Abstract
Upland blueberry (Vaccinium ashei Read.) requires acidic and organic matter rich soils for its cultivation. Recently, the raised bed cultivation system has widely been used for blueberry cultivation, but it has a potential to cause nitrogen (N) losses via leaching and nitrous oxide (N2O) emission especially with high rate of N application. We investigated the effects of organic amendments on N losses; via leaching ammonium () and nitrate (
) and N2O emission under acid soil conditions. The treatments were (i) ammonium sulfate application (AS), (ii) AS with peat moss (AS + PM), and (iii) AS with sawdust compost (AS + SD). A container (surface area: 47 cm × 40 cm = 0.188 m2) experiment to simulate raised bed cultivation system using an ochric Cambisol was conducted at Chiba University, Japan, during 2008 and 2009. A blueberry plant in each container received N fertilizer three times: 14.8, 23.8, and 14.8 g N m−2 in July 2008, March, and July 2009, respectively.
Incorporation of peat moss and sawdust compost with ferrous sulfate effectively decreased soil pH to its optimal ranges (4.0–5.2) and resulted in increased growth and yield of blueberry (berry yield of 2009: 93, 285, and 348 g plant−1 in AS, AS + PM, and AS + SD treatment, respectively) due to increased soil porosity and consequently enhanced plant root biomass. Concurrently, the AS + SD treatment resulted in the least N losses, followed by AS + PM and AS treatment both through leaching and N2O emission. The emitted N2O amount over about two-year blueberry cultivation were 1.28, 0.80, and 0.58 g N m−2 for AS, AS + PM, and AS + SD treatments, respectively. These results indicated that application of organic amendments decreased N losses to the environment most likely due to increased plant N uptake, microbial N immobilization, and consequent reduction in nitrification, denitrification, and leaching.
Introduction
Nitrogen (N) losses from many agricultural systems like arable land and pastures have been studied extensively, but limited information is available on N losses from perennial orchards like blueberries (Vaccinium sp.) planted on upland soils. Chemical N fertilizers may result in heavy N losses that can cause water contamination through nitrate () leaching and fertilizer induced nitrous oxide (N2O) emission that is a current concern worldwide (Mosier Citation1994; Di and Cameron Citation2002). Blueberries require light textured high organic content soils with low soil pH (4.0–5.2) (Coville Citation1910) and shallow water table (<40 cm) for their normal growth (Williamson et al. Citation2006). The annual N demand of blueberries generally ranges from 20 to 140 kg N ha−1, depending on the regions (Eck Citation1988; Strik et al. Citation1993) and on growth stages; however, mulched blueberry plantations on upland soils require much more N to offset the microbial immobilization during mulch decomposition (Strik and Hart Citation1991). Also, blueberry seedlings that have been recently planted require more N fertilizer application to establish their good growth, creating possibilities of N losses to the environment. Moreover, as many other acidophilous plants, the blueberries can utilize only ammonium (
) efficiently as a form of N (Sugiyama and Hanawa Citation1992; Tamada Citation2004). Therefore, nitrification is an undesirable process for this crop. Due to the scarcity of edaphically suited sites for blueberries worldwide, commercial plantation on upland soils has recently been accepted as a popular establishment mean for blueberry cultivation. However, upland soils in general have high pH and low organic matter content, in contrast to what blueberries require (Banados 2009). To provide favorable conditions for blueberries, the application of soil acidifiers (Haynes Citation1986) and incorporation of soil organic amendments (Hayler Citation2006; Taquini Citation2006) to a pre-planting soil during soil preparation are required.
Peat moss is considered as an optimal organic amendment to improve the physical properties of upland soils for blueberry cultivation due to its low pH, fine texture and good water retention ability (Spiers Citation1986). Forestry by-products can also be suggested as useful and sustainable soil amendments for blueberries compared with peat moss. Approximately 6.1 million tons of wood industry by-products (i.e. sawdust and bark) are generated annually in lumber mills (Ministry of Construction Japan Citation1998); 5.8 million m3 of wood chips are produced in Japan (MAFF 2008); these organic materials are annually applied to agricultural lands as a soil amendment.
Increasing organic matter contents in soils and lowering soil pH through the incorporation of organic materials for blueberry cultivation are likely to affect the processes of N transformations and losses. Treating soils with composts is reported to change the physical and hydraulic characteristics in a positive way due to the beneficial effects of organic matter additions (Correa et al.
Citation2005). The total N content in such compost consists of organic N (80%), -N (19%), and
-N (less than 1%) (US EPA Citation1995). Immobilization of N in organic amendment occurs right after its incorporation into soils; therefore, chemical N fertilizer should be applied considering the time when blueberry requires it. Specifically, blueberries take up N most vigorously from flowering to berry maturity stages (Darnell et al.
Citation1992). These phenological stages coincide with the heavy rainfall events in June and July in the Kanto region of Japan. Therefore, the risk for leaching losses of
after fertilizer application is high. Moreover, N2O could also be released as a by-product of nitrification (Inubushi et al.
Citation1996) or as the intermediate product in denitrification, and these processes may take place in the semi-aerobic aggregates of mineral soil after heavy rainfalls (Rappold and Crawford Citation1999). Under these conditions, the magnitude of nitrification and subsequent
leaching and N2O emission can be regulated by soil acidification through the application of amendments (Daum and Schenk Citation1998; Mogge et al.
Citation1999).
Incorporation of soil organic amendments with high carbon/nitrogen (C:N) ratio enhances the microbial immobilization of N and may reduce the N2O emission (Aulakh et al.
Citation1991; Shelp et al.
Citation2000; Huang et al.
Citation2004; Toma and Hatano Citation2007). Normally, when plant residues having a C:N ratio that is greater than 20 are added to the soil, the available N is immobilized during the first few weeks of decomposition (Green and Blackmer Citation1995). Due to the high C:N ratio of sawdust, it has a potential to reduce the leaching losses of when applied as compost (Bugbee Citation1999). Furthermore, blueberries can particularly respond to incorporated sawdust (Cummings et al.
Citation1981; Peterson et al.
Citation1987) or peat moss (Spiers Citation1986) before plantation that can enhance root growth resulting in increased N uptake and water use efficiency (Throup-Kristensen Citation2001; Kristensen and Throup-Kristensen Citation2004; Hashimoto et al. Citation2007).
This study was conducted to evaluate the environmental impacts of upland ochric Cambisol blueberry cultivation using peat moss or sawdust compost as amendments before plantation together with ammonium sulfate ((NH4)2SO4). Specifically, we aimed to investigate the effects of peat moss and sawdust compost incorporation together with (NH4)2SO4 on (i) the plant yield and biomass of blueberry plants and (ii) the N losses via leaching and N2O emission.
Materials and Methods
Soil samples and its sampling site
The soil used in this experiment was collected from 0 to 20 cm depth in Chiba Prefectural Agricultural Experimental Station, Tateyama, Japan in March 2007. The soil was classified as ochric Cambisol (FAO/UNESCO 1990) or Brown Forest Soil (Obara et al. Citation1995). Brown Forest Soil represents the major soil type in Japan (Anzai Citation2001). Soil samples were collected, air-dried, and passed through a 2 mm sieve mesh. The selected physical and chemical properties of the original soil served for the blueberry cultivation are presented in . The soil-sampling site has a temperate and humid climate with the mean annual temperature of 15.9°C and annual precipitation of 1466 mm that occurs mainly as rainfall during the months of June and July. The native vegetation of the sampling site was a mixture of deciduous and broad leaf evergreen forest.
Table 1 Selected chemical and physical properties of Tateyama soil (Cambisol), peat moss (PM), and sawdust (SD) compost served for the cultivation experiment and soils amended with PM and SD compost plus ferrous sulfate. The values for PM and SD compost amended soils were measured before planting blueberry seedlings in April 2008
Blueberry cultivation
Cultivation of Rabbiteye blueberry cv. “Tifblue” (Vaccinium ashei Read. “Tifblue”) in this experiment simulated the widespread conventional raised bed cultivation method using upland soils. Blueberries were grown in the experimental field of Chiba University, Graduate School of Horticulture, Matsudo, Japan (35°46′N, 139°54′E), under field conditions where the climatic condition is similar to that of Tateyama. The experiment was conducted using a randomized complete block design with three replications. The treatments received (i) (NH4)2SO4 alone (AS); (ii) (NH4)2SO4 with peat moss (AS + PM), and (iii) (NH4)2SO4 with sawdust compost and ferrous sulfate (AS + SD). Ferrous sulfate was applied at the rate of 200 g per experimental unit (a container) as a soil acidifier. The bottom layers of 40 L containers (47 cm × 40 cm × 22 cm, width × length × height, surface area: 0.188 m2) were filled with quartz grain to facilitate drainage. Then, the containers for AS + PM and AS + SD treatments were filled with soil (20 L) and commercially supplied peat moss (imported from Canada) or market available sewage sludge compost amended with sawdust (hereafter referred to as sawdust compost) (20 L), while the containers for AS treatment were filled with only soil (40 L). The organic amendments and ferrous sulfate were thoroughly mixed with the soil. Phosphorous and potassium were not applied due to low requirements of a blueberry plant (Gough Citation1994; Wilber and Williamson Citation2008). Selected properties of peat moss and sawdust compost are shown in . A seedling of one-year old Rabbiteye blueberry with well developed generative buds was planted to each container on April 20, 2008. Seedlings that had similar branch growth were selected to serve the experiments, so that the initial characteristics of seedling may not affect the treatment effects. The surface of the growth media in all the pots was covered with a 5 cm pine bark mulch to simulate upland raised bed conditions for blueberry plants. According to the recommendations of Strik and Hart (Citation1991) for a mulched upland blueberry plantation, (NH4)2SO4 was applied to each seedling at the rate of 38.6 g N m−2 year−1 (7.27 g N plant−1), broadcasted three times during the experiment to satisfy the recommendation (14.8, 23.8, and 14.8 g N m−2 on July 9, 2008, March 18, and July 1, 2009, respectively).
Growth and nitrogen uptake of blueberry plant
Five leaves positioned at the branch terminal were collected periodically and dried at 62°C for 14 days and were ground using a ball mill (Retsch MM200, Germany) to determine total N concentration using a CN corder (Yanaco MT-700, Yanagimoto, Japan). The blueberry fruits were harvested and weighed as fresh (and after dried in an oven at 62°C for 14 days) throughout the ripening season of berry maturity. In the end of the experiment on October 31, 2009, all the roots, leaves, and shoots were removed, weighed after drying at 62°C for 14 days, and analyzed to determine N concentrations. The total N uptake of a plant was calculated based on the N contents of roots, leaves, shoots, and blueberry fruits after the destructive harvest on October 31, 2009. The calculation assumes 1.0 m × 1.0 m plant spacing (Strik and Hart Citation1991).
Soil samples and leachate analyses
Soil samples were collected from the 0–5 cm depth at different times from three randomly selected points from each container and served as a composite sample of 10 g soil to analyze pH and and
concentrations. The amounts of soil taken from each container were immediately replaced with the same soil to minimize soil disturbance during the experimental period. The pH (1:2.5, soil/water) of the soil and drainage water were determined using a glass electrode method (HORIBA D52 pH meter, Japan). Soil samples were extracted using 0.5 M potassium sulfate (K2SO4) solution and analyzed for
concentration by the nitroprusside method (Anderson and Ingram Citation1989) and for
concentration by the hydrazine reduction method (Hayashi et al.
Citation1997), respectively, using a spectrophotometer (UV-1200 V, Shimadzu, Japan). Soil retained
and
concentrations were determined based on the values measured using the samples taken on the last sampling day (September 15, 2009).
A plastic bottle to collect leachates was attached to the base of each cultivation container, connected by a plastic tube, and leachates were collected after each significant rainfall. Cumulative leaching losses of and
in leachates were calculated by multiplying the volume by each mineral N concentration in the leachates.
Nitrous oxide flux measurements
Nitrous oxide (N2O) flux from soil surface was monitored using the closed chamber (1.8 L, 155 mm ×130 mm × 90 mm, width × length × height) method throughout the experimental period. A chamber base was installed throughout the experimental period, and a chamber was placed on the top of the base to minimize the soil disturbance effects on N2O emission (Jumadi et al. Citation2008). Gas samples were taken three times at 15-min intervals (at times t 0, t 15, and t 30) from each chamber using a 30-mL polypropylene syringes fitted with a three-way stopcock during the mid-morning hours (9–10 a.m.) of each sampling day to exclude the effects caused by the variation in sampling times. Gas samples were taken on every other day during one month period followed by each fertilizer application, on weekly basis after one month of application, except from January to middle March due to the cold temperature. Gas samples were immediately transferred to a pre-evacuated 22 mL gas vials and analyzed N2O concentrations using a gas chromatograph (GC-14B, Shimadzu, Japan) equipped with a 63Ni-electron capture detector and an automatic headspace gas sampler (HS 40 XL, Perkin Elmer, Germany). The carrier gas consisted of 5% methane in argon, and its flow rate was 20 mL min−1. The temperature of column, injector, and detector were set at 70, 120, and 280°C, respectively. The flux of N2O was calculated as the rate of the changes in the N2O concentration in the chamber, estimated as a slope of linear regression between N2O concentration and time (Pang et al. 2009). Cumulative N2O emissions from each treatment were calculated by integrating daily fluxes from the three replicates, assuming the constant flux rates between two gas sampling times. Emission factors were calculated as the proportions of emitted N2O during the experimental period over total applied mineral N that was a sum of applied N as fertilizer and growth media (soil plus organic amendments).
Soil temperature and precipitation monitoring
Soil temperature at 5-cm depth on minute basis was recorded using HOBO® H8 Temp Loggers (Onset Computer Corporation, USA), and precipitation data were obtained from a weather station located in the experimental field.
Statistical analysis
One-way analysis of variance (ANOVA) was carried out to determine the effects of treatments (AS, AS + PM, and AS + SD) on the measured parameters. Least significant difference (LSD) values (α = 0.05) for multiple comparisons were calculated when the treatment effect was significant. All the analyses were performed using StatView 5.0.1.
Results
Blueberry growth, its nitrogen uptake, and distribution of applied mineral nitrogen
The effects of peat moss and sawdust compost applications on blueberry seedling growth were significant over about two-years of cultivation (). Both applications of peat moss and sawdust compost significantly increased shoot dry weights (396 and 406 g plant−1, respectively) as compared with AS treatment (84 g plant−1). Also, incorporation of sawdust compost significantly increased the root dry weight by 3.6 times (610 g plant−1) over AS treatment (166 g plant−1), while 2.8 times increase was observed in AS + PM (470 g plant−1). There was a strong positive correlation (r = 0.992, n = 9) between soil porosity and root dry weight.
Table 2 Total dry weight (shoot and root) of blueberry plants, annual blueberry yields, and total nitrogen (N) uptake of blueberry plant over the experimental period in each treatment
Fresh berry yield was significantly greater in AS + SD treatment (272 g plant−1) as compared with in AS and AS + PM treatments in 2008 (27 and 28 g plant−1, respectively) (). In 2009, AS + PM and AS + SD treatments resulted in greater fresh berry yields (285 and 348 g plant−1, respectively) as compared with AS treatment (93 g plant−1, ).
Throughout the two-growing seasons, the effects of treatments on leaf N concentrations were not significant. The leaf N concentrations in every treatment were lower (9 g N kg−1 on average) than the optimal range of blueberry plant (12–17 g N kg−1) before the first fertilizer N application. The first fertilizer application increased leaf N concentration in all the treatments to the optimal ranges (13 g N kg−1 on average), and blueberry plants did not show any N deficiency symptoms. Leaf N concentration ranged from 11 (in October 2008) to 23 g N kg−1 (in April 2009) on average. The total N uptake of a plant, which was considered as the sum of N contents in shoots, leaves, roots and fruits of a plant and measured after the destructive harvest on October 31, 2009, was significantly higher in AS + PM and AS + SD treatments (12.5 and 11.1 g N) compared with that in AS treatment (3.2 g N, ).
Soil pH and ammonium and nitrate concentrations
The initial soil pH of the soil used in the experiment was 6.3 (). Incorporation of peat moss and sawdust compost with ferrous sulfate before the plantation significantly decreased soil pH to 4.9 and 5.4, respectively (). The first application of (NH4)2SO4 in July 2008 significantly reduced soil pH in AS and AS + PM treatments to 5.3 and 4.3, respectively, but this sharp decrease was not observed in AS + SD treatment. After the disturbance due to fertilizer application, soil pH in AS treatment increased and stayed slightly higher than in AS + PM and AS + SD treatments. Soil pH did not differ among the treatments after the second and third applications of N fertilizer in March and July 2009 but gradually decreased in all the treatments towards the termination of the experiment.
Figure 1 Soil pH changes during the experimental period as affected by organic amendments. AS, ammonium sulfate applied alone; AS + PM, ammonium sulfate applied with peat moss; AS + SD, ammonium sulfate applied with sawdust compost and ferrous sulfate. Bars show least significant difference (LSD) (α = 0.05). Vertical arrows show the timing of nitrogen fertilizer applications.
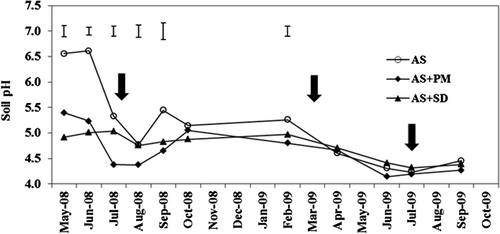
Soil concentrations in all the treatments increased sharply right after the application of (NH4)2SO4 in July 2008 to the values of 46, 142, and 164 mg N kg−1 soil in AS, AS + PM, and AS + SD treatments, respectively (). During two months after the fertilization, soil
concentration decreased and became almost zero by the next fertilization in March 2009 in every treatment. The spring application of (NH4)2SO4 in March 2009 increased the soil
concentrations sharply in all treatments, leading to the maximum soil
concentration of 287 mg N kg−1 soil in AS treatment, followed by AS + PM and AS + SD treatments (205 and 206 mg N kg−1 soil, respectively). The last fertilization in July 2009 increased
concentration in AS treatment to less extent than the first and second fertilizations but did not affect soil
concentration in AS + PM and AS + SD treatments.
Figure 2 Changes in ammonium ion () (a) and nitrate ion (
) (b) concentrations in soils during the experimental period. AS, ammonium sulfate applied alone; AS + PM, ammonium sulfate applied with peat moss; AS + SD, ammonium sulfate applied with sawdust compost and ferrous sulfate. Bars show least significant difference (LSD) (α = 0.05). Vertical arrows show the timing of nitrogen fertilizer applications.
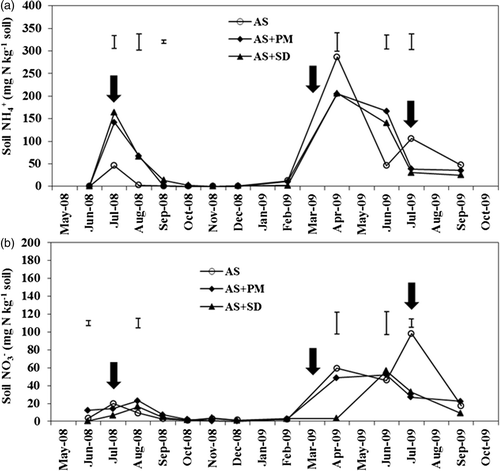
The soil concentration responded more intensively to the second and third fertilizations in March and July 2009 than to the first fertilization in July 2008 (). The AS treatment showed an earlier peak of
(19.7 mg N kg−1 soil) right after fertilizer application in July 2008, whereas AS + PM and AS + SD treatments showed their peaks of soil
concentration one month after fertilization. Afterwards, soil
concentrations declined gradually in all the treatments and became almost zero. Followed by the fertilizer application in March 2009, the
concentration abruptly increased in April 2009 in AS and AS + PM treatments, while AS + SD treatment remained low during April 2009. The
concentration of AS + SD treatment increased significantly in June 2009. Fertilizer application in July 2009 did not significantly increase the
concentrations in AS + SD and AS + PM treatments, while AS treatment resulted in a significantly increased soil
concentration (98.0 mg N kg−1 soil). Soil
concentrations in all the treatments decreased steadily after July 2009.
Losses of nitrogen as nitrous oxide emission and mineral nitrogen leaching
In the initial stage of the cultivation, significant N2O fluxes in AS + SD treatment were observed (). After these events, the major N2O fluxes were recorded after the application of (NH4)2SO4 in July 2008 and March 2009 (), somewhat coincided with large precipitation events and summers with relatively high soil temperatures (). The AS + SD treatments usually showed the least N2O fluxes, except the initial peaks (). The last application of (NH4)2SO4 in July 2009 induced a significant N2O fluxes only in AS treatment (). During the experiment, the AS treatment resulted in the greatest cumulative N2O emission (1.28 g N m−2), followed by AS + PM and AS + SD (0.80 and 0.58 g N m−2, respectively) treatments (). The application of sawdust compost significantly reduced cumulative N2O emission by 25.5 and 73.1% in 2008 (May–December) and 2009 (middle of March to September), respectively, relative to the emission in AS treatment (). Peat moss incorporation also reduced cumulative N2O emission by 14.1 and 52.4% in 2008 and 2009, respectively, even though the effects were less pronounced and insignificant relative to the emission in AS treatment (). Assuming that all the emitted N2O originated from applied (NH4)2SO4 and mineral N in soil and organic amendments, the proportions of lost N as N2O emission over total applied N were 2.4, 1.5, and 1.0% in AS, AS + PM, and AS + SD treatments, respectively, throughout the experimental period ().
Figure 3 Changes in daily nitrous oxide (N2O) fluxes (a), precipitation (b), and soil temperature at 5-cm depth (c) in 2008 and 2009. AS, ammonium sulfate applied alone; AS + PM, ammonium sulfate applied with peat moss; AS + SD, ammonium sulfate applied with sawdust compost and ferrous sulfate. Bars show least significant difference (LSD) (α = 0.05). Vertical arrows show the timing of nitrogen fertilizer applications.
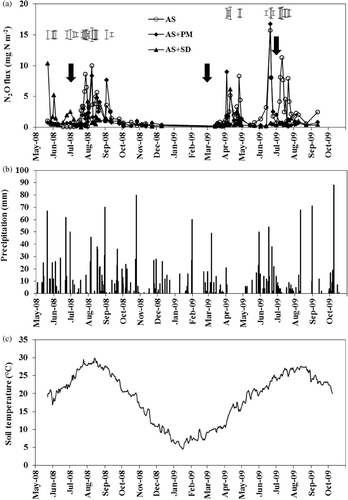
Table 3 Total mineral nitrogen (N) applied to blueberry cultivating soils, cumulative N loss as emitted nitrous oxide (N2O), and emission factors of N2O throughout the experimental period in each treatment
The total precipitation was 1253 mm from May to December in 2008. There were more frequent precipitation events from middle to the end of August in 2008 and from the end of May to the beginning of July in 2009 (). There were no significant differences in the drainage volumes between AS (146.1 mm) and AS + PM (138.2 mm) treatments, while significantly less drainage was recorded in AS + SD (116.9 mm) treatment in 2008. The total precipitation was 1058 mm from January to October in 2009, leading to significantly different cumulative drainage volumes among the treatments (102.2, 88.9, and 82.2 mm in AS, AS + PM, and AS + SD, respectively). The drainage water volume significantly correlated (r = −0.958, n = 9) with the root dry weight of blueberry seedlings. The AS + SD treatment resulted in a large N loss as in leachates in 2008 () that increased rapidly during the first two months after the cultivation establishment in 2008. More than 90% of the total annual N loss as
was lost during this period in AS + SD treatment. In the other treatments, the N losses as
and
gradually increased after the establishment, accompanied with moderate peaks after the N fertilizer application events in 2008 (). In 2009, N losses as
and
in leachates were more significant than those in 2008 (). Particularly, the fertilizer application in March 2009 and frequent rainfall events during June 2009 significantly increased N losses as
and
in leachates. Both N losses as
and
were largest in AS treatment, followed by in AS + PM and in AS + SD treatments (). In 2009, both peat moss and sawdust compost applications successfully reduced cumulative N losses as both forms of
and
in leachates, resulting in 35 and 65%
reduction and 35 and 90% of
reduction by peat moss and sawdust compost applications, respectively, as compared with AS treatment. Assuming that every mineral N losses in leachates originated from applied N fertilizer as (NH4)2SO4 and mineral N in soil and organic amendments, the proportions of lost N as
over total applied N were 26.5, 18.6, and 11.2% in AS, AS + PM, and AS + SD treatments, respectively, and those as
were 27.3, 17.6, and 7.4% in AS, AS + PM, and AS + SD treatments, respectively, throughout the cultivation period. There was a negative correlation relationship between the root weight of blueberry seedlings and
concentrations in leachates (r = −0.975, n = 9).
Figure 4 Cumulative nitrogen (N) losses as ammonium ion () and nitrate ion (
) in leachates in 2008 (a) and 2009 (b). AS, ammonium sulfate applied alone; AS + PM, ammonium sulfate applied with peat moss; AS + SD, ammonium sulfate applied with sawdust compost and ferrous sulfate. Vertical arrows show the timing of nitrogen fertilizer applications.
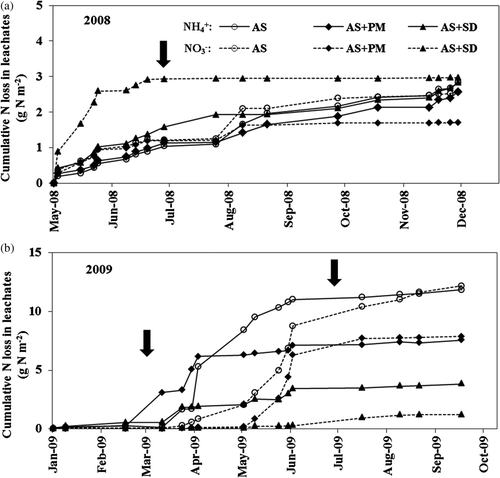
Distribution of applied mineral nitrogen
The proportional distribution of applied mineral N as fertilizers and growth media is shown in . Assuming that the sum of plant uptake and soil retained and
is counted as remaining N, this remaining N was largest in AS + PM treatment, followed by in AS and in AS + SD treatments (). The total amount of lost N as
and
in leachates and N2O emission was largest in AS treatment, followed by in AS + PM and in AS + SD treatments. The AS + SD treatment resulted in the largest unaccounted portion of applied mineral N, followed by AS + PM and AS treatments.
Figure 5 Distribution of mineral nitrogen (N) applied as ammonium sulfate after two-year cultivation of blueberry. AS, ammonium sulfate alone; AS + PM, ammonium sulfate with peat moss; AS + SD, ammonium sulfate with sawdust compost and ferrous sulfate. N2O, nitrous oxide; , ammonium ion;
, nitrate ion.
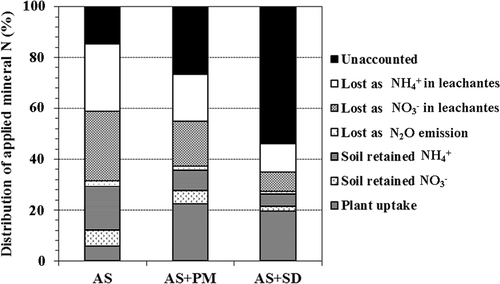
Discussion
Effects of incorporated peat moss and sawdust compost on blueberry growth and nitrogen uptake
Low concentration of available N in growth media () limited plant growth before the first fertilizer application in July 2008, supported by leaf N concentration that increased significantly after the first fertilizer application in line with the findings of Spiers (1982). At the same time, applied N as might have been taken up by the blueberry plants quickly in all the treatments, as indicated by low N losses as leaching and N2O emission ( and ). The applied N in July 2008 was sufficient to fulfill the N requirement for the rest of the growing season since the plants did not show any N deficiency symptoms in this year. Applied
in the third fertilizer application in July 2009 was also assumed to be taken up by plants quickly, as indicated by the small increase in mineral N in leachates and soils ( and ). The blueberry plant did not respond to the second fertilizer application in March 2009 as vigorously as to the summer applications in July 2008 and 2009, as indicated by the more intensive increase in
of soil and leachates, probably due to a lack of deficiency and low temperature in March.
Sawdust compost application improved blueberry growth more effectively than peat moss application () in agreement with previous studies (Haynes and Swift Citation1986; Li et al. Citation2006). There was no N limitation to blueberry in all treatments, judging from the absence of N deficient symptoms and leaf N concentrations; therefore, the main reason for the poor performance of AS treatment was the lack of incorporated organic amendment and the many beneficial effects derived from its application. Organic matter is amended to soils in blueberry cultivation system basically to improve water drainage and other physical properties of soils (Tamada 2009), especially when using soils with low porosity such as Brown Forest Soil (orthic Cambisol) used in this study. A strong positive correlation (r = 0.992, n = 9) between soil porosity and root dry weight suggested the beneficial effect of organic matter incorporation for root extension of blueberry. Our results confirmed the positive effects of pre-planting incorporation of organic amendments on blueberry performance as previously reported by other authors either by incorporation of peat moss (Spiers Citation1986), sawdust (Peterson et al. Citation1987), or other by-products (Banados 2009). However, the interpretation of positive effects of sawdust compost may need cautions since not only the improved soil physical properties but also original high nutrient concentrations in sawdust compost affected the growth of young blueberry seedlings in this study.
Correlations of the root dry weight with drainage volume and with concentration of in leachates indicated that enhanced root growth and its N uptake contribute significantly to reduce
leaching, as indicated by previous studies (Benjamin et al.
Citation1996; Hashimoto et al.
Citation2007), and regulate summer leaching losses in corn field and off-season leaching losses in catch crop field (Vos et al.
Citation1998; Throup-Kristensen Citation2001; Kristensen and Throup-Kristensen Citation2004).
Effects of incorporated peat moss and sawdust compost on soil conditions and nitrogen losses
The sharply decreased soil pH after the first application of (NH4)2SO4 in July 2008 in AS and AS + PM treatments () may be due to a significant uptake by blueberry and nitrification as indicated by the emissions of N2O in AS and AS + PM treatments (). Consequently, soil
concentration significantly decreased after the fertilization with slight increases in
concentration in AS + PM and AS treatments (). The nitirification rate was probably minimal in AS + SD treatments, supported by the absence of soil pH changes and N2O response ( and a), while the initial N2O fluxes and
leaching losses of AS + SD treatment ( and ) were probably derived from the nitrification of the high initial
contained in the sawdust compost (). The later decreases in soil pH in all the treatments after the fertilization in March and July 2009 () may be related with nitrification of applied (NH4)2SO4 since decreased soil
and increased soil
concentrations were observed (). Taking a year after applications of peat moss, ferrous sulfate, and/or
fertilizer, soil pH can reach low ranges (4.0–5.2) enough to offer favorable conditions for blueberry plants (Coville Citation1910).
Cumulative N2O loss shows the significant effects of both sawdust compost and peat moss applications to reduce the N2O emission as compared with AS treatment (). These results could be related with the uptake by blueberry plant, that was facilitated by improved plant growth in peat moss and sawdust compost applied treatments, and the microbial immobilization of the applied N due to the high C:N ratio of peat moss and even higher one in sawdust compost (). The soils in AS treatment, however, contained more mineral N supplied for nitrification and denitrification, with relatively higher pH (). Due to the presence of recalcitrant lignin content in sawdust, its decomposition may require longer time than the cellulose dominated peat moss (Levanon et al. 1998; Gani and Naruse Citation2007); therefore, more prominent immobilization was expected in AS + SD treatment than in AS + PM treatment. This hypothesis was supported by Schipper and Vojvodic-Vukovic (Citation2001) where sawdust was utilized successfully for five years as a denitrification wall to remove nitrate from migrating groundwater in soil. In AS + PM treatment, leaching losses started to increase one month and two months after the fertilizer application in May 2009 for
and
, respectively, and these leaching increases were followed by the same increases for AS + SD treatment (), suggesting a longer period required for decomposition in combination with mineral N immobilization for sawdust compost applied soil than for peat moss applied soil. There was a time lag between the timings of increasing
and
in leachates after fertilization in March 2009 observed in every treatment; the latter increment in
than in
indicated nitirification and consequent leaching of
(). Also, the study of Huang et al. (Citation2004) who found a reduced N2O emission due to microbial immobilization and decreased N availability for N2O emitting microbes supports our hypothesis.
Evidently, the prerequisite for denitrification is the presence of (Bremner Citation1997). Since the applied N was in the
form, both nitrification and denitrification may have contributed to N2O production in this study. Nitrification produces N2O as a by-product (Inubushi et al.
Citation1996), and denitrification produces N2O and N2 through enzymatic reduction of
for ATP synthesis (Bremner Citation1997; Delaune et al. Citation1998). It is not possible to attribute the N2O emission to nitrification and denitrification in this study. As many other studies in different types of ecosystems have shown, the N2O emission in blueberry cultivation system seemed to be affected by mineral N availabilities, precipitation (soil water content), temperature, and probably soil pH. Except for AS + SD treatment that initially contained a certain amount of
in soils, N2O fluxes of AS and AS + PM treatments in the initial stage of the experiment suggested limited N availabilities for nitrifiers and denitrifiers (). The first application of N fertilizer did not lead to an immediate N2O flux probably due to many factors (), including N uptake by blueberry plant and microbial immobilization as mentioned earlier. Also lowered soil pH () might suppress the activities of nitrifiers that originated from Tateyama soil having an original high pH, since nitrifiers such as Nitrobacter and Nitrosomonas are sensitive to low pH (<6.6) and nitrification rate becomes smaller when soil pH decreases further (White Citation2006). The AS treatment that had a relatively higher pH resulted in the largest peaks of N2O fluxes without any precipitation peak in the end of July 2008 (), indicating that nitrification resulted in N2O production. However, the N2O flux peaks in AS and AS + PM treatments from the beginning to the end of August 2008 and June 2009 can be attributed to concomitant frequent precipitation events () and consequent denitrification process since soil
concentrations were relatively high in these periods (), as the relationship between soil moisture and N2O production was pointed out by Papen and Butterbach-Bahl (Citation1999). The amount of annual N2O losses (0.21–0.79 g N m−2) observed in our study was comparable to the findings of Pang et al. (2009) who reported an annual loss of 3.2 kg N2O-N ha−1 in an apple orchard soil that received 311 kg N ha−1 year−1. The absence of N2O fluxes and mineral N losses in leachates from October 2008 to March 2009 ( and 4) indicates minimal nitrification and denitrification rates during this period due to mineral N availabilities and low temperature.
Distribution of applied mineral nitrogen after two-year cultivation of blueberry
Large proportions of applied mineral N were lost over the cultivation period as mineral N in leachates and N2O, especially in AS treatment (). Application of peat moss and sawdust compost successfully increased N uptake of tree plants and decreased the lost N proportions over applied mineral N. In , “unaccounted N” was calculated by subtracting the sum of N distributed to plant, soil, and lost N as leachates and N2O from the total amount of mineral N applied as fertilizer ((NH4)2SO4) and growth media (soil and organic amendments); therefore, this portion, the greatest in AS + SD treatment followed by AS + PM and AS treatments, indicates lost N as N2 gas through denitrification and immobilized N to soil microbes as organic N. As significant N2O fluxes were recorded concomitant with rainfall events (), the used soils in this experiment had chances of denitrification in anaerobic microsites. Other than these occasions, we suspect that denitrification and N2 production were minimal, especially in AS + PM and AS + SD treatments due to the increased porosities by organic amendments (). Therefore, the largest N immobilization might have taken place in AS + SD treatment, followed by AS + PM treatment, leading to reduced N losses as leachates and N2O emission as compared with AS treatment. However, soil microbial biomass and N immobilization rate as affected by organic amendments in blueberry cultivating soils were not directly measured in this study and should be further studied to clarify the relationship between N losses to environment and microbial immobilization.
Conclusions
Incorporation of peat moss and sawdust compost with ferrous sulfate effectively improved soil conditions for blueberry cultivation and resulted in increased growth and yield of blueberry in simulated raised bed cultivation system. The beneficial effects of organic amendment incorporation were mainly to increase soil porosity and plant root biomass. Concurrently, the application of organic amendments decreased N losses as mineral N in leachates and N2O emission during two-year of blueberry cultivation, probably due to increased plant N uptake and microbial N immobilization. More detailed studies to clarify the effects of organic amendments and better establishments are necessary to establish sustainable and environmentally friendly cultivation system of upland blueberry.
Acknowledgments
The authors thank Dr Kazunori Sakamoto and Dr Mohammad Zaman for their constructive suggestions, Professor Tetsuo Anzai for providing soil samples, Dr Oslan Jumadi and Dr Silvio Y. Ushiwata for their practical help, and Dr Crisanta Bueno from IRRI for editing this manuscript.
References
- Anderson , JM and Ingram , JSI . 1989 . “ Colorimetric determination of ammonium ” . In Tropical Soil Biology and Fertility , Edited by: Anderson , JM and Ingram , JSI . 42 – 43 . Wallingford : CAB International .
- Anzai , T . 2001 . “ Chapter 12: Soil classification and investigation ” . In Introduction to Soil Science , Edited by: Inubushi , K and Anzai , T . 93 – 108 . Tokyo (in Japanese) : Asakura Publishing .
- Aulakh , MS , Doran , JW , Walters , DT , Mosier , AR and Francis , DD . 1991 . Crop residue type and placement effects on denitrification and mineralization . Soil Sci. Soc. Am. J. , 55 : 1020 – 1025 .
- Banados , MP . 2009 . Expanding blueberry production into non-traditional production areas: northern Chile and Argentina, Mexico and Spain . Acta Hort. , 810 : 439 – 444 .
- Benjamin , JG , Ahuja , LR and Allmaras , RR . 1996 . Modelling corn rooting patterns and their effects on water uptake and nitrate leaching . Plant Soil , 179 : 223 – 232 .
- Bernal , MP , Alburquergue , JA and Moral , R . 2009 . Composting of animal manures and chemical criteria for compost maturity assessment. A review . Bioresour. Tech. , 100 : 5444 – 5453 .
- Bremner , JM . 1997 . Sources of nitrous oxide in soils . Nutr. Cycl. Agroecosys. , 49 : 7 – 16 .
- Bugbee , JG . 1999 . Effects of hardwood sawdust in potting media containing biosolids compost on plant growth, fertilizer needs, and nitrogen leaching . Commun. Soil Sci. Plant Anal. , 30 : 689 – 698 .
- Correa , RS , White , RE and Weatherley , J . 2005 . Modelling the risk of nitrate leaching from two soils amended with five different biosolids . R. Bras. Ci. Solo , 29 : 619 – 626 .
- Coville , FV . 1910 . Experiments in Blueberry Culture, USDA Bureau of Plant Industry Bulletin 193 , Washington, DC : US Department of Agriculture .
- Cummings , GA , Mainland , CM and Lilly , JP . 1981 . Influence of soil pH, sulfur and sawdust on rabbiteye blueberry survival, growth and yield . J. Am. Soc. Hort. Sci. , 106 : 783 – 785 .
- Darnell , RL , Stutte , GW , Martin , GC , Lang , GA and Early , JD . 1992 . Developmental physiology of rabbiteye blueberry . Horticulture Reviews , 13 : 339 – 406 .
- Daum , D and Schenk , MK . 1998 . Influence of nutrient solution pH on N2O and N2 emissions from a soilless culture system . Plant Soil , 203 : 279 – 287 .
- Delaune , RD , Lindau , CW , Suaema , E and Jugsujinda , A . 1998 . Nitrification and denitrification estimates in a Louisiana swamp forest soil as assessed by 15N isotope dilution and direct gaseous measurements . Water Air Soil Pollut , 106 : 149 – 161 .
- Di , HJ and Cameron , HC . 2002 . Nitrate leaching in temperate agroecosystems: sources, factors and mitigation strategies . Nutr. Cycl. Agroecosys. , 46 : 237 – 256 .
- Eck , P . 1988 . Blueberry Science , 135 – 169 . New Brunswick, NJ : Rutgers University Press .
- Gani , A and Naruse , I . 2007 . Effect of cellulose and lignin content on pyrolisis and combustion characteristics for several type of biomass . Renewable Energy , 32 : 649 – 661 .
- Gough , R . 1994 . The Highbush Blueberry and its Management , 132 – 133 . New York : Food Products Press .
- Green , CJ and Blackmer , AM . 1995 . Residue decomposition effects on nitrogen availability to corn following corn or soybean . Soil Sci. Soc. Am. J. , 59 : 1065 – 1070 .
- Hashimoto , M , Hirai , Y , Nagaoka , T and Kouno , K . 2007 . Nitrate leaching in granitic regosol as affected by N uptake and transpiration by corn . Soil Sci. Plant Nutr. , 53 : 300 – 309 .
- Hayashi , A , Sakamoto , K and Yoshida , T . 1997 . A rapid method for determination of nitrate in soil by hydrazine reduction procedure . Jpn. J. Soil Sci. Plant Nutr. , 68 : 322 – 326 (in Japanese with English summary) .
- Hayler , U . 2006 . Blueberries for Growers, Gardeners, Promoters , 237 – 238 . Gainesville, FL : E.O. Painter Printing Company, Inc. Dr Norman F. Childers Publications .
- Haynes , RJ . 1986 . Laboratory study of soil acidification and leaching of nutrients from a soil amended with various surface-incorporated acidifying agents . Fertilizer Research , 10 : 165 – 174 .
- Haynes , RJ and Swift , RS . 1986 . Effect of soil amendments and sawdust mulching on growth, yield and leaf nutrient content of high bush blueberry plants . Sci. Hortic.- Amsterdam , 29 : 229 – 238 .
- Huang , Y , Zou , J , Zheng , X , Wang , Y and Xu , X . 2004 . Nitrous oxide emissions as influenced by amendments of plant residues with different C N ratios . Soil Biol. Biochem. , 36 : 973 – 981 .
- Inubushi , K , Naganuma , H and Kitahara , S . 1996 . Contribution of denitrification and autotrophic and heterotrophic nitrification to nitrous oxide production in andosols . Biol. Fertil. Soils , 23 : 292 – 298 .
- Jumadi , O , Hala , Y , Muis , A , Ali , A , Pallenari , M , Yagi , K and Inubushi , K . 2008 . Influences of chemical fertilizers and a nitrification inhibitor on greenhouse gas fluxes in a corn (Zea mays L.) field soil in Indonesia . Microbes Environ. , 23 : 29 – 34 .
- Kristensen , HL and Throup-Kristensen , K . 2004 . Root growth and nitrate uptake of three different catch crops in deep soil layers . Soil Sci. Soc. Am. J. , 68 : 529 – 537 .
- Levanon , D , Danai , O and Masaphy , S . 1988 . Chemical and physical parameters in recycling organic wastes for mushroom production . Biological Wastes , 26 : 341 – 348 .
- Li , T , Tang , X , Wu , L and Zhang , Z . 2006 . Effects organic material on soil properties, plant growth, leaf photosynthesis, nutrient uptake and mycorrhizal infection of blueberries . Acta Hort. , 715 : 375 – 380 .
- Ministry of Agriculture, Forestry and Fisheries (MAFF) 2008: Annual Report on Trends in Forest and Forestry. http://www.rinya.maff.go.jp/j/kikaku/hakusyo/20hakusho/pdf/20_e.pdf
- Ministry of Construction Japan . 1998 . Annual Statistics of Water Treatment and Related Activities , 48 – 49 . Tokyo : Ministry of Construction Japan .
- Mogge , B , Kaiser , EA and Munch , JC . 1999 . Nitrous oxide emissions and denitrification N-losses from agricultural soils in the Bronhoved-lake region: influence of organic fertilizers and land use . Soil Biol. Biochem. , 31 : 1245 – 1252 .
- Mosier , AR . 1994 . Nitrous oxide emissions from agricultural soils . Fertilizer Research , 37 : 191 – 200 .
- Obara , H , Ohkura , T , Takata , Y , Kohyama , K , Maejima , Y and Hamazaki , T . 1995 . Comprehensive soil classification system of Japan, first approximation . Bul. Nat. Inst. Agro-Environ. Sci. , 29 : 1 – 73 .
- Pang , J , Wang , X , Mu , Y , Ouyang , Z and Liu , W . 2009 . Nitrous oxide emissions form an apple orchard soil in the semiarid Loess Plateau of China . Biol. Fert. Soils , 46 : 37 – 44 .
- Papen , H and Butterbach-Bahl , K . 1999 . A 3-year continuous record of nitrogen trace gas fluxes from untreated and limed soil of a N-saturated spruce and beech forest ecosystem in Germany 1. N2O emissions . J. Geophys. Res. , 104 : 487 – 503 .
- Peterson , DV , Mullins , CA , Lietzke , DA and Deyton , DE . 1987 . Effects of soil-applied elemental sulfur, aluminium sulfate, and sawdust on growth of rabbiteye blueberries . J. Am. Soc. Hort. Sci. , 112 : 612 – 616 .
- Rappold , C and Crawford , JW . 1999 . The distribution of anoxic volume in a fractal model of soil . Geoderma , 88 : 329 – 347 .
- Schipper , LA and Vojvodic-Vukovic , M . 2001 . Five years of nitrate removal, denitrification and carbon in a denitrification wall . Wat. Res. , 35 : 3473 – 3477 .
- Shelp , ML , Beauchmap , EG and Thurtell , GW . 2000 . Nitrous oxide emissions from soil amended with glucose, alfalfa, or corn residues . Commun. Soil Sci. Plant Anal. , 31 : 877 – 892 .
- Spiers , JM . 1982 . Seasonal variation of leaf nutrient composition in ‘Tifblue’ rabbiteye blueberry . J. Am. Soc. Hort. Sci. , 107 : 255 – 257 .
- Spiers , JM . 1986 . Root distribution of ‘Tifblue’ rabbiteye blueberry as influenced by irrigation, incorporated peatmoss, and mulch . J. Am. Soc. Hort. Sci. , 111 : 877 – 880 .
- Strik B, Finn CE, Hart J, et al. 1993: Highbush blueberry production. Pacific Northwest Extension Bulletin 215. Oregon State University, Corvallis, OR
- Strik , B and Hart , J . 1991 . Fertilizer Guide. Blueberries. Oregon State University Extension Service Publication, FG78 , Corvallis, OR : Oregon State University .
- Sugiyama , N and Hanawa , S . 1992 . Growth responses of rabbiteye blueberry plants to N forms at constant pH in solution culture . Soil Sci. Plant Nutr. , 61 : 25 – 29 .
- Tamada , T . 2004 . Effects of nitrogen sources on growth and nutrient concentrations of ‘Tifblue’ rabbiteye blueberry under water culture . Small Fruit Review , 3 : 149 – 158 .
- Tamada , T . 2009 . Current trends of blueberry culture in Japan . Acta Hort. , 810 : 109 – 116 .
- Taquini , IL . 2006 . Blueberries for Growers, Gardeners, Promoters , 257 – 259 . Gainesville, FL : E.O. Painter Printing Company, Inc. Dr Norman F. Childers Publications .
- Throup-Kristensen , K . 2001 . Are differences in root growth of nitrogen catch crops important for their ability to reduce soil nitrate-N content, and how can this be measured? . Plant Soil , 230 : 185 – 195 .
- Toma , Y and Hatano , R . 2007 . Effect of crop residue C:N ratio on N2O emissions from Gray Lowland soil in Mikasa, Hokkaido, Japan . Soil Sci. Plant Nutr. , 53 : 198 – 205 .
- US Environmental Protection Agency (EPA) . 1995 . a. 40 CFR 403 and 503 amendments to standards for the use or disposal of sewage sludge: Final rule , 314 – 356 . Washington, DC : US EPA .
- Vos , J , Van Der Putten , PEL , Hussein , MH , Van Dam , AM and Leffelaar , PA . 1998 . Field observations on nitrogen catch crops. II. Root length and root distribution in relation to species and nitrogen supply . Plant Soil , 201 : 149 – 155 .
- White , RE . 2006 . “ Chapter 8: Soil Aeration ” . In Principles and Practice of Soil Science – the Soil as a Natural Resource , 4th , Edited by: White , RE . 158 – 175 . Cambridge, MA : Blackwell Publishing .
- Wilber , WL and Williamson , JG . 2008 . Effects of fertilizer rate on growth and fruiting of containerized southern highbush blueberry . Hort. Sci. , 43 : 143 – 145 .
- Williamson , J , Krewer , G , Pavlis , G and Mainland , CM . 2006 . Blueberries for Growers, Gardeners, Promoters , 60 – 74 . Gainesville, FL : E.O. Painter Printing Company, Inc. Dr Norman F. Childers Publications .