Abstract
Iron (Fe) deficiency primarily damages photosystems. A large proportion of the reducing equivalents in mesophyll cells are dependent on the photosynthetic apparatus in the chloroplasts; thus, Fe deficiency profoundly affects nitrogen (N) and sulfur (S) assimilation. Microarray data suggested that nutrient metabolisms concerning the assimilation and re-utilization of essential elements were modulated in barley under Fe-deficient condition. We compared the concentrations of the major essential elements between Fe-deficient barley leaves and Fe-deficient rice leaves. The Fe-deficient barley and rice plants had the same Fe and chlorophyll concentrations, but growth was reduced more in Fe-deficient rice than in Fe-deficient barley. The accumulation of ribulose-1,5-bisphosphate carboxylase/oxygenase protein and nitrite reductase, sulfite reductase, and ferredoxin-dependent glutamate synthase mRNAs was reasonably decreased in the chlorotic young leaves of Fe-deficient barley. ,
,
,
, and Ca2+ concentrations were altered to a larger extent in Fe-deficient rice leaves than in Fe-deficient barley leaves, even in the non-chlorotic old leaves. In this paper, we discuss the adaptation of macronutrient metabolism to Fe deficiency in barley leaves.
Introduction
Deficiency of iron (Fe)—an essential plant nutrient—results in a remarkable decrease in yield. Iron-deficient plants display visual symptoms as well. Thus, a great deal of effort is being directed toward solving the problem of Fe deficiency in plants (Marschner Citation1995), and investigating ways to increase Fe acquisition has been a central theme. To date, Fe acquisition and transport mechanisms have been explained thoroughly at the molecular biological level (Jeong and Guerinot Citation2009). Several attempts to reinforce Fe acquisition have successfully produced Fe deficiency-tolerant transgenic plants (Takahashi et al. Citation2001; Ishimaru et al. Citation2007; Ogo et al. Citation2007). However, Fe concentration is not positively correlated with Fe-deficiency tolerance. We have previously reported that Fe deficiency-tolerant barley had low Fe concentration and that Fe deficiency-sensitive rice had high Fe concentration (Maruyama et al. Citation2005; Hirai et al. Citation2007). Further, Fe concentrations are less in some Fe deficiency-tolerant transgenic rice lines than in wild-type rice (Ogo et al. Citation2007).
Chloroplasts are the largest sink of Fe in leaves as well as the specialized organelles for photosynthesis (Terry and Abadia Citation1986). In the process termed photophosphorylation, electrons activated by light energy in photosystem II (PSII) are transferred to photosystem I (PSI), coupled with adenosine triphosphate (ATP) synthesis. These electrons move through an electron transport chain and are finally received by ferredoxin, which acts as a major reducing agent (Allen Citation2002) in the processes of carbon (C), nitrogen (N), and sulfur (S) assimilation. Many kinds of Fe-containing proteins are involved in the electron transport or biological redox reactions. PSI is the primary system affected by Fe deficiency, probably because of its high Fe concentration (12 Fe atoms per PSI unit) (Moseley et al. Citation2002). Consequently, Fe deficiency decreases the production of ATP (Winder and Nishio Citation1995) and reducing power derived from ferredoxin (Tognetti et al. Citation2007). Therefore, plant chloroplasts should be equipped with adaptive mechanisms for Fe deficiency. However, such mechanisms have not been studied extensively in higher plants. Recently, we reported an adaptation mechanism by the light-harvesting antenna system in barley, which was related to heat dissipation to compensate for excess light energy under prolonged Fe deficiency (Saito et al. Citation2010).
Although the mechanism highly contributed to the protection of young leaves of barley from photoinhibition caused by long-term Fe deficiency, the electron transport rate (ETR) from PSII to PSI in Fe-deficient barley leaves was markedly decreased like that in Fe-deficient rice leaves (Saito et al. Citation2010). The decrease in ETR may immediately limit production of ATP and reductants, negatively affecting subsequent assimilation pathways under Fe deficiency. Despite this, young chlorotic leaves of barley could continue to grow slowly under prolonged Fe deficiency, which was in contrast to the observation in rice in which continuous development of young leaves could not be observed even under short-term Fe-deficient conditions (Saito et al. Citation2010). This different growth response between the two graminaceous plants implies that barley possesses an additional adaptation mechanism for energy use efficiency downstream of the photosynthetic electron transport chain. However, the mechanism related to the compensation of decrease in energy supplied from the electron transport chain to subsequent photosynthetic reactions, including Calvin cycle and assimilation of N and S, has not been elucidated. Since barley requires assimilated C, N, and S to synthesize and release large amounts of mugineic acids (Mori and Nishizawa Citation1987), it is important to evaluate the alteration in fundamental metabolic pathways under Fe-deficient conditions.
Most N and S assimilation occurs in the plastids, and the expression of N and S assimilation-related genes is closely regulated to avoid the accumulation of intermediates (Buchanan et al. Citation2000). In addition, Fe is required by many enzymes involved in N and S assimilation, including nitrate reductase (NR, containing heme Fe), nitrite reductase (NIR, containing a [4Fe-4S] cluster), glutamate synthase (GOGAT, containing a [3Fe-4S] cluster), adenosine 5′-phosphosulfate reductase (APR, containing a [4Fe-4S] cluster), sulfite reductase (SIR, containing a [4Fe-4S] cluster), and the protein ferredoxin (containing a [2Fe-2S] cluster) (Campbell Citation1996; Balk and Lobréaux Citation2005). Thus, prolonged Fe deficiency markedly reduces N and S assimilation, leading to cessation of growth even if carbon dioxide (CO2) assimilation is maintained.
The interaction between plant nutrients and metabolic pathways has been discussed recently (Ohkama-Ohtsu and Wasaki Citation2010). However, knowledge about the relationships between Fe nutrition and other macronutrients within plant tissues is limited. In this study, we investigated the levels of transcripts involved in C, N, and S photoassimilation pathways in barley. We also compared the concentrations of C, N, and S as well as other major essential elements between barley leaves and rice leaves. The modulation of metabolite distribution in barley leaves to adapt to Fe deficiency is discussed.
Materials and Methods
Plant materials and growth conditions
Plants were hydroponically grown in a growth chamber (14-h light/10-h dark; photosynthetic photon flux density (PPFD), approximately 230 µmol m−2 s−1; for barley, 24°C light/20°C dark; for rice, 28°C light/25°C dark). The composition of the standard culture solution has been described by Maruyama et al. (Citation2005). Barley (Hordeum vulgare L. cv. Ehimehadaka No. 1) and rice (Oryza sativa L. cv. Nipponbare) seeds were germinated on wet paper for three days and six days, respectively. The seedlings were transferred to culture solutions containing half the concentrations of all the ingredients of the standard culture solution [supplemented with 30 µM Fe-ethylenediaminetetraacetic acid (EDTA)] and grown for four days (barley) or six days (rice). When the first true leaf appeared, the plants were transferred to the standard culture solution supplemented with 30 µM Fe-EDTA and grown for four days. When the second true leaf appeared in the barley plants, or the third true leaf appeared in the rice plants, nine barley plants or 15 rice plants were transplanted into a 5-L container. One group was grown with 30 µM Fe-EDTA and the other group was grown without Fe for eight days. The pH of the culture solution was adjusted to 5.5 with 0.5 M sulfuric acid (H2SO4) and 1 M sodium hydroxide (NaOH), and a fresh solution was prepared every 3 d.
At harvest, the leaves were numbered as follows: cotyledon, 1; first true leaf, 2; second true leaf, 3; and so on. Only the leaf blade was harvested. Three barley plants or five rice plants were pooled as one replicate. The chlorotic leaves (or parts of leaves) (leaf 5, leaf 4, and the basal part of leaf 3 of the barley plants; leaf 5 and the basal part of leaf 4 of the rice plants) of Fe-deficient plants were pooled and designated as “younger-Fe leaves.” The green leaves (or parts of leaves) (leaf 1, leaf 2, and the tip of leaf 3 of the barley plants; leaf 2, leaf 3, and the tip of leaf 4 of the rice plants) of Fe-deficient plants were pooled and designated as “older-Fe leaves.” Leaves 4 and 5 of the control barley plants or leaves 5 and 6 of the control rice plants were pooled and designated as “younger control leaves.” Leaves 1, 2, and 3 of the control barley plants or leaves 2, 3, and 4 of the control rice plants were pooled and designated as “older control leaves.” The pooled leaves were cut into about 1-cm segments, and the segments were divided into two groups. One group was frozen in liquid nitrogen and stored in a −80°C freezer until use for the measurement of chlorophyll and ion concentrations, western blot analysis, and reverse transcriptase-polymerase chain reaction (RT-PCR). The other group was dried and stored at room temperature until use for elemental analyses.
Microarray analysis
Total RNA was extracted from leaf 8 (the youngest fully expanded leaf) of Fe-deficient (0.3 µM Fe-EDTA) and Fe-sufficient (10 µM Fe-EDTA) plants from which the leaves were harvested during daylight at 14 days or 25 days after initiating treatment with each Fe concentration (Hirai et al. Citation2007). The prepared samples were hybridized to the Affymetrix GeneChip® Barley1 Genome Array, as described previously (Saito et al. Citation2010).
Chlorophyll concentrations
Chlorophylls were extracted with 80% acetone from liquid nitrogen-ground leaves; absorbance of the supernatants was measured at 663.6, 646.6, and 750 nm using a spectrophotometer (UV-1600; Shimadzu Co. Ltd., Kyoto, Japan), according to the method described by Porra et al. (Citation1989).
Elemental analyses
Total N and total C concentrations were measured using an elemental analyzer (CE-440; Exeter Analytical Inc., USA). Dried leaves were digested in a solution of concentrated nitric acid (HNO3) at 150°C and dissolved in 1% HNO3. Iron concentration was measured using an atomic absorption spectrophotometer (AA-6300; Shimadzu Co. Ltd); S, phosphorus (P), and calcium (Ca) concentrations were measured using an inductively coupled plasma-atomic emission spectrophotometer (ICPS-8000; Shimadzu Co. Ltd).
Ion concentrations
Frozen leaves were extracted with water, and the extracts were filtered through an ultrafiltration membrane (Microcon Ultracel YM-10; 10,000 molecular weight cut-off (MWCO); MILLIPORE Co. Ltd, USA). The flow-through solution was analyzed using an ion chromatograph (PIA-1000; Shimadzu Co. Ltd).
Reverse transcriptase-polymerase chain reaction (RT-PCR)
Total RNA was extracted using an RNeasy Plant Mini Kit (Qiagen K.K., Tokyo, Japan), and cDNA was synthesized from the total RNA by using the PrimeScript® II 1st strand cDNA Synthesis Kit (TaKaRa Bio Inc., Shiga, Japan). KOD FX DNA polymerase (TOYOBO Co. Ltd, Osaka, Japan) was used for amplification. Primers were designed from highly conserved sequences among graminaceous plant species. Thus, these primers could amplify the majority of the homologous genes in barley. The primers and reaction conditions are shown in . Signal intensity was determined using ImageJ v. 1.43.
Table 1 Primers and reaction conditions
Western blot analysis
Proteins were extracted from liquid nitrogen-ground leaves by using an extraction buffer containing 62.5 mM Tris-HCl at pH 6.8, 2.5% sodium dodecyl sulfate (SDS), and 10% glycerol. Homogenates were boiled and centrifuged, and protein concentrations in the supernatants were measured using the bicinchoninic acid (BCA) method (Smith et al. Citation1985). Sample aliquots were supplemented with 2-mercaptoethanol at a final concentration of 5%. SDS-polyacrylamide gel electrophoresis was performed with 12.5% gel. Proteins were electrotransferred to polyvinylidene difluoride (PVDF) membranes (Hybond™-P; GE Healthcare UK Ltd, Buckinghamshire, UK), and the membranes were probed with 1:1000 diluted anti-ferredoxin antibodies (Agrisera, Vännäs, Sweden). Detection was performed using the enhanced chemiluminescence (ECL) Plus Western Blotting Detection System (GE Healthcare UK Ltd) according to the manufacturer's protocols, with a 1:30,000 diluted peroxidase-labeled anti-rabbit antibody as the secondary antibody. Signal intensity was determined using ImageJ v. 1.43.
Results
Analysis of microarray data
In our previous study, we analyzed the microarray data of barley, focusing on photosynthesis under Fe-deficient condition (Saito et al. Citation2010). In this study, we re-analyzed those data, searching for genes related to the photoassimilation, transport, utilization, and recycling of C, N, and S among those upregulated or downregulated by Fe deficiency. Further, we focused on glutathione (GSH) metabolism as an intracellular redox buffer (Rouhier et al. Citation2008). GSH is also a major cysteine reservoir and thus greatly affects S metabolism.
(14-days Fe deficiency) and (25-days Fe deficiency) show the upregulated and downregulated genes related to the photoassimilation and transport of C, N, and S in Fe-deficient barley. As expected, ferredoxin gene was downregulated by Fe deficiency in the young leaves ( and ). Nitrogen and S assimilation-related genes were downregulated by 14-days Fe deficiency () but not by 25-days Fe deficiency (). Upregulation of thioredoxin/glutaredoxin gene ( and ) was consistent with the previous reports that indicated that thioredoxin/glutaredoxin is important under reducing power-starved conditions (Stenbaek et al. Citation2008; Forner-Giner et al. Citation2010). GSH transferases (GSTs) were also upregulated up to three-fold by Fe deficiency in the leaves ( and ). Furthermore, several sugar transporter genes were upregulated by Fe deficiency ( and ).
Table 2 Changed expression of genes related to photoassimilation and transport of carbon (C), nitrogen (N), and sulfur (S)
Table 3 Changed expression of genes related to photoassimilation and transport of carbon (C), nitrogen (N), and sulfur (S)
(14-days Fe deficiency) and (25-days Fe deficiency) show the upregulated and downregulated genes related to the utilization and recycling of C, N, and S in Fe-deficient barley. Cell wall and protein degradation-related genes were remarkably upregulated. The fact that several senescence- and ubiquitination-related genes were upregulated suggests controlled degradation of leaf cells. Upregulation of both protease and protease inhibitor genes also supports this suggestion (Solomon et al. Citation1999). These microarray data led us to conceive that extensive modulation of metabolism occurred in barley under Fe-deficient condition.
Table 4 Changed expression of genes related to utilization and recycling of carbon (C), nitrogen (N), and sulfur (S)
Table 5 Changed expression of genes related to utilization and recycling of carbon (C), nitrogen (N), and sulfur (S)
Conditions of plant materials used in biochemical analyses
Iron deficiency is more serious in young organs than in those that develop in the early stage of plant development. An effective way to monitor the disturbance in various metabolic pathways due to Fe deficiency at the whole-plant level is to differentiate the organs according to their age, depending on which the organs show different degrees of Fe-deficiency symptoms. Thus, we compared the concentrations of various components between younger and older leaves in this study. The culture solution used in this work contained only Ca(NO3)2 as the N source. This enabled us to compare barley and rice, which were grown under the same condition regarding the cost of reducing inorganic N.
First, we confirmed the growth of and chlorophyll and Fe concentrations in the plant materials used in all the experiments. Plant height and shoot fresh weight were more reduced in rice than in barley during the eight day Fe-deficiency treatment (): barley and rice shoot fresh weight decreased to 70.4% and 44.4%, respectively. However, the reduction in chlorophyll and Fe concentrations were similar between barley and rice (). These findings were consistent with our previous observations (Maruyama et al. Citation2005; Saito et al. Citation2010). These plants were used in subsequent experiments.
Figure 1 Chlorophyll and iron (Fe) concentrations in leaf blade. (A, B) Chlorophyll a + b concentrations are represented as average ± standard error (SE) (n = 3). (C, D) Iron concentrations are represented as average ± SE (n = 3). (A, C) Barley. (B, D) Rice. See Materials and Methods section for sample names.
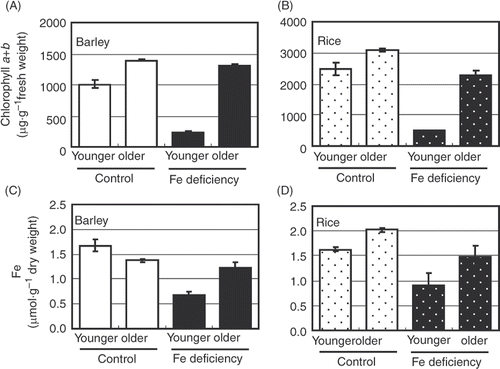
Table 6 Growth of barley and rice
Expression of molecules related to carbon, nitrogen, and sulfur assimilation in leaves
The accumulation of ribulose-1,5-bisphosphate carboxylase/oxygenase (RuBisCO) protein was decreased by Fe deficiency in the younger leaves of barley (). However, the accumulation was higher in the older leaves of Fe-deficient barley than in the older control leaves. RuBisCO accumulation was not altered considerably by Fe deficiency in rice. Thus, no relationship was found between chlorophyll concentration and RuBisCO accumulation. Next, we compared the accumulation of ferredoxin protein between barley and rice (). Predictably, it was dramatically reduced in both Fe-deficient barley and Fe-deficient rice.
Figure 2 Expression of molecules related to carbon (C), nitrogen (N), and sulfur (S) assimilation. (A) Western blot analysis of ferredoxin. Each lane was applied with 10 µg of proteins. Coomassie brilliant blue (CBB)-stained ribulose-1,5-bisphosphate carboxylase/oxygenase (RuBisCO) bands are also shown. (B) Reverse transcriptase-polymerase chain reaction (RT-PCR) of N and S assimilation-related genes from barley. See for gene names and reaction conditions. Values indicated in (A) and (B) represent the signal intensity relative to that for younger leaf of control plant. Values on the image of (B) represent the normalized values to intensity of elongation factor 1α (EF1-α). Western blot analysis and RT-PCR were performed at least twice. Typical images are presented. See Materials and Methods section for sample names.
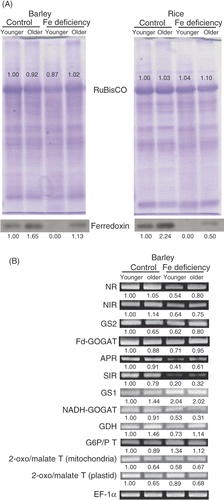
RT-PCR revealed that the accumulation of mRNAs of S assimilation-related APR and SIR was decreased in Fe-deficient barley (). The accumulation of mRNAs of N assimilation-related NR, NIR, glutamine synthase 2 (GS2, chloroplastic GS), and ferredoxin-dependent GOGAT (Fd-GOGAT) was decreased in Fe-deficient barley, especially in the younger leaves (). The accumulation of mRNAs of reduced nicotinamide adenine dinucleotide (NADH)-dependent GOGAT (NADH-GOGAT) and glutamate dehydrogenase (GDH) was decreased in both the younger and older leaves of Fe-deficient barley (). The expression of other genes involved in the metabolism of organic acids that support N assimilation, such as mitochondrial 2-oxoglutarate/malate translocator gene, was decreased in the younger leaves of Fe-deficient barley, while that of glucose-6-phosphate/phosphate (G6P/P) translocator gene was increased in both the younger and older leaves of Fe-deficient barley (). The accumulation of mRNA of N translocation-related GS1 (cytosolic GS) was increased in both the younger and older leaves of Fe-deficient barley ().
Leaf blade components
Molecular and biochemical analyses suggested that Fe deficiency affects the metabolism of major essential elements. Thus, we analyzed the total concentrations and concentrations of the major inorganic forms of several elements in barley and rice leaves.
Total C concentration was decreased in both the younger and older leaves of Fe-deficient rice (), whereas it was decreased in the younger leaves of Fe-deficient barley but increased in the older leaves ().
Table 7 Components of leaf blade
Total N and nitrate ion () concentrations were increased in the younger leaves and decreased in the older leaves of Fe-deficient barley. Ammonium ion (
) concentration was decreased in the younger leaves of Fe-deficient barley. Total N concentration was decreased in both the younger and older leaves of Fe-deficient rice. Nitrate ion (
) concentration was dramatically increased in the younger leaves and decreased in the older leaves of Fe-deficient rice. Ammonium ion (
) concentration was increased in both the younger and older leaves of Fe-deficient rice ().
Total S and sulfate ion () concentrations were increased by Fe deficiency in the older leaves of barley but in the younger leaves of rice (). Total P and total Ca concentrations were increased by Fe deficiency in both the younger and older leaves of barley and rice. Dihydrogen phosphate ion (
) and Ca2+ concentrations were also increased by Fe deficiency, but the increases were greater in rice than in barley (). Concerning other macronutrients, total potassium (K), total magnesium (Mg), K+, and Mg2+ concentrations were not altered significantly by Fe deficiency in both barley and rice (data not shown).
Thus, the effects of Fe deficiency on the metabolism of major essential elements were different between barley and rice leaves.
Discussion
In this study, a reasonable decrease was observed in ferredoxin protein by Fe deficiency. Total C concentration decreased by Fe deficiency in the younger leaves of barley and rice. However, the effects of Fe deficiency on the growth of and components of leaf blades of barley were opposite to or lesser than those on the growth of and components of leaf blades of rice (). In particular, growth inhibition in barley was lesser than that in rice (). Nevertheless, the decrease in NR, NIR, GS2, and Fd-GOGAT mRNAs was remarkable in the younger-Fe leaves of barley. These results suggest that barley could suppress the fluctuation in nutrient concentrations caused by Fe deficiency and regulate the assimilation rate to become rather low under the Fe-deficient condition. Since the energy cost of reducing to
corresponds to 12–26% of the photosynthetically generated reductant (Bloom Citation1997; Noctor and Foyer Citation1998), downregulation of N assimilation may be one of the important adaptations to Fe deficiency.
Table 8 Summary of the impacts of iron (Fe) deficiency
We have previously reported that visible senescence of older leaves of barley is accelerated by Fe deficiency (Maruyama et al. Citation2005). Total N and concentrations were decreased by Fe deficiency in the older leaves of barley ( and ), and proteolysis-, senescence-, and amino acid re-utilization-related genes were upregulated by Fe deficiency in both the younger and older leaves of barley ( and ). Therefore, turnover and redistribution of N could be induced in Fe-deficient barley. Our finding that the expression of GS1, which contributes to N remobilization (Tobin and Yamaya Citation2001), was induced by Fe deficiency ( and ) also supports this hypothesis. In the younger-Fe leaves of barley, the genes related to N assimilation were greatly downregulated as mentioned earlier. Thus, the ability of N assimilation from
newly acquired via the roots should be markedly reduced in the younger-Fe leaves of barley, which would result in an increase in
concentration in these leaves. However, the rate of increase in
concentration in the younger-Fe leaves was lower in barley (144%) than in rice (513%), compared with the younger control leaves (). These results seem to indicate active remobilization of organic N from the older leaves to the younger leaves of Fe-deficient barley. Ferredoxin protein and mRNAs of N assimilation-related genes could be well detected in the older leaves of Fe-deficient barley ( and ), as also the concentrations of chlorophylls and Fe (). Thus, the older-Fe leaves of barley may be able to assimilate newly acquired
. Interestingly, high-affinity
transporter gene was upregulated in the older leaves of barley after 25 days of Fe deficiency by approximately two-fold that in the leaves of control barley (). This seems to support active N assimilation in the older-Fe leaves. Further, Arabidopsis thaliana nitrate transporter NRT1.7 is induced in senescent leaves and contributes to remobilize
(Fan et al. Citation2009). In Fe-deficient barley, both organic N and
could be transported from the older leaves to the younger leaves. Taken together, there is a possibility that the older leaves of Fe-deficient barley simultaneously assimilated
and supplied N compounds, supported by enhanced proteolysis, senescence, and amino acid recycling at the transcriptional level ( and ).
In contrast to the increased total N concentration in the younger leaves of Fe-deficient barley, the total N concentration decreased in both the older and younger leaves of Fe-deficient rice. However, concentration increased in both the younger and older leaves of Fe-deficient rice but not in Fe-deficient barley. Because the
concentration in the culture solution used in this study was extremely low (4 mM
as a calcium salt and 60 nM
as a molybdenum salt) and the primary
assimilation pathway should be strongly inhibited under Fe-deficient conditions, increased
in the leaves of Fe-deficient rice was thought to be derived from the photorespiration pathway. Indeed,
handled by the photorespiratory N cycle in a photorespiring leaf can be up to 20 times than that resulting from the reduction of
(Guo et al. Citation2007). However, photorespiration requires an extra amount of NADH and ATP for the reassimilation process of
, which is consequently produced in the photorespiration process. As a result, it can be considered that Fe-deficient rice could not appropriately reassimilate
from the photorespiration pathway because of energy shortage during Fe deficiency. However, an increase in GS1 in the whole shoots of Fe-deficient barley might prevent an increase in
concentration in barley, even though the production of photorespiratory
was enhanced during Fe deficiency. It is suggested that there is sufficient GS1 to support primary assimilation in barley leaves (Tobin and Yamaya Citation2001).
In the younger leaves of Fe-deficient barley, S assimilation-related genes and transporter gene were downregulated (). It is reasonable because the reducing power in these leaves could be remarkably decreased in Fe deficiency. However, Fe deficiency-induced increase in S assimilation in Strategy II plants may contribute to the biosynthesis of mugineic acid phytosiderophores (Astolfi et al. Citation2006). Total S and
concentrations increased in the older leaves of Fe-deficient barley ( and ). Since ferredoxin protein and mRNAs of APR and SIR could be detected in the older-Fe leaves of barley (), it is indicated that barley may assimilate S in the older-Fe leaves. Increased total C concentration in the older leaves of Fe-deficient barley may provide the basis for S assimilation. Unlike the total S and
concentrations in the younger leaves of Fe-deficient barley, total S and
concentrations were increased in the younger leaves of Fe-deficient rice ( and ). However, ferredoxin protein could not be detected and
concentration increased two-fold in the younger-Fe leaves of rice (); thus, active S assimilation is questionable in Fe-deficient rice.
Our microarray analysis revealed the upregulation of mitochondrial monothiol glutaredoxin (GRX) homologs in Fe-deficient barley leaves ( and ). In yeast, Grx5 knockout leads to Fe accumulation in the cell and inactivation of [Fe-S]-containing enzymes in mitochondria, suggesting that GRXs play a role in [Fe-S] protein synthesis or repair of the scaffoldings (Wingert et al. 2005; Rouhier et al. Citation2008). Recently, it has been reported that plastidic plant GRXs can act as scaffold proteins in the transfer of [Fe-S] clusters from the synthesis machinery to target apoproteins (Bandyopadhyay et al. Citation2008). The upregulation of GRXs in Fe-deficient barley leaves ( and ) might be important not only to overcome the limited reducing power from photosynthesis (Forner-Giner et al. Citation2010) but also to facilitate synthesis of [Fe-S] proteins under Fe-deficient conditions.
GSTs were also upregulated up to three-fold by Fe deficiency ( and ). Glutathionylation of protoporphyrinogen, which is a precursor of chlorophylls and heme, can be catalyzed by maize GSTs in vitro (Dixon et al. Citation2008), suggesting the possibility of direct involvement of GSTs in Fe homeostasis and photosynthesis. Thus, GSTs in Fe-deficient barley may contribute to better tolerance to Fe deficiency in leaves. Increased level of a GST transcript has been found in Fe-starved alfalfa (Arakawa et al. Citation2002), indicating that a common scenario of GST function under Fe deficiency may be observed in land plants.
In addition to GRXs and GSTs, 2 glutathione reductase (GR) genes, HvGR1 (chloroplastic) and HvGR2 (cytosolic), have been reported as Fe deficiency-responsive genes (Bashir et al. Citation2007). The investigators reported that the genes might be involved in internal Fe homeostasis and scavenging reactive oxygen species. However, we could not detect the responses of HvGR1 and HvGR2 to Fe deficiency in leaves in the present study ( and ). This discrepancy was thought to be due to the weak response of HvGR1 to Fe deficiency and the weak expression level of HvGR2 in shoot tissues, as indicated in the report of Bashir et al. (Citation2007) (i.e. GR activity was increased by only 1.27-fold in the shoot tissues of Fe-deficient barley). Nevertheless, the response of the GR genes to Fe deficiency was insignificant even in the barley leaves. The steady levels of the HvGR transcripts under Fe-deficient conditions may indicate the importance of these genes in maintaining the cellular redox status under Fe-deficient conditions.
The expression patterns of the majority of genes shown in Tables were similar between 14-day and 25-day Fe-deficiency treatments. However, N and S assimilation-related genes were downregulated by 14-days Fe deficiency () and by eight-days Fe deficiency () but not by 25-days Fe deficiency (). RuBisCO protein accumulation was decreased by eight-days Fe deficiency (), while both its small and large subunit genes were upregulated by 14-days Fe deficiency () and showed no change in expression after 25 days of Fe deficiency () in barley leaves. A cycle of leaf-color change, severe chlorosis, and re-greening can be observed during long-term Fe deficiency in barley but not in rice (Maruyama et al. Citation2005; Hirai et al. Citation2007). In our growth chamber or greenhouse, severe chlorosis was observed at about two weeks, and new green leaves developed at about three weeks in Fe-deficient barley after transplantation in Fe-deficient nutrient solution. The highest chlorophyll a/b ratio was observed at 12 days, and this ratio decreased to the control level at 20 days (Saito et al. Citation2010). Primary C, N, and S assimilation-related genes could be transiently downregulated to reduce the consumption of reducing power during the early stage of adaptation to Fe deficiency and restored to the normal level after complete adaptation to Fe deficiency.
Alteration of metabolism as mentioned earlier could contribute to mugineic acid synthesis in Fe-deficient barley. In the younger-Fe leaves of barley, N and S assimilation may be suppressed, and insufficient organic N in these leaves may be supplemented with remobilized organic N from the older-Fe leaves, accompanying senescence enhanced by Fe deficiency. The organic N released from the older-Fe leaves may also be remobilized to the roots. Moreover, the older-Fe leaves may assimilate S, and the assimilated S may be remobilized to the roots and contribute to mugineic acid synthesis with remobilized C and N. Mori et al. (Citation1991) reported that the secretion of mugineic acids by rice ceased within 10 days after Fe-deficiency treatment. Rice could not remobilize and supply enough substrates to synthesize a large amount of mugineic acids. Tracer experiments and metabolomic analyses will clarify the adaptive mechanisms for substance distribution in barley in Fe deficiency.
Disturbance in the metabolism of fundamental organic compounds could influence other minerals. Total P and total Ca concentrations were increased by Fe deficiency in both barley and rice ( and ). Such an increase has also been reported previously (Fleming and Foy Citation1982). However, water-extractable and Ca2+ concentrations were dramatically increased in both the younger and older leaves of Fe-deficient rice, but not in Fe-deficient barley. Thus, the metabolism of major essential elements was significantly disturbed in rice by only the eight day of Fe-deficiency treatment not only in the younger leaves but also in the older leaves. Phosphorus decreases the concentration of water-soluble Fe and promotes Fe-deficiency symptoms in rice (Ladouceur et al. Citation2008; Zheng et al. Citation2009). We have previously reported that Fe deficiency dramatically decreases the ratio of water-soluble Fe to total Fe in rice shoots, but not in barley (Maruyama et al. Citation2005). The increase in
concentration in rice may also be a reason for the susceptibility of rice to Fe deficiency.
Our findings suggest that barley possesses a comprehensive mechanism for Fe-deficiency tolerance. Elucidation of such a mechanism will provide a cue for improving low Fe-deficiency tolerance and potentially enhancing Fe utilization efficiency in crops.
References
- Allen , JF . 2002 . Photosynthesis of ATP—electrons, proton pumps, rotors, and poise . Cell , 110 : 273 – 276 .
- Arakawa , Y , Masaoka , Y , Sakai , J , Higo , H and Higo , K . 2002 . An alfalfa gene similar to glutathione S-transferase is induced in root by iron deficiency . Soil Sci. Plant Nutr. , 48 : 111 – 116 .
- Astolfi , S , Zuchi , S , Cesco , S , di Toppi , LS , Pirazzi , D , Badiani , M , Varanini , Z and Pinton , R . 2006 . Iron deficiency induces sulfate uptake and modulates redistribution of reduced sulfur pool in barley plants . Funct. Plant Biol. , 33 : 1055 – 1061 .
- Balk , J and Lobréaux , S . 2005 . Biogenesis of iron–sulfur proteins in plants . Trends Plant Sci. , 10 : 324 – 331 .
- Bandyopadhyay , S , Gama , F Molina-Navarro , MM . 2008 . Chloroplast monothiol glutaredoxins as scaffold proteins for the assembly and delivery of [2Fe–2S] clusters . EMBO J. , 27 : 1122 – 1133 .
- Bashir , K , Nagasaka , S , Itai , RN , Kobayashi , T , Takahashi , M , Nakanishi , H , Mori , S and Nishizawa , NK . 2007 . Expression and enzyme activity of glutathione reductase is upregulated by Fe-deficiency in graminaceous plants . Plant Mol. Biol. , 65 : 277 – 284 .
- Bloom , AJ . 1997 . “ Nitrogen as a limiting factor: crop acquisition of ammonium and nitrate ” . In Ecology in Agriculture , Edited by: Jackson , LE . 145 – 172 . San Diego , CA : Academic Press .
- Buchanan , BB , Gruissem , W and Jones , RL . 2000 . Biochemistry and Molecular Biology of Plants, , 4th , Rockville , MD : American Society of Plant Physiologists .
- Campbell , WH . 1996 . Nitrate reductase biochemistry comes of age . Plant Physiol. , 111 : 355 – 361 .
- Dixon , DP , Lapthorn , A , Madesis , P , Mudd , EA , Day , A and Edwards , R . 2008 . Binding and glutathione conjugation of porphyrinogens by plant glutathione transferases . J. Biol. Chem. , 283 : 20268 – 20276 .
- Fan , SC , Lin , CS , Hsu , PK , Lin , SH and Tsay , YF . 2009 . The Arabidopsis nitrate transporter NRT1.7, expressed in phloem, is responsible for source-to-sink remobilization of nitrate . Plant Cell , 21 : 2750 – 2761 .
- Fleming , AL and Foy , CD . 1982 . Differential response of barley varieties to Fe stress . J. Plant Nutr. , 5 : 457 – 468 .
- Forner-Giner , MA , Llosá , MJ , Carrasco , JL , Perez-Amador , MA , Navarro , L and Ancillo , G . 2010 . Differential gene expression analysis provides new insights into the molecular basis of iron deficiency stress response in the citrus rootstock Poncirus trifoliata (L.) Raf . J. Exp. Bot. , 61 : 483 – 490 .
- Guo , S , Zhou , Y , Shen , Q and Zhang , F . 2007 . Effect of ammonium and nitrate nutrition on some physiological processes in higher plants – growth, photosynthesis, photorespiration, and water relations . Plant Biol. , 9 : 21 – 29 .
- Hirai , M , Higuchi , K , Sasaki , H , Suzuki , T , Maruyama , T , Yoshiba , M and Tadano , T . 2007 . Contribution of iron associated with high-molecular-weight substances to the maintenance of the SPAD value of young leaves of barley under iron-deficient conditions . Soil Sci. Plant Nutr. , 53 : 612 – 620 .
- Ishimaru , Y , Kim , S Tsukamoto , T . 2007 . Mutational reconstructed ferric chelate reductase confers enhanced tolerance in rice to iron deficiency in calcareous soil . Proc. Natl. Acad. Sci. USA , 104 : 7373 – 7378 .
- Jeong , J and Guerinot , ML . 2009 . Homing in on iron homeostasis in plants . Trends Plant Sci. , 14 : 280 – 285 .
- Ladouceur , A , Akiha , F and Kawai , S . 2008 . Effect of supplied phytosiderophore on 59Fe absorption and translocation in Fe-deficient barley grown hydroponically in low phosphorus media . Soil Sci. Plant Nutr. , 54 : 560 – 565 .
- Marschner H 1995: Mineral Nutrition of Higher Plants (2nd edition), pp. 58–62, 313–324, 641–648. Academic Press, London.
- Maruyama , T , Higuchi , K , Yoshiba , M and Tadano , T . 2005 . Comparison of iron availability in leaves of barley and rice . Soil Sci. Plant Nutr. , 51 : 1035 – 1042 .
- Mori , S and Nishizawa , N . 1987 . Methionine as a dominant precursor of phytosiderophores in Graminaceae plants . Plant Cell Physiol. , 28 : 1081 – 1092 .
- Mori , S , Nishizawa , NK , Hayashi , H , Chino , M , Yoshimura , E and Ishihara , J . 1991 . “ Why are young rice plants highly susceptible to iron deficiency? ” . In Iron Nutrition and Interactions in Plants , Edited by: Chen , Y and Hadar , Y . 175 – 188 . Dordrecht : Kluwer Academic Publishers .
- Moseley , JL , Allinger , T , Herzog , S , Hoerth , P , Wehinger , E , Merchant , S and Hippler , M . 2002 . Adaptation to Fe-deficiency requires remodeling of the photosynthetic apparatus . EMBO J. , 21 : 6709 – 6720 .
- Noctor , G and Foyer , CH . 1998 . A re-evaluation of the ATP: NADPH budget during C3 photosynthesis: a contribution from nitrate assimilation and its associated respiratory activity? . J. Exp. Bot. , 49 : 1895 – 1908 .
- Ogo , Y , Itai , RN , Nakanishi , H , Kobayashi , T , Takahashi , M , Mori , S and Nishizawa , NK . 2007 . The rice bHLH protein OsIRO2 is an essential regulator of the genes involved in Fe uptake under Fe-deficient conditions . Plant J. , 51 : 366 – 377 .
- Ohkama-Ohtsu , N and Wasaki , J . 2010 . Recent progress in plant nutrition research: cross-talk between nutrients, plant physiology and soil microorganisms . Plant Cell Physiol. , 51 : 1255 – 1264 .
- Porra , RJ , Thompson , WA and Kriedemann , PE . 1989 . Determination of accurate extinction coefficients and simultaneous equations for assaying chlorophylls a and b extracted with four different solvents: verification of the concentration of chlorophyll standards by atomic absorption spectroscopy . Biochim. Biophys. Acta , 975 : 384 – 394 .
- Rouhier , N , Lemaire , SD and Jacquot , JP . 2008 . The role of glutathione in photosynthetic organisms: emerging functions for glutaredoxins and glutathionylation . Annu. Rev. Plant Biol. , 59 : 143 – 166 .
- Saito , A , Iino , T , Sonoike , K , Miwa , E and Higuchi , K . 2010 . Remodeling of the major light-harvesting antenna protein of photosystem II protects the young leaves of barley (Hordeum vulgare L.) from photoinhibition under prolonged iron deficiency . Plant Cell Physiol. , 51 ( 12 ) : 2013 – 2030. . DOI: 10.1093/pcp/pcq160
- Smith , PK , Krohn , RI , Hermanson , GT , Mallia , AK , Gartner , FH , Provenzano , MD , Fujimoto , EK , Goeke , NM , Olson , BJ and Klenk , DC . 1985 . Measurement of protein using bicinchoninic acid . Anal. Biochem. , 150 : 76 – 85 .
- Solomon , M , Belenghi , B , Delledonne , M , Menachem , E and Levine , A . 1999 . The involvement of cysteine proteases and protease inhibitor genes in the regulation of programmed cell death in plants . Plant Cell , 11 : 431 – 444 .
- Stenbaek , A , Hansson , A , Wulff , RP , Hansson , M , Dietz , KJ and Jensen , PE . 2008 . NADPH-dependent thioredoxin reductase and 2-Cys peroxiredoxins are needed for the protection of Mg–protoporphyrin monomethyl ester cyclase . FEBS Lett. , 582 : 2773 – 2778 .
- Takahashi , M , Nakanishi , H , Kawasaki , S , Nishizawa , NK and Mori , S . 2001 . Enhanced tolerance of rice to low iron availability in alkaline soils using barley nicotianamine aminotransferase genes . Nat. Biotechnol. , 19 : 466 – 469 .
- Terry , N and Abadia , J . 1986 . Function of iron in chloroplasts . J. Plant Nutr. , 9 : 609 – 646 .
- Tobin , AK and Yamaya , T . 2001 . Cellular compartmentation of ammonium assimilation in rice and barley . J. Exp. Bot. , 52 : 591 – 604 .
- Tognetti , VB , Zurbriggen , MD , Morandi , EN , Fillat , MF , Valle , EM , Hajirezaei , MR and Carrillo , N . 2007 . Enhanced plant tolerance to iron starvation by functional substitution of chloroplast ferredoxin with a bacterial flavodoxin . Proc. Natl. Acad. Sci. USA , 104 : 11495 – 11500 .
- Winder , TL and Nishio , JN . 1995 . Early iron deficiency stress response in leaves of sugar beet . Plant Physiol. , 108 : 1487 – 1494 .
- Wingert , RA , Galloway , JL Barut , B . 2000 . Screen Consortium 2005: Deficiency of glutaredoxin 5 reveals Fe-S clusters are required for vertebrate haem synthesis . Nature , 436 : 1035 – 1039 .
- Zheng , L , Huang , F Narsai , R . 2009 . Physiological and transcriptome analysis of iron and phosphorus interaction in rice seedlings . Plant Physiol. , 151 : 262 – 274 .