Abstract
The root interaction between neighboring plants is neither obvious nor well studied because there are no easy and convenient methods to distinguish the root system among individual plants. Previously, we developed a method of pressure–injection of dye into the roots of pot-grown plants in dry soil condition. Here, we modified the method for high-pressure injection in wet soil or outdoor fields to counter root pressure. In our preliminary pot experiment, the roots of tomato plants (Lycopersicon esculentum “Momotaro”) were stained well in wet soil by dye injection at 0.5 MPa. In the field test, tomato plants were grown in a 2 m × 2 m concrete frame field. Four successive plant shoots were cut 159 and 170 days after sowing, and dye solutions (Fantasy) of different colors were pressure-injected into the roots from the stumps from 0.05 to 0.5 MPa. The volume of the dye and the time required for staining were respectively in the ranges of 120 to 150 mL and 9 to 96 h. Soil blocks or cores were sampled and the roots were collected by hand picking and the water floating method, and distinguished by color. The recognition and collection of roots were easy because most of them were stained dark. In the 0–15 cm soil layer, most of the roots in the four blocks surrounding the hills came from the hills. In blocks found at the intermediate position between two adjacent plants, the roots of both plants were distributed. In the 15–30 cm soil layer, most of the roots in the four blocks surrounding the hills came from the hills, although roots of other plants also existed. The roots of each plant extended to adjacent plants, even reaching distant plants. The roots of a plant did not grow toward another plant in the opposite row. Findings of this study are useful in relation to effective fertilizer application. The proposed injection method is expected to facilitate the study of the interactions between root systems in the field.
Introduction
In natural or agricultural vegetation, plants do not stand alone but grow with neighboring plants. They interact with each other in various ways through their shoots and roots including competition for light (e.g. Zimdahl Citation2004) and soil resources (Aspinall Citation1960; Baldwin Citation1976; Caldwell et al. Citation1985, Citation1991; Wilson Citation1988), growth retardation by allelopathy (Rice Citation1984), and cooperation through natural root grafts (Graham and Bormann Citation1966; Atsatt Citation1970). Information on root interaction is useful for understanding plant life or developing agricultural techniques, such as planting at optimal density and mixed cropping. However, such information cannot be obtained easily because roots cannot be observed directly and cannot be discriminated easily owing to their similarity in shape and/or color even among different species. Various methods such as the separation of roots according to differences in color or shape (Katayama et al. Citation1996), the isotope labeling of roots (Litav and Harper Citation1967, Bookman and Mack Citation1982) and the direct mapping of root location (Caldwell et al. Citation1991, Van Rees et al. Citation1994), have been proposed to overcome these constraints, but they are laborious.
Previously, we developed a simple method of staining root systems in pot culture by dye injection and demonstrated its validity by performing a quantitative analysis of root distribution (Murakami et al. Citation2006). Using this method, Ikenaga et al. (Citation2009) clarified the more rapid root growth of wheat and barely than soybean in mixed cropping in a small container 24–30 days after sowing (DAS), Miyazawa et al. (Citation2010) clarified the difference in root distribution between sole cropping and intercropping of green manure crops in a non-woven fabric bag 42 DAS. Thus, the staining method is essential for clarifying the relationship between the roots of two or more plants.
However, the container or bag method has limitations for root growth, particularly in the late growth stage. Staining roots in the field is essential to solve the problem, but this is difficult because of exudation of xylem sap caused by root pressure. Roach (Citation1939) reported the difficulty in staining tree roots under the high-soil-moisture condition. Therefore, we modified the previous method for high-pressure injection, verified the staining condition in a wet soil in a preliminary pot experiment and demonstrated the root distribution of field-grown tomato using the new method.
Materials and Methods
Modification of equipment for high-pressure injection
Plant culture
Two tomato seedlings (Lycopersicon esculentum var. Momotaro) 14 DAS were planted 4 cm apart at the center of a 10-cm-diameter polyethylene pot (two pots were used; the inner pot was transparent, the outer pot, black) that was filled with culture soil [Kureha Co., Tokyo; nitrogen/phosphorus/potassium/magnesium, 0.4 : 0.8 : 0.5 : 0.1 g kg−1; maximum water holding capacity (MWHC), 0.56 g g soil−1]. The plants were grown in a glasshouse with conventional irrigation. Soil moisture content was set at four levels by controlling irrigation 0–9 days before staining; driest, dry, wet, and wettest. Four replications (two pots) were made for each condition. The experiment was conducted three times, namely, 80, 84, and 100 DAS.
Root staining
Under the two dry conditions, staining was carried out using the previously proposed equipment (Murakami et al., Citation2006). Under the remaining two wet conditions, the modified system for high pressure injection, which consists of pressure-resistant tubes, two-way stopcocks, plastic pipettes, and a nitrogen gas tank, was employed (). The tube for fitting to the plant stem (stem tube) was made of polyurethane (resisting pressure, 0.8 MPa) and 120 mm in length, with an inner diameter in the range of 4 to 6 mm; it was equipped with a tube connector at the upper end (). The pipette was 10 mL in volume, with a transparent tape that was wound around the pipette three times to prevent it from bursting. The tube connector was sealed with paste at the lower end, the circumference was further reinforced with epoxy adhesives, and the upper end was equipped with a two-way stopcock. The stopcock was connected to the regulator on the nitrogen gas tank with a pressure-resistant polyurethane tube with an inner diameter of 6 mm and a pressure resistance of 0.8 MPa.
Figure 1. Dye injection system for pot (a) and field (b). (a) a, Two dry treatments with low-pressure injection system; b, two wet treatments with high-pressure injection system; c, Y-type connector; d, two-way stopcock; e, plastic pipette; f, dye (Fantasy); g, stem tube; h, wire; i, pot; j, pesticide sprayer; k, regulator; l, gas tank; m, pressure- tolerant tube; n, stand. (b) o, Hose band.
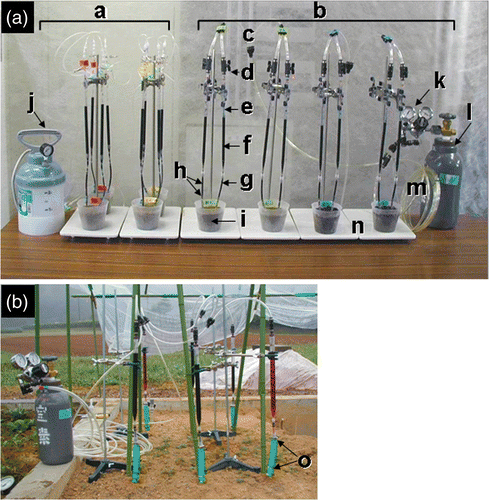
Figure 2. Pipette and stem tube: a, pipette 50 mL volume; b, pipette and connector; c, pipette 10 mL volume; d, tube connectors in stem tube; e and f, stem tubes with hose band for thick stem; g and h, stem tubes for thin stem.
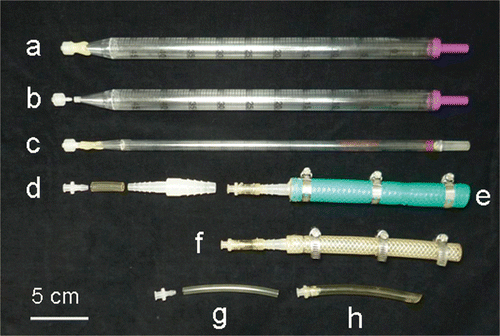
The shoots were cut at 4 cm above the ground. The stem was plastered with vacuum grease, fitted with a stem tube (soft vinyl type for the two dry conditions, polyurethane for the two wet conditions). The tube and the stem were secured with a paraffin film strip, and the upper and lower portions of the stem were tied with wire bands or hose bands. Then, the outer black pot was removed to allow observation of the soil. After the pipette and the tube were joined with connectors, dyes of different colors (Fantasy, for cut flower staining, Palace Chemical Co., Ltd, Yokohama) were added into each pipette, and air in the tube was expelled by pumping or vibrating manually. Initially, the dye was pressurized at 0.05 MPa for all the conditions. After 6 h, for the two wet conditions under which dye consumption was low, pressure was increased to 0.5 MPa. This maximum pressure was employed as determined on the basis of preliminary tests, which is a rational choice because the highest root pressure reported is approximately 0.6 MPa in excised tomato root in culture solution (White Citation1938) and it is in the range 0.15–0.38 MPa under field condition (Araki and Tsuji Citation2005). Dye consumption was recorded every 60 min (15–30 min during the first 1 h) until 8–10 mL of the dye was consumed or 24 h had passed. The injection volume was decided on the basis of a previous report (Murakami et al. 2006). After staining, the stained roots were washed and separated by the water floating method described in a previous report. The percentage of stained roots was determined visually and root volume was determined using a measuring cylinder. Soil mass water content was determined before root washing by desiccation of two 5 g subsample at 105°C for 24 h.
Root staining of tomato in concrete-framed field
Plant culture
Tomato seedlings grown in 10-cm-diameter polyethylene pots with culture soil 70 DAS were transplanted to the center of a concrete-framed field (2 m × 2 m) at the National Agricultural Research Center for Tohoku region (Fukushima city) at a hill space of 24 cm and a row space of 80 cm on June 17, 2005 as shown in . This planting density is the smallest for commercial production and is convenient for root sampling. The field was filled with Haplic Andosol (soil texture: CL (Sand; 43.7%, Silt; 36.7% and Clay; 19.6%), MWHC: 0.79 g g soil−1) to 1 m depth and the bottom was exposed to the ground. Before transplanting, 150 kg nitrogen ha−1, 65 kg phosphorus ha−1, 124 kg potassium ha−1, and 2000 kg calcium ha−1 were dressed as basal fertilizer. Twenty-one days before staining, the field was covered with a vinyl roof to make the soil dry as much as possible.
Root staining
Root staining was replicated two times; 159 DAS (September 13) and 170 DAS (September 24). As shown in , for 159 DAS, the arrangement of dyes was white (not stained), red, blue, and white from left to right in row S (corresponding to south position), and white, lavender, green, and white in row N (corresponding to north position). For 170 DAS (), the arrangement of dyes was red, green, lavender, and white in row S, and lavender, blue, red, and white in row N. Root staining was carried out using the apparatus described earlier (). The shoot of four successive plants in the two rows were cut at 6 cm above the ground and allowed to stand for 24 h to reduce the exudation of xylem sap. The cutting was practiced at 17:00 p.m. on September 12 (28.3°C, fine day) and 8:00 a.m. on September 23 (22.0°C, fine day). The stumps were fitted with stem tubes (hose equipped with the tube connector at the top, 15 cm in length, ). The stem and the tube were tied with steel hose bands. The earlier-mentioned 50 mL pipette was connected to the stem tube and a dye was filled in. Then, the air in the stem tube was expelled by pumping manually. At the beginning, the dye was pressurized at 0.05 MPa using the nitrogen gas cylinder. After 24 h, pressure was increased step by step (0.1 MPa at a time) when dye consumption rate decreased. The pressure was maintained at 0.5 MPa until 150 mL of the dye was consumed or 96 h had passed. The volume of the dye used was determined as follows: average shoot weight × root/shoot ratio (8.6%, Jackson and Bloom Citation1990) × factor (2.4–3.8, Murakami et al. Citation2006).
Root collection and analysis
After completion of the staining, the 72 cm × 32 cm × 30 (depth) cm soil near the hills was collected by dividing into 36 blocks (8 cm × 8 cm × 15 cm) each in the 0–15 cm and 15–30 cm soil layers with steel plates and a knife. Soil cores (5 cm diameter × 15 cm in each soil layer) were also collected with a hand auger at 12 cm intervals 40 cm away from the hills (). After the soil collection, the soil was dug to a depth of 1 m to observe root distribution and the staining condition. The roots were collected by a previously reported method with modification, that is, the roots were separated into thick (more than 1 mm diameter) and fine roots prior to length determination. Thick-root length was determined directly with a curve meter and fine-root length, with the modified line intersection method (Murakami et al. Citation1999). Soil moisture content was determined at intervals of a depth of 10 cm by desiccation of two 5 g sample at 105°C for 24 h.
Results
Root staining in pot experiment
The relationships among dye consumption, soil moisture content, pressure of injection, and staining situation were essentially the same in the three experiments (80 DAS, 84 DAS, and 100 DAS); therefore, we show here the results of 100 DAS as representative. The percentages of MWHC under the four conditions (i.e. driest, dry, wet, and wettest) were 27, 29, 68 and 86%, respectively. At 27% MWHC, the shoots were almost dried up, 29% MWHC, the shoots were wilted, and at 68 and 86% MWHC, the plants were vigorous. The shoot dry weight, root dry weight, root volume and root length were substantially the same under all conditions, as shown in . The relationships among dye consumption, soil moisture content, and injection pressure are shown in . At 27% MWHC, 3.32 mL of the dye was consumed at 24 h and 70–80% of the roots were stained dark; at 29% MWHC, 5.48 mL of the dye was consumed at 24 h and whole roots except root tips were stained dark. The reason for the lower volume of dye consumed at 27% MWHC than at 29% MWHC was that probably the stem was wilted and part of the roots died, thus hindering penetration of the dye. At 68% MWHC, 0.08 mL of the dye was consumed at 6 h. After the application of 0.5 MPa pressure, dye consumption was increased and 7.75 mL of the dye was consumed at 24 h; three of four plants were stained dark but remaining plants were stained light. At 86% MWHC, first, xylem sap exuded and the volume of the dye consumed was –0.39 mL at 6 h. However, after the application of 0.5 MPa pressure, 6.85 mL of the dye was consumed at 24 h and 50–70% of the roots were stained light. The reason for the light color is the dilution of the dye by the water from the surrounding soil. In the 68 and 86% MWHC, some roots were stained by the other dye that eluted from the roots of other plants. From these findings, it was concluded that the soil should be as dry as possible so that the plant wilts, although a pressure of 0.5 MPa enables root staining even if the soil is wet.
Figure 4. Relationship among dye consumption, soil moisture content and injection pressure in roots of pot-grown tomatoes 100 days after sowing. Results are expressed as means of two pots ± standard deviation (bars). Values following legends indicate percentage of maximum water holding capacity. Injection pressure was 0.05 MPa initially in all treatments. In both 68% and 86% treatments, the pressure was increased to 0.5 MPa at 6 h (indicated by an arrow).
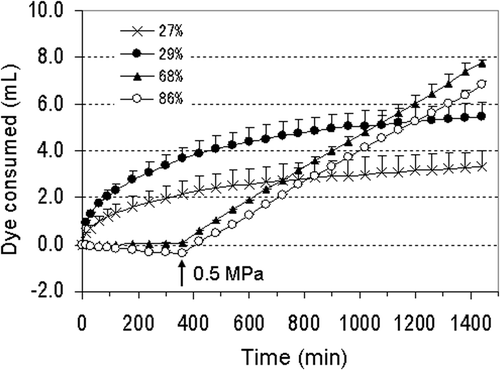
Table 1. Relationship between soil moisture content and root staining condition in pot experiment 100 days after sowing†
Tomato root distribution in field
Here, we show the results for 170 DAS because the two staining replications (159 DAS and 170 DAS) showed substantially the same results. The discrimination and collection of roots were easy because most of them were stained dark, although many residual roots of weeds existed. The soil was driest (30% MWHC) at the 0–20 cm layer and wettest (58%) at the 30–40 cm plow pan layer, which is similar to the neighboring outdoor filed. The moisture content was within the range obtained in the preliminary pot experiment (27–86%). The light staining of roots was observed at a maximum depth of 58 cm. The root length densities of the block-sampled area (72 cm × 32 cm) were 3.95 ± 3.28 cm cm−3 at the 0–15 cm soil layer and 0.96 ± 0.42 cm cm−3 at the 15–30 cm layer (mean with standard deviation). The root length density in the surface layer was four-fold that in the lower layer (n = 72, p < 0.0001). While those of cores (ϕ5 × 15 cm) were 0.09 ± 0.07 cm cm−3 at the 0–15 cm soil layer and 0.06 ± 0.05 cm cm−3 at the 15–30 cm layer (n = 18, not significant). The average shoot fresh weight of the eight plants excluding their fruit was 497 ± 93 g (mean with standard deviation), and maximum weight was 642 g. The average plant height was 156 ± 8 cm and the stem diameter was 11.6 ± 0.8 mm.
shows the percent root length in soil blocks and cores from the two rows of tomato plants. The bar and pie graphs correspond to the sampling positions of soil. There was little difference in root distribution between the two rows. In the 0–15 cm soil layer, most roots in the four blocks surrounding the hills came from the hills. This is expected because plants having many roots were transplanted at those positions. In the four blocks at the intermediate positions between two adjacent plants, the roots of both plants were well distributed. This trend agreed with that observed in the container experiment (Murakami et al. Citation2006). In the 15–30 cm soil layer, most of the roots in the four blocks surrounding the hills came from the hills, although roots of other plants also existed. In the blocks at the intermediate positions between the two plants, not only the roots of the two plants but also those from plants located far away also existed. The roots of each plant extended to adjacent plants, even reaching distant plants. Note that the unstained roots (indicated as white) found at locations far from the unstained hills in the 0–15 cm soil layer are most likely due to error, judging from the range of root distribution of the other stained plants. However, in the 15–30 cm soil layer, the white roots may contain both of unstained roots due to error and roots from the unstained hill far from the block. The data of core are also useful to know the root distribution although the precision is slightly lower than the block data. The roots of plants in both rows reached to the area 40 cm apart from the hill, but did not extend to the opposite row in the both soil layer. In this experiment, previously stained roots (red, in row S, green in row N, 159 DAS) were not detected in the left area in both soil layers.
Figure 5. Percent root length of each soil block and core in field-grown tomatoes 170 days after sowing. Each bar or pie graph is arranged to correspond to the sampling position. The colors of the graphs correspond to the dye colors. The circles with letters in the graphs indicate positions of the hills and the dye colors. Row S corresponds to the south position, row N corresponds to the north position. R is red, G is green, L is lavender, B is blue and W is white (no dye).
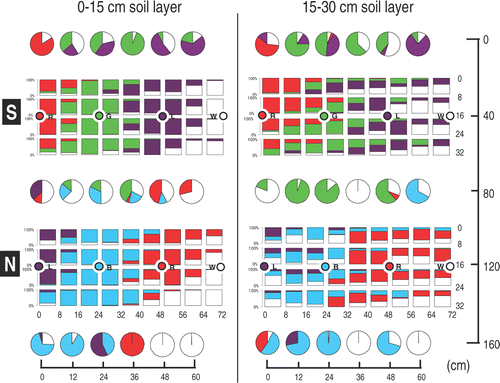
The percent root dry weight in the soil blocks showed a similar trend to the percent root length because the (root length)/(root dry weight) ratios of the blocks did not vary much (data not shown). The ratio of residual root length with respect to the total root length for each color in each block was 26.1 ± 28.5% on average (n = 381). The collection of residual roots is necessary for determining the exact root length.
shows the curves of the volumes of dyes consumed in the field. The volumes of dyes consumed were large during the first 15 h and decreased thereafter. When the pressure was increased from 0.05 to 0.1, 0.2, 0.3, 0.4 and 0.5 MPa, the volumes of dyes consumed increased. Four of six plants consumed 150 mL of dye from 53 to 82 h. In the north plot, 120 mL of lavender dye was consumed at 96 h, whereas in the south plot, 150 mL of the lavender dye was consumed within 9 h. This rapid consumption was thought to be caused by leakage from a crack in the tap root, because soaking of the soil surface with the dye was observed. This, however, had little effect on root collection; as shown in , the distribution of roots stained by the lavender dye was similar to that stained by the other dyes.
Figure 6. Relationship between dye consumption and injection pressure in each tomato plant in field (170 days after sowing). The injection pressure was 0.05 MPa initially. Arrows with figures indicate changing points of the pressure (MPa). The symbol S corresponds to the south position, N corresponds to the north position.
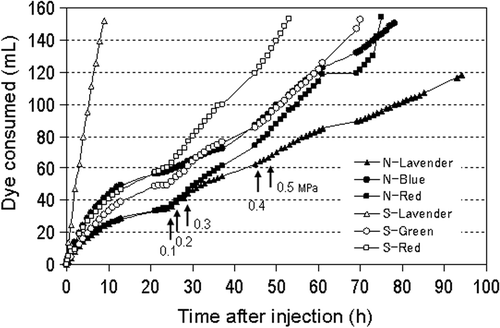
Discussion
There are several studies of root distribution in tomato plants in the field (Weaver and Bruner Citation1927; Spencer Citation1951; Jackson and Bloom Citation1990); however, most of them focused on a single plant root system. The proposed method enabled the demonstration of the root distribution in a plant community, as shown in . The obtained data are expected to contribute to improving fertilizer placement or irrigation position. For example, when a fertilizer is applied at positions between any adjacent plants in the same row, as shown in , the fertilizer can be possibly absorbed by both plants. Also, a fertilizer applied in the inter-row area can be absorbed by the plants on both sides. It may reduce the number of top dressing points and labor.
The staining method could be used to improve the efficiency of root collection by expediting the discrimination of roots from organic matter or residual roots, to facilitate physiological studies, to supply some nutrients directly to the shoots in order for them to recover from a deficiency, and to apply pesticides for controlling soil borne diseases, because various kinds of chemicals can be injected other than dyes. Bacterial wilt is one of the persistent diseases because the pathogen (Pseudomonas solanacearum) survives in the deep soil zone for several years (Okabe Citation1969). If a pesticide is supplied to the zone by injection through the root system, bacterial wilt may be controlled. The method can be used to estimate the extent of the allelopathy effect, because the rooting area can be easily detected by finding stained roots. Note that excess amount of dye leaks from the roots to the soil, but it does not cause serious problems because the dye is not toxic.
The staining was confirmed in the field using eggplant (Solanum melongena L.), common lamb's quarters (Chenopodium album L. var. album), horse weed (Erigeron Canadensis L.) as well as tomato. In soybean (Glycine max Merr.), the roots were not stained well because the stem was easily broken by the high-pressure injection and the color of the stained root was not clear. The staining of monocot roots has not yet been examined; however, it is probably difficult because their stem is formed by the overlapping of leaf sheaths; the dye solution would therefore leak easily from the gap between the sheaths during high-pressure injection.
In conclusion, the method of injecting pressurized dye at 0.05 to 0.5 MPa can stain intact root systems in the field and will contribute to facilitating the study of root interactions.
Acknowledgments
The authors wish to thank Mr N. Yamashita for support in the preliminary tests of root staining, Mr R. Shishido, Mr T. Sakurai and Mr A. Ogasawara for assistance in plant growth, Mr N. Izawa for making the root collecting plate, and Ms M. Saito for help in root analysis. This work was partly supported by a Grant-in-Aid for Scientific Research (No. 17380196) from the Japan Society for the Promotion of Science.
References
- Aspinall, D , 1960. An analysis of competition between barley and white persicaria , II Factors determining the course of competition. Ann. Appl. Biol. 48 (1960), pp. 637–654.
- Araki, H , and Tsuji, W , 2005. Evaluation of root pressure of legumes and vegetables in various soil conditions , Root Research 14 (2005), p. 180, (abstract of 23rd Root Research Meeting, in Japanese).
- Atsatt, PR , 1970. "Biochemical bridges between vascular plants". In: Kenton, L , ed. Proc. of the 29th Annual Biology Colloquium . Corvallis, OR: Oregon State University; 1970. pp. 53–68.
- Baldwin, JP , 1976. Competition for plant nutrients in soil; a theoretical approach , J. Agric. Sci. Camb. 87 (1976), pp. 341–356.
- Bookman, PA , and Mack, RN , 1982. Root interaction between Bromus tectorum and Poa pratensis: a three dimensional analysis , Ecology 63 (1982), pp. 640–646.
- Caldwell, MM , Eissenstat, DM , Richards, JH , and Allen, MF , 1985. Competition for phosphorus: differential uptake from dual-isotope-labeled soil interspaces between shrub and grass , Science 229 (1985), pp. 384–386.
- Caldwell, MM , Manwaring, JH , and Durham, SL , 1991. The microscale distribution of neighbouring plant roots in fertile soil microsites , Functional Ecology 5 (1991), pp. 765–772.
- Graham, BF , and Bormann, FH , 1966. Natural root grafts , The Botanical Review 32 (1966), pp. 255–292.
- Ikenaga, S , Murakami, T , Kobayashi, H , Yamashita, N , Yoshino, N , and Uchida, T , 2009. Root distribution of soybean in living mulch cultivation with winter-type barley or wheat , Root Research 18 (2009), pp. 15–19, (in Japanese with English summary).
- Jackson, LE , and Bloom, AJ , 1990. Root distribution in relation to soil nitrogen availability in field-grown tomatoes , Plant Soil 128 (1990), pp. 115–126.
- Katayama, K , Adu-Gyamfi, J , Gayatri, D , Rao, TP , and Ito, O , 1996. "Root system development of component crops in intercropping". In: Ito, O , et al., eds. Roots and Nitrogen in Cropping Systems of the Semi-arid Tropics . Tsukuba: JIRCAS; 1996. pp. 199–209.
- Litav, M , and Harper, JL , 1967. A method for studying spatial relationships between the root systems of two neighbouring plants , Plant Soil 26 (1967), pp. 389–392.
- Miyazawa, K , Murakami, T , Takeda, M , and Murayama, T , 2010. Intercropping green manure crops-effects on rooting patterns , Plant Soil 331 (2010), pp. 231–239.
- Murakami, T , Shimano, S , Kaneda, S , Nakajima, M , Urashima, Y , and Miyoshi, N , 2006. Multicolor staining of root systems in pot culture , Soil Sci. Plant Nutri. 52 (2006), pp. 618–622.
- Murakami, T , Yamada, K , and Yoshida, S , 1999. Improved method for easy and rapid determination of root length of vegetables , Soil Sci. Plant Nutr. 45 (1999), pp. 471–478.
- Okabe, N , 1969. Population changes of Pseudomonas solanacearum and soil microorganisms in artificially infested natural field soils , Bulletin of the Faculty of Agriculture, Shizuoka University 19 (1969), pp. 1–29, (in Japanese).
- Rice, E , 1984. Alellopathy, . London: Academic Press; 1984.
- Roach, WA , 1939. Plant injection as a physiological method , Ann. Bot. N. S. 3 (1939), pp. 155–226.
- Spencer, K , 1951. Tomato root distribution under furrow irrigation , Aust. J Agric. Res. 2 (1951), pp. 118–125.
- Van Rees, KCJ , Hoskins, JA , and Hoskins, WD , 1994. Analyzing root competition with Dirichlet tessellation for wheat on three landscape positions , Soil Sci. Soc. Am. J. 58 (1994), pp. 423–432.
- Weaver, JE , and Bruner, WE , 1927. "Tomato". In: Root Development of Vegetable Crops . New York: McGraw-Hill; 1927. pp. 241–262.
- White, PR , 1938. “Root-pressure” – an unappreciated force in sap movement , Am. J. Bot. 25 (1938), pp. 223–227.
- Wilson, JB , 1988. Shoot competition and root competition , J. Appl. Ecology 25 (1988), pp. 279–296.
- Zimdahl, RL , 2004. Weed–Crop Competition, . Ames, IA: Blackwell Publishing; 2004. pp. 136–139.