantly higher among ATTR patients than AL (19.1 ± 2.9 mm versus 15.6 ± 3.3 mm, p < 0.001). Likewise, posterior wall thickness was larger in ATTR patients (15.0 mm (12.5-18.0 mm) versus 13.0 mm (11.0-15.0 mm), p = 0.014). The difference in LV ejection fraction and global longitudinal strain by echocardiography was not significant between AL and ATTR patients.
Survival analysis
During a median follow-up of 27 (IQR 7–61) months, a total of 48 out of 94 patients (57% of AL group and 36% of ATTR, p = 0.079) died. Median survival for the AL group was 30 (IQR 5–74) months and for the ATTR group 24 (IQR 8.3–38.8) months from the initial diagnosis, which was not significantly different (p = 0.550). Similarly, no significant difference in survival was found between males and females (p = 0.930). Overall, amyloidosis patients with cardiac involvement had a median survival of 23 (IQR 7–48) months versus 51.5 (9.8–98.0) months in patients without cardiac involvement (p = 0.035). Median survival in patients with AF was 15 (IQR 3.0–38.3) months, and without AF 36 (IQR 9–73) months (p = 0.017). The Kaplan-Meier curves () show that the patient group with atrial dilations had comparable outcomes. Univariate and multivariate predictors of all-cause mortality are presented in . Pleural effusion was identified as a strong predictor of mortality (HR: 7.49; 95% CI: 3.64–15.14; p < 0.001), followed by AF, atrial dilatation, elevated NT-pro-BNP, and elevated troponin T (). The association between pericardial effusion and LGE on CMR did not reach statistical significance.
Figure 6. Kaplan–Meier curves of atrial fibrillations (upper) and atrial dilatation (lower), with log rank p values.
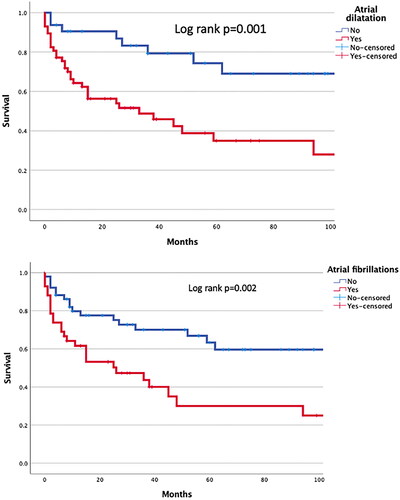
Table 3. Univariate and multivariate associations with all-cause mortality.
Discussion
Our data demonstrate several disparities and similarities between AL and ATTR amyloidosis. First, patients with ATTR amyloidosis are older, and often men, compared with patients of the AL group. These findings were somehow expected in view of previous reports [Citation17,Citation18]. When comparing AL with ATTR patients, we found important differences among the laboratory data. Albumin and total serum protein were more deviated from standard reference limits among AL patients. Also, immunoglobulins and immunoglobulin light chains had differences as expected, since AL-amyloidosis is a disease of immunoglobulins, and proteins are often abnormal [Citation19]. In both groups, cardiac biomarkers were elevated (NT-proBNP and troponin T), reflecting a higher extent of cardiac involvement in the groups and other findings like AF and signs of fibrosis [Citation20]. We did not expect the median NT-pro-BNP to be higher in the AL group than ATTR, because a smaller proportion of AL patients had known cardiac involvement (68 versus 100%). This implies that the AL patients tend to have higher levels of NT-pro-BNP than ATTR patients, supported by other studies [Citation21]. On the other hand, troponin T was higher in the ATTR group as expected, considering a larger proportion of myocardial damage in this subgroup. Cardiac mass and wall thickness are generally greater in ATTR amyloidosis, and cardiac hypertrophy is more prominent compared to AL amyloidosis. This shows that cardiac involvement presents differently based on the underlying subtype of amyloidosis. Some of these findings may be attributed to elevated blood pressure, a condition prevalent within the AL group.
shows that with increased myocardial mass on CMR, the levels of NT-proBNP in serum tended to increase (correlation coefficient 0.410). Of note, higher myocardial mass is a well-known marker of LV dysfunction and is associated with NT-proBNP [Citation22].
We found a strikingly high prevalence of AF, especially among ATTR patients (68%), which was almost 2-fold higher than the AL group. The prevalence of AF in amyloidosis patients has varied in other studies, ranging between 15 and 70% [Citation23,Citation24]. This large variation in the prevalence of AF may be explained by differences in the study population regarding age, comorbidities, and the methodology of detecting AF. In our patients, AF was associated with a poorer outcome. Other studies in the past looking at the general disease burden for amyloidosis patients with versus without AF also support these results [Citation25]. Jamal et al. found a 31% prevalence of AF in amyloidosis patients, and significantly higher rates of mortality and cardiac complications in patients coexisting with AF compared to patients without AF [Citation25].
In contrast, Donellan et al. [Citation26], studied the outcome of AF versus sinus rhythm in ATTR patients. During a mean follow-up of 35 months, they found no difference in mortality between patients with AF or without AF (65 vs. 49% mortality, p = 0.76), although mortality rates were numerically high in the AF group. AF increases with age and structural heart disease, and the patients in this study are older and, with cardiac involvement, they have some levels of structural heart disease [Citation25,Citation27], as well as left atrial dilation and diastolic dysfunction, as shown by our Kaplan-Meier curves. This is mainly because of the pathophysiology of AF, having a strong association with structural and functional remodeling [Citation28].
We also demonstrated that pleural effusion (16%) was a powerful predictor of mortality. Binder et al. had the same findings with a dismal outcome when the patients had pleural or pericardial effusions, with a significantly higher association with all causes of mortality with pleural effusion than pericardial effusion [Citation29]. Pleural effusion is known to be a sign of advanced disease, reflecting a poor outcome [Citation29,Citation30]. Most of the patients with pleural effusion among our patients had AL amyloidosis, implying that AL amyloidosis patients may be more prone to developing pleural effusion. Also, most of the patients with pericardial effusion had AL amyloidosis, but a quite high proportion of patients with ATTR amyloidosis had pericardial effusions as well, although the differences did not reach significance.
LV ejection fraction on echocardiography did not differ significantly, while LV ejection fraction on CMR, on the other hand, reached a significant difference. The ATTR group had a 5% lower LV ejection fraction when measured with CMR. Of note, CMR is considered the gold standard method and provides a more accurate measurement of LV ejection fraction, based upon 3D volume measurements of the heart [Citation31]. Another important difference observed was the extent of myocardial fibrosis on CMR. Although GLS by echocardiography correlates well with LGE from CMR [Citation32], it was fibrosis on CMR that was significantly different between the groups. Notably, 92% of patients with ATTR amyloidosis had typical LGE on CMR, compared with 53% in the AL group, while the difference in GLS on echocardiography was not statistically significant. Again, this indicates the value of CMR in clinical practice to evaluate accurate levels of extracellular amyloid deposition. Patients often undergo an echocardiogram earlier than CMR, as not all patients have access to CMR. This implies that echocardiography-based assessment of early myocardial involvement, both in AL and ATTR groups, may underestimate the burden of true subclinical LV dysfunction and myocardial fibrosis. This highlights the importance of referral to CMR for early diagnosis and treatment, which are crucial for better outcomes [Citation7].
Our study has some limitations. First, a single diagnostic gold standard, like endomyocardial biopsy, was not used in all patients to define cardiac involvement. Second, not all the patients who had echocardiograms also had CMR. In total, there was a small number of patients in both groups, especially in the ATTR group with only 25 patients, as the main source of recruitment was referrals from the medical ward in routine clinical care. Lastly, we did not investigate the effect of treatment in those in whom it was indicated, so the survival analysis does not differentiate between patients who received treatment and those who did not receive any treatment at all.
Conclusion
The findings are consistent with previous studies indicating that cardiac involvement in amyloidosis is highly prevalent and associated with an increased risk of all-cause mortality. Pleural effusion, AF, atrial dilatation, and elevated levels of NT-pro-BNP and troponin T were predictors of mortality. A thorough clinical, laboratory, and multimodality-imaging-based evaluation is important for risk stratifying patients with amyloidosis. CMR is a more robust method than echocardiography for the assessment of LV function, as well as typical and atypical myocardial fibrosis, both in patients with AL and ATTR amyloidosis.
Acknowledgment
The study received no funding.
Disclosure statement
No potential conflict of interest was reported by the author(s).
References
- Vaxman I, Gertz M. When to suspect a diagnosis of amyloidosis. Acta Haematol. 2020;143(4):304–311. doi: 10.1159/000506617.
- Picken MM. The pathology of amyloidosis in classification: a review. Acta Haematol. 2020;143(4):322–334. doi: 10.1159/000506696.
- Tanskanen M, Peuralinna T, Polvikoski T, et al. Senile systemic amyloidosis affects 25% of the very aged and associates with genetic variation in alpha2-macroglobulin and tau: a population-based autopsy study. Ann Med. 2008;40(3):232–239. doi: 10.1080/07853890701842988.
- Martinez-Naharro A, Baksi AJ, Hawkins PN, et al. Diagnostic imaging of cardiac amyloidosis. Nat Rev Cardiol. 2020;17(7):413–426. doi: 10.1038/s41569-020-0334-7.
- Merlini G. CyBorD: stellar response rates in AL amyloidosis. Blood. 2012;119(19):4343–4345. doi: 10.1182/blood-2012-03-413112.
- Falk RH, Alexander KM, Liao R, et al. AL (light-chain) cardiac amyloidosis: a review of diagnosis and therapy. J Am Coll Cardiol. 2016;68(12):1323–1341. doi: 10.1016/j.jacc.2016.06.053.
- Ioannou A, Patel RK, Razvi Y, et al. Impact of earlier diagnosis in cardiac ATTR amyloidosis Over the course of 20 years. Circulation. 2022;146(22):1657–1670. doi: 10.1161/CIRCULATIONAHA.122.060852.
- Jurcuţ R, Onciul S, Adam R, et al. Multimodality imaging in cardiac amyloidosis: a primer for cardiologists. Eur Heart J Cardiovasc Imaging. 2020;21(8):833–844. doi: 10.1093/ehjci/jeaa063.
- Sperry BW, Vranian MN, Hachamovitch R, et al. Are classic predictors of voltage valid in cardiac amyloidosis? A contemporary analysis of electrocardiographic findings. Int J Cardiol. 2016;214:477–481. doi: 10.1016/j.ijcard.2016.04.030.
- Gillmore JD, Maurer MS, Falk RH, et al. Nonbiopsy diagnosis of cardiac transthyretin amyloidosis. Circulation. 2016;133(24):2404–2412. doi: 10.1161/CIRCULATIONAHA.116.021612.
- Elliott PM, Anastasakis A, Authors/Task Force m., et al. 2014 ESC guidelines on diagnosis and management of hypertrophic cardiomyopathy: the task force for the diagnosis and management of hypertrophic cardiomyopathy of the european society of cardiology (ESC). Eur Heart J. 2014;35:2733–2779.
- Paeng JC, Choi JY. Nuclear imaging for cardiac amyloidosis: bone scan, SPECT/CT, and amyloid-targeting PET. Nucl Med Mol Imaging. 2021;55(2):61–70. doi: 10.1007/s13139-020-00681-4.
- Singh A, Musa TA, Treibel TA, et al. Sex differences in left ventricular remodelling, myocardial fibrosis and mortality after aortic valve replacement. Heart. 2019;105(23):1818–1824. doi: 10.1136/heartjnl-2019-314987.
- Cohen OC, Sathyanath A, Petrie A, et al. Prognostic importance of the 6 min walk test in light chain (AL) amyloidosis. Heart. 2022;108(20):1616–1622. doi: 10.1136/heartjnl-2021-320703.
- Gillmore JD, Wechalekar A, Bird J, et al. Guidelines on the diagnosis and investigation of AL amyloidosis. Br J Haematol. 2015;168(2):207–218. doi: 10.1111/bjh.13156.
- Lang RM, Badano LP, Mor-Avi V, et al. Recommendations for cardiac chamber quantification by echocardiography in adults: an update from the American society of echocardiography and the european association of cardiovascular imaging. J Am Soc Echocardiogr. 2015;28(1):1–39 e14. doi: 10.1016/j.echo.2014.10.003.
- González-López E, Gagliardi C, Dominguez F, et al. Clinical characteristics of wild-type transthyretin cardiac amyloidosis: disproving myths. Eur Heart J. 2017;38(24):1895–1904. doi: 10.1093/eurheartj/ehx043.
- Basset M, Milani P, Ferretti VV, et al. Prospective urinary albumin/creatinine ratio for diagnosis, staging, and organ response assessment in renal AL amyloidosis: results from a large cohort of patients. Clin Chem Lab Med. 2022;60(3):386–393. doi: 10.1515/cclm-2021-0912.
- Ryšavá R. AL amyloidosis: advances in diagnostics and treatment. Nephrol Dial Transplant. 2019;34(9):1460–1466. doi: 10.1093/ndt/gfy291.
- Nicol M, Baudet M, Brun S, et al. Diagnostic score of cardiac involvement in AL amyloidosis. Eur Heart J Cardiovasc Imag. 2020;21(5):542–548. doi: 10.1093/ehjci/jez180.
- Lehrke S, Steen H, Kristen AV, et al. Serum levels of NT-proBNP as surrogate for cardiac amyloid burden: new evidence from gadolinium-enhanced cardiac magnetic resonance imaging in patients with amyloidosis. Amyloid. 2009;16(4):187–195. doi: 10.3109/13506120903421538.
- de Simone G, Gottdiener JS, Chinali M, et al. Left ventricular mass predicts heart failure not related to previous myocardial infarction: the cardiovascular health study. Eur Heart J. 2008;29(6):741–747. doi: 10.1093/eurheartj/ehm605.
- Griffin JM, Rosenthal JL, Grodin JL, et al. ATTR amyloidosis: current and emerging management strategies: JACC: cardiooncology state-of-the-art review. JACC CardioOncol. 2021;3(4):488–505. doi: 10.1016/j.jaccao.2021.06.006.
- Pour-Ghaz I, Bath A, Kayali S, et al. A review of cardiac amyloidosis: presentation, diagnosis, and treatment. Curr Probl Cardiol. 2022;47(12):101366. doi: 10.1016/j.cpcardiol.2022.101366.
- Jamal S, Kichloo A, Bailey B, et al. Clinical outcomes and disease burden in amyloidosis patients with and without atrial fibrillation-insight from the national inpatient sample database. J Innov Card Rhythm Manag. 2021;12(6):4542–4549. doi: 10.19102/icrm.2021.120605.
- Donnellan E, Wazni OM, Hanna M, et al. Atrial fibrillation in transthyretin cardiac amyloidosis: predictors, prevalence, and efficacy of rhythm control strategies. JACC Clin Electrophysiol. 2020;6(9):1118–1127. doi: 10.1016/j.jacep.2020.04.019.
- Heeringa J, van der Kuip DA, Hofman A, et al. Prevalence, incidence and lifetime risk of atrial fibrillation: the Rotterdam study. Eur Heart J. 2006;27(8):949–953. doi: 10.1093/eurheartj/ehi825.
- Xiao HB, Rizvi SA, McCrea D, et al. The association of chronic atrial fibrillation with right atrial dilatation and left ventricular dysfunction in the elderly. Med Sci Monit. 2004;10(9):CR516–20.
- Binder C, Duca F, Binder T, et al. Prognostic implications of pericardial and pleural effusion in patients with cardiac amyloidosis. Clin Res Cardiol. 2021;110(4):532–543. doi: 10.1007/s00392-020-01698-7.
- Berk JL, Keane J, Seldin DC, et al. Persistent pleural effusions in primary systemic amyloidosis: etiology and prognosis. Chest. 2003;124(3):969–977. doi: 10.1378/chest.124.3.969.
- Rigolli M, Anandabaskaran S, Christiansen JP, et al. Bias associated with left ventricular quantification by multimodality imaging: a systematic review and meta-analysis. Open Heart. 2016;3(1):e000388. doi: 10.1136/openhrt-2015-000388.
- Fujimiya T, Iwai-Takano M, Igarashi T, et al. Late gadolinium enhancement predicts improvement in global longitudinal strain after aortic valve replacement in aortic stenosis. Sci Rep. 2019;9(1):15688. doi: 10.1038/s41598-019-51930-2.