Abstract
We investigated the effect of increasing soil temperature and nitrogen on greenhouse gas (GHG) emissions [carbon dioxide (CO2), methane (CH4) and nitrous oxide (N2O)] from a desert steppe soil in Inner Mongolia, China. Two temperature levels (heating versus no heating) and two nitrogen (N) fertilizer application levels (0 and 100 kg N ha−1 year−1) were examined in a complete randomized design with six replications. The GHG surface fluxes and their concentrations in soil (0 to 50 cm) were collected bi-weekly from June 2006 to November 2007. Carbon dioxide and N2O emissions were not affected by heating or N treatment, but compared with other seasons, CO2 was higher in summer [average of 29.6 versus 8.6 mg carbon (C) m−2 h−1 over all other seasons] and N2O was lower in winter (average of 2.6 versus 4.0 mg N m−2 h−1 over all other seasons). Desert steppe soil is a CH4 sink with the highest rate of consumption occurring in summer. Heating decreased CH4 consumption only in the summer. Increasing surface soil temperature by 1.3°C or applying 100 kg ha−1 year−1 N fertilizer had no effect on the overall GHG emissions. Seasonal variability in GHG emission reflected changes in temperature and soil moisture content. At an average CH4 consumption rate of 31.65 µg C m−2 h−1, the 30.73 million ha of desert steppe soil in Inner Mongolia can consume (sequestrate) about 85 × 106 kg CH4-C, an offset equivalent to 711 × 106 kg CO2-C emissions annually. Thus, desert steppe soil should be considered an important CH4 sink and its potential in reducing GHG emission and mitigating climate change warrants further investigation.
Introduction
World-wide increases in greenhouse gas (GHG) emissions are influenced by agriculture and other anthropogenic activities (Moore and Dalva Citation1993; Mosier et al. Citation1996; Loro et al. Citation1997). Within the agricultural sector, nitrogen (N) fertilizer application has contributed the most to increases in GHG emission as N influences plant growth, carbon (C) and N mineralization/immobilization, biological activity, and conversion of plant residue into soil organic matter (Hyde et al. Citation2006; Maljanen et al. Citation2006; Al-Kaisi et al. Citation2008). However, there are conflicting reports on nitrous oxide (N2O) and carbon dioxide (CO2) emission from, and methane (CH4) consumption by, soil following N fertilizer application (Kowalenko et al. Citation1978; Sitaula et al. Citation2000; Lampe et al. Citation2006), which may be explained by soil moisture content (Liu et al. Citation2008) and temperature (Moore and Dalva Citation1993).
Nitrous oxide emissions generally increase as a result of denitrification following an increase in soil moisture content (Maag and Vinther Citation1996; Schaufler et al. Citation2010) as soil becomes more anaerobic. The soil moisture content has a better relationship with CO2 and CH4 emission (Schaufler et al. Citation2010), it has been found that the CO2 emission was higher between 20 and 60% water-filled pore space, while CH4 uptake was highest at driest soil moisture condition, and was negatively correlated with soil moisture content.
Increasing temperature resulted in an exponential increase in N2O emission and linear increase in CH4 consumption on a shortgrass steppe (Mosier et al. Citation1996) while CO2 emissions increased 25–40% when soil temperature increased by 5°C (Rustad and Fernandez Citation1998). Similar emission increases were reported by Zhou et al. (Citation2006) in upland tallgrass prairie soil and Lang et al. (2011) in grassland soils. Greenhous gas emission could also be impacted indirectly by temperature increases (Gregorich et al. Citation2006) by influencing soil nutrient interactions (Mosier Citation1998) and availability (Hyde et al. Citation2006). Increases in soil temperature are generally associated with a decrease in moisture content (Harte et al. Citation1995) but, in some cases, warming may increase soil moisture content through accelerated canopy senescence and reduced transpiration losses (Zavaleta et al. Citation2003).
World-wide grassland occupies about 24% of the land area and contributes to 17% of total agricultural GHG emissions (Schils et al. Citation2005). In China, grasslands occupy about 400 million ha. Of this, 78.8 million ha is located in Inner Mongolia with 30.7 million ha (39%) as desert steppe (Han et al. Citation2008).
The desert steppe in Inner Mongolia is the most arid and least productive grassland in China. It has experienced an increase in air temperature of 0.2°C per decade from 1959 to 1994 (Han Citation2002). Even if GHG concentrations in the atmosphere were to remain at the year 2000 level, increases of about 0.1°C per decade could still be expected (IPCC Citation2007). Thus, we need to develop management practices that reduce GHG emission and mitigate global climate change.
Most research has focused on the effects of global warming on forestry and rice fields with few studies examining the effects on semi-arid natural grasslands. Others have examined GHG emissions from semi-arid grassland focusing on the effect of different management systems (Mosier et al. Citation1991; Mosier et al. Citation1998; Holst et al. Citation2007). The effects of increased temperature and N fertilizer application have rarely been studied in the arid desert steppe environment where soil processes are severely constrained by limited soil moisture content. Thus, the objectives of this research were to investigate the effect of increasing temperature and N input on GHG emission from a desert steppe. We hypothesized that increasing temperature and N input affects fluxes of CO2, CH4 and N2O, but the effects would be different on the GHG emission. Increasing temperature and N input would increase CO2 and N2O emissions, while they would have no effects on the CH4 emissions.
Materials and Methods
Site description
The experiment was conducted from June 2006 to November 2007 in Siziwang Banner, Inner Mongolia, China (41°46′43.6″ N, 111°53′41.7″ E; elevation 1456 m) in the desert steppe. The dominant species are Stipa breviflora Griseb., Artemisia frigida Willd and Cleistogenes songorica (Roshev.) Ohwi. The mean vegetation canopy cover ranged from 17 to 20%. Sheep had grazed the experimental site year-round with the stocking rate at less than 1 sheep ha−1 year−1 prior to 1988. The grassland had no previous fertilizer application and hydrological manipulations prior to our experiment. In the 1990s, the stocking rate in this region was increased to about 1.5 sheep ha−1 year, leading to some degradation reflected in a reduction of average vegetation height from about 10 cm to 5 cm (unpublished data).
The climate is continental with a mean annual precipitation of 183 mm. Over 50% of the precipitation occurs from June to August. Mean annual air temperature is 3.4°C with a 175 day frost-free period. Annual precipitation in 2006 (161 mm) and 2007 (162 mm) was about 88% of the annual mean with most (115 mm in 2006 and 90 mm in 2007) occurring during the growing season (June to August; ). The mean annual air temperatures in 2006 (4.0°C) and 2007 (4.1°C) were above the long-term average.
Figure 1. Monthly precipitation (bars) and mean monthly air temperature (line) from January 2006 to December 2007 in a desert steppe. Data was measured at a micro-meteorological station located adjacent to the experimental site.
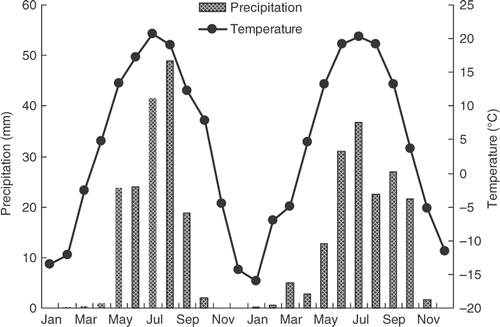
The soil is a Kastanozem [Food and Agricultural Organization (FAO) soil classification] with a sandy loam texture (FAO-UNESCO 1988), pH 8.6 in the surface soil (0–10 cm), increasing to 8.9 at 20–30 cm. Soil organic C and total N was 13.7 and 1.45 g kg−1, respectively, at the soil surface and decreased to 9.70 and 1.01 g kg−1, respectively, at 20–30 cm soil depth. Soil bulk density averaged 1.42 g cm−3 in the 0 to 10 cm depth.
Experimental design
The effect of increased soil temperature (by heating) and N enrichment was examined in a 2 (heating versus no heat) × 2 (0 versus 100 kg N ha−1 fertilizer application) randomized complete split-plot factorial design with six replications. The main plots (3 m × 4 m) were either heated continuously (H1) using infrared radiators (Kalglo Electronics, Bethlehem, PA, USA, power: 2000 watts) or left unheated as a control (H0). The main plots were split into two sub-plots (3 m × 2 m in size) with one sub-plot receiving 100 kg N ha−1 year−1 fertilizer application [F1 ammonium nitrate (NH4NO3)] and the other receiving no N fertilizer (F0). In order to simulate enhanced N deposition, the fertilizer application rate was approximately twice as much as the current N deposition rate (Lü and Tian Citation2007). The fertilizer was broadcast once each year during the rainy season (July) and coordinated with a high probability of rain. A total of 11.2 mm precipitation in six rain events occurred within 12 days following fertilizer application in 2006, and 9 mm precipitation in nine rain events occurred within 12 days in 2007. The heating equipment was mounted 2.25 m aboveground to cover the main plot area. A “dummy” heater with the same shape and size but no heating source was also mounted above the H0 treatment. The heater increased surface soil temperature on average by 1.3°C, an increase that could be expected as a result of global warming within the current century.
Soil sampling and analyzes
Soil samples were collected from each sub-plot at the beginning of the study (May 2006) and again when the GHG experiment ended (November 2007). Samples were taken at 0–10, 10–20 and 20–30 cm in 2006, and 0–5, 5–15, 15–30 and 30–60 cm depths in 2007. Soil samples were air-dried and coarsely ground to pass a 2-mm sieve mesh; sub-samples were ground to pass a 0.150-mm size sieve. These fine ground soil samples were analyzed for total C (TC) and total N (TN) concentrations using a gas chromatography mass spectrometer (GC-MS CNS) analyzer (Carla Erba® Italy). Inorganic C was measured using a Varian GC-3400 analyzer (Varian Instruments, Walnut Creek, CA). The method was reference to the modified method by Amundson et al. (Citation1998). Soil organic carbon (SOC) is the difference between TC and inorganic C. Coarsely ground samples were analyzed for available N [ammonium nitrogen (-N) and nitrate nitrogen (
-N)], measured by the alkali diffusion method (Khan et al.
Citation1997) in 2006 and the potassium chloride (KCl)-extractable method in 2007. In the alkaline method, the available N is liberated by diffusion through 1 M sodium hydroxide (NaOH) from a 2-g soil sample and determined by titration with 0.005 M sulphuric acid (H2SO4), the available N can be calculated by the amount of titration solution. For the KCl-extraction methods, 2 M KCl at 1:5 solid to liquid ratio was shaken for 1 h, filtered and the concentration in the extract measured using a Bran+Luebbe AutoAnalyzer III (Bran + Luebbe, Germany). The values of available N obtained in 2006 were converted to equivalent values of
-N and
-N using a regression equation developed between the two based on data (12 data points) from a nearby field.
The coarsely ground soil samples were also used to determine pH and electrical conductivity (EC) from a 1:5 solid to liquid ratio in water. The soil–water slurry was measured with a PHS-3D pH meter (Shanghai Precision and Scientific Instrument Co. Ltd, Shanghai, China) and a DDSJ.308A EC meter (Shanghai Precision and Scientific Instrument Co. Ltd, Shanghai, China). Soil bulk density was measured in May 2006 before the experiment started. Undisturbed soil cores from each plot were collected in the surface 0–5 cm using steel rings (5 cm diameter) (Drewry et al. Citation1999).
Soil moisture content, temperature and gas measurements
Soil moisture content, temperature and gases were determined in three out of six randomly selected replicates for each treatment at approximately bi-weekly intervals over the duration of the experiment. Soil moisture content sensors (HOBO® model S-SMA-M003, ONSET Computer Corporation, Pullman, WA) were installed at three depths (10, 20 and 30 cm) horizontally in six of 12 main plots (three heated and three no heat) in May 2006 and in the other six plots in May 2007. Soil moisture content readings were logged hourly with a data logger (HOBO® Weather Station Data Logger, model H21-001, ONSET Computer Corporation, Pullman, WA), and downloaded to a computer at two-week intervals.
Temperature and gases were sampled at the soil surface (0 cm) and at 7.5, 15, 30 and 50 cm below the surface. Temperature readings and gas samples below the soil surface were obtained using a depth sampler as described by Hao et al. (Citation2001). Briefly, a carrying tube with gas lines and temperature sensor inside and positioned at required depths was installed into the soil profile. Temperature readings were obtained every two weeks using the thermocouples (Digital Omega HH – 25TC, Omega Technology, Stamford, CT). Gas samples were taken without further disturbing soil at the same time temperature readings were taken. A digital thermocouple thermometer was used to measure surface soil temperatures.
Surface gas flux was measured using vented static chambers at opposite corners of the sub-plot. Chamber bases (circular polypropylene collar, 8 cm high and 10.5 cm diameter) were installed 6 cm into the soil, leaving 2 cm above the surface. During sampling, chambers with a volume of 1798 cm3 (22 cm high by 10.2 cm diameter), were attached to the chamber base. At 0, 10, 20, 30 and 60 min after the chamber placement, 11 mL gas samples were drawn through a septum from the chamber headspace and immediately injected into pre-evacuated vials (Exetainer, Labco Ltd, Buckinghamshire, UK). During the winter months, snow was brushed off the soil surface before surface flux gas collection.
Gas samples from different soil depths (0, 7.5, 15, 30 and 50 cm) were taken at the same time when surface flux samples were taken. Vials containing gas samples were shipped to the Agricultural and Agri-Food Canada, Lethbridge Research Center via express air mail for analysis. Possible leakage from the vials during shipping was checked using vials filled with standard gas and shipped between the experimental site in China and the laboratory in Canada. Gas samples were analyzed for CO2, CH4 and N2O using a gas chromatograph (Varian 3800, Varian Instruments, Walnut Creek, CA) equipped with an electron capture detector (ECD), thermal conductivity detector (TCD) and flame ionization detector (FID).
Data handling and statistical analyzes
Average daily soil moisture content, temperature, and TN, organic C, -N and
-N concentrations were analyzed using mixed linear models (PROC MIXED, SAS Institute Inc. 2008). Heating, N application, soil depth and their interactions were the fixed effects while replication and its interaction with heating and soil N treatment were the random effects. Soil depth was treated as a repeated variable and analyzed using the covariance structure type that produced the lowest AIC value.
The GHG data were calculated by fitting a second-order polynomial equation to the GHG concentration versus time, and flux levels were calculated by taking derivatives of the second-order polynomial equation (Hao et al. Citation2001). The GHG flux data were converted into mg or µg m−2 h−1 and pooled for each season [defined as spring (March to May), summer (June to August), autumn (September to November), and winter (December to February)]. The cumulative annual GHG emissions over the study period were calculated based on bi-weekly flux measurements and assuming that the hourly rates, measured at the time, were representative of the two-week interval. These estimates were summed over the study period and expressed as kg ha−1 year−1.
The effects of heating, soil N application, season and their interactions on average gas concentrations and gas flux rates were analyzed as previously described for soil properties. Heating, soil N application, season and their interactions were the fixed effects while replication and its interactions with heating and season were the random effects. When treatment effects were significant (P < 0.05), means were separated using least significant difference. Linear regression analyzes were used to investigate the relationship between surface GHG flux and soil temperature and soil moisture content.
Results
Soil responses
The soil temperatures at the surface (0 cm) and in the soil profile (7.5, 15, 30 and 50 cm) ranged from −10 to 40°C. The average temperature in the H1 treatment was 1.3 and 0.9°C warmer (P < 0.05) at the surface and sub-surface (7.5–50 cm) than the H0 treatment ().
Table 1. Average desert steppe soil temperature (in °C) in heating (H1) and no heating (H0) treatment over the study period in different soil depth
Neither heating nor N fertilizer application had any effect on soil TN, organic C, nitrate ion () and ammonium ion (
) concentrations after two years of treatment (P > 0.05). However, the average
-N and
-N concentrations in the H1 were 10.5 and 7.8% lower than in H0 treatment. The SOC and TN concentrations were highest in the surface soil, decreased significantly with soil depth and reached their lowest values at 30 to 60 cm depth. Soil
and
concentrations from 0 to 30 cm were also higher than from 30 to 60 cm (P < 0.05).
The average soil moisture varied from 0.001 to 0.090 m3 m−3, with the surface soil moisture not affected by heating treatment. A significantly higher subsoil moisture content was observed with H1 (0.08 m3 m−3) than the H0 control (0.06 m3 m−3) (). Soil moisture was also higher in spring and summer than in autumn, with the value in winter being the lowest at all soil depths.
Greenhouse gas distribution in soil profile
The CO2 concentration in the soil profile was not affected by heating and N applications, but was affected by season (S), depth (D) and their interactions (S × D). The CO2 concentration in the soil profile was low (360–410 µL L−1) and varied little in the soil profile during the winter months. The CO2 concentration peaked during the summer for all treatments, increasing from 448 µL L−1 at the soil surface to 1174 µL L−1 at 50 cm. In addition, the lowest CO2 concentration occurred at the surface and increased with soil depth ().
Table 2. Average carbon dioxide (CO2), methane (CH4) and nitrous oxide (N2O) concentration (in µL L−1) in soil profile (0, 7.5, 15, 30 and 50 cm) in different seasons over the study period in a desert steppe†
Methane concentration in the soil profile was not affected by N application, but was affected by heating (H), season (S), depth (D) and the S × D interaction. Methane concentration in the soil profile decreased sharply with soil depth in all treatments, and was higher in winter than the other three seasons at soil depths below 7.5 cm ().
The N2O concentration in the soil profile was not affected by heating, N application, season or any interactions among them, but was affected by the sampling depth. The N2O concentration was < 0.340 µL L−1 throughout the whole experimental period (). The N2O concentration in summer was lower at the surface (0.319 µL L−1) than at all other depths (0.322–0.324 µL L−1) but not significantly different among soil depths for the other three seasons.
Greenhouse gas surface flux
The CO2 emissions over the entire study period varied from 0 to 67 mg C m−2 h−1 (), and were affected by season (S) and the interaction of season and N (S × F). The average CO2 emission of 29.6 mg C m−2 h−1 was higher (P < 0.05) in summer (25.6–33.6 mg C m−2 h−1) than all other seasons (average 8.6 mg C m−2 h−1, range 4.2–14.2 mg C m−2 h−1). Heating had no effect (P > 0.05) on CO2 emission, while the CO2 emission was higher from F0 control than F1, but only in summer (). Cumulative annual CO2 emissions were not affected (P > 0.05) by heating or N application ().
Figure 3. The surface flux of greenhouse gases (a) carbon dioxide (CO2), (b) methane (CH4) and (c) nitrous oxide (N2O) from a desert steppe soil in relation to heating and nitrogen fertilizer treatments (H1F0, heating no fertilizer application; H1F1, heating fertilizer application; H0F0, no heating no fertilizer application; H0F1, no heating fertilizer application).
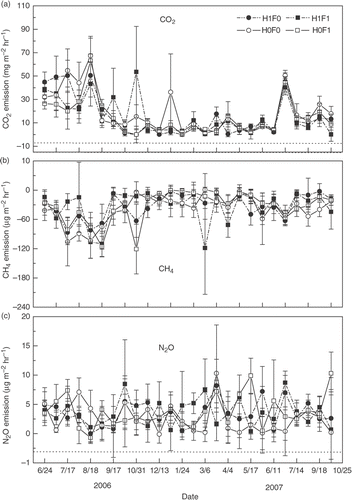
Table 3. The effects of heating, soil nitrogen level, season and their interactions on seasonal greenhouse gas flux rates over the whole study period from a desert steppe soil†
Table 4. Annual rate of carbon dioxide (CO2), methane (CH4) and nitrous oxide (N2O) emissions over the experimental period from the desert steppe soil†
Soil CH4 surface flux exhibited seasonal variations that ranged from –120.8 µg C m−2 h−1 to 0.9 µg C m−2 h−1 (average –34.8 µg C m−2 h−1) over the study period (). The negative values indicate CH4 consumption while positive values indicate CH4 emissions. Of the 104 CH4 surface flux data points collected, 103 were negative (consumption) and only one was positive (emission). The one positive CH4 flux value was consistent with the high CH4 concentration in soil profile at that time. The concentration at 50 cm depth was higher than at 30 cm which was responsible for the observed one time emission of CH4. On average, maximum CH4 consumption occurred during the summer. Methane consumption in summer was reduced (P = 0.057) by the heating treatment. In contrast, in spring CH4 consumption in the heated treatment was double that of the unheated treatment (). Methane consumption was not affected (P > 0.05) by N application. Nevertheless, the cumulative annual CH4 consumption/emission was not affected by either heating or N application ().
The N2O emissions varied from −0.7 to 10.3 µg N m−2 h−1 () but there was no consistent pattern for the four treatments. Heating, N application and their interactions had no significant effect (P > 0.05) on the cumulative annual N2O emissions (). The overall cumulative N2O emission was about 0.3 kg N ha−1 year−1.
Effects of soil temperature and soil moisture content on greenhouse gas flux rates
Soil temperature and moisture had a positive effect (P < 0.05) on CO2 emission and CH4 consumption. However, the N2O emission was unaffected (P > 0.05) by either factor (). Soil moisture content explained greater variation in CO2 (20%) and CH4 flux (9%), while soil temperature explained less (16% for CO2 and 6% for CH4, respectively) ().
Table 5. Relationships between mean daily greenhouse gas (GHG) flux rates and mean daily soil temperature (7.5 cm), moisture (10 cm) over the two year study period in the desert steppe
Discussion
Effects of nitrogen fertilizer on greenhouse gas emissions
Nitrogen fertilizer application stimulates microbial activity, which produces N2O and CO2 production in the soil (Abbasi and Adams Citation2000; Mosier et al. Citation1996; Maljanen et al. Citation2006). The lack of a response of N2O and CO2 emissions from soils in our study indicates that the primary constraint to biological activity in desert steppe soil is not N fertilizer, it maybe correlated with soil moisture content (Yao et al. Citation2010). We did not observe reduced CO2 emissions that might be associated with depressed microbial activity, which could have resulted from lower soil moisture (Yao et al. Citation2010).
The lack of CH4 emission response to N fertilizer application in our study was inconsistent with the results of Mosier et al. (Citation1996) who reported decreased CH4 consumption with N application in a shortgrass steppe. The observations of Mosier et al. (Citation1996) were the result of methanotroph activities in soil being inhibited by and
(Reay and Nedwell Citation2004). The relatively low N application rates and more xeric soil environmental conditions of our study apparently did not produce the conditions that would inhibit methanotroph activities. Also, the weak response of N2O emission on the N application reflected the lower use efficiency of N fertilizer in relative xeric soil condition. Most N fertilizer maybe lost via volatilization.
Effects of soil moisture content on greenhouse gas emissions
Our observation that soil moisture content below 10 cm depth increased with heating was consistent with that reported by Zavaleta et al. (Citation2003). A possible explanation may be that heating increases plant senescence, which reduces water demand by the plants thus reducing water losses from transpiration. This argument is supported by a lower canopy cover (unpublished data) under heating observed in our study.
The largest CO2 concentrations in the soil profile occurred in summer, corresponding to the highest precipitation and soil moisture content. High soil moisture content promotes the production of CO2 in soil (Schaufler et al. Citation2010), which is particularly important in the desert steppe where soil moisture content levels are low most of the time. The increases in CO2 emission, following each precipitation event in summer months, suggests that there is a positive relation between soil moisture content and CO2 production, similar to upland tallgrass prairie soil reported by Zhou et al. (Citation2006).
Carbon dioxide emissions are strongly related to soil moisture content and temperature (Gregorich et al. Citation2006; Liu et al. Citation2008). Soil moisture content affects gas diffusion and microbial activity in the soil (Mosier et al. Citation1996). In the desert steppe, soil moisture content was a more important factor affecting GHG emission than soil temperature as indicated by a higher regression coefficient and greater slope coefficient ().
Methane consumption is linked with soil moisture content (Liu et al. Citation2008). In our study, heating accelerated snowmelt in spring, which promoted microbial activity and increased CH4 consumption (Liu et al. Citation2008). The result was consistent with Schaufler et al. (Citation2010) in the laboratory incubation study, which concluded that soil moisture content has a positive effect on CH4 consumption. The inhibition of CH4 consumption in the summer appears to be caused by an increase of soil moisture content (Le Mer and Roger Citation2001) that was caused by precipitation.
The relatively low N2O concentration in the soil profile and surface emission in our study reflects the weak denitrification and nitrification activity in soil, constrained by very low soil moisture content (Mosier et al. Citation1998; Abdalla et al. Citation2009). This was further confirmed by the simultaneous occurrence of low N2O concentration and low moisture content in the soil profile of our study. Our results are consistent with those reported by Liu et al. (Citation2010) from an Leymus chinensis typical steppe in Inner Mongolia, China.
Effects of soil temperature on greenhouse gas emissions
Similar to N fertilizer application, heating had no consistent effect on GHG surface emissions or the distribution of their concentrations in the soil profile of the desert steppe. As discussed earlier, GHG emissions are primarily the result of microbial activity, which is limited by soil moisture content conditions in the desert steppe. In our study, the lack of response (P > 0.05) to heating might be due to the relatively small temperature increases, which resulted in minimal impact on mineralizable N and C, or on the relatively xeric environment that impedes mineralization. The high CO2 emission in summer observed in our study corresponded to high microbial (Rustad and Fernandez Citation1998) and root activities (Rastogi et al. Citation2002) when seasonally higher precipitation and temperature occurs. Zhou et al. (Citation2006) reported a 13% increase in CO2 emissions after elevating the soil temperature by 2°C in a more mesic upland tallgrass prairie (annual precipitation is ∼915 mm).
Our study showed that increasing soil temperature by heating had no effect on CH4 consumption, which is consistent with the study results of Lang et al. (Citation2010) in grassland soil. We confirmed again that CH4 production is less temperature-dependent in grassland soil. This may be the result of the narrow temperature changes between different heat treatments (Lang et al. Citation2010). On average, the CH4 consumption rate was 31.65 µg C m−2 h−1 from the desert steppe soil in Inner Mongolia. Mosier et al. (Citation1996) observed that soil temperature had relatively little effect (r 2 < 0.30) on CH4 consumption in the shortgrass steppe where average annual precipitation was about 350 mm. At a rate of 31.65 µg C m−2 h−1 CH4 consumption, the 30.73 million ha desert steppe soil in Inner Mongolia could consume (sequestrate) about 85 × 106 kg CH4-C annually. This is equivalent to offset 711 × 106 kg CO2-C emission annually since CH4 global warming potential is 23 times that of CO2. Thus, the desert steppe soil should be considered as an important CH4 sink and its potential in mitigating climate change warrant future investigation.
The small variations (ranging from 0.319 to 0.338 µL L−1) in N2O concentration in the soil profile reflects low microbial activity at low soil moisture content (0.001 to 0.090 m3 m−3). The N2O emissions from the desert steppe, which averaged 3.28 µg N m−2 h–1, are lower than for meadow grassland (∼5.7 µg N m−2 h−1) and forest (290 µg N m−2 h−1) soil (Ullah et al. Citation2005; Kanerva et al. Citation2007), but greater than that reported for shortgrass steppe soil (1.9 µg N m−2 h−1; Mosier et al. 1997). The highest N2O emission occurred during the spring, most likely when freeze–thawing occurred (). Freeze–thaw action can increase the availability of SOC, which promotes microbial activity and increases N2O emission (Edwards and Killham Citation1986). Freeze–thaw also enhances nitrification and hence produces more nitrates for denitrification (Mosier et al. Citation1996). The relatively low annual N2O emission from the desert steppe suggests that it is not a major contributor to global emissions and that global warming is not likely to significantly alter its contribution.
Conclusion
We could not detect any effect from warming or applying N fertilizer on GHG emissions from desert steppe soil. The variations in GHG emission associated with occurrence of precipitation events suggests that soil moisture is one of the most limiting factors affecting microbial activities that produce the GHG while temperature may influence indirectly through its impact on soil moisture. Thus, increasing soil temperature by 1.3°C or applying 100 kg ha−1 year−1 N fertilizer had no significant effect on the overall GHG emissions. Our result rejected the hypothesis that heating and N application will increase GHG emission.
Acknowledgments
The funding for this research was provided by the Agriculture and Agri-Food Canada (AAFC), the National Natural Science Foundation of China (No. 30860060, 30590382), “sustainable utilization for grassland resources” innovation team (NDTD2010-5) and the Science and Technology Supporting Program (No. 2008BAD95B03), P. R. China. The authors gratefully acknowledge the scholarship to the senior authors by China Scholarship Council, Ministry of Education, P. R. China and the technical support from Greg Travis, Pam Caffyn, Brett Hill and Clarence Gilbertson in gas and soil analysis at the AAFC Lethbridge Research Centre. Special thanks to Alata and Baoyin Hexige for their dedication in carrying out the fieldwork and students from Inner Mongolia Agricultural University in collection of field samples and laboratory analyzes, and Dr Jim Moyer, Chi Chang and Mark S. Goettel for helpful suggestions and comments for the revised manuscript.
References
- Abbasi, MK , and Adams, WA , 2000. Gaseous N emission during simultaneous nitrification-denitrification associated with mineral N fertilization to a grassland soil under field conditions , Soil Biol. Biochem. 32 (2000), pp. 1251–1259.
- Abdalla, M , Jones, M , Smith, P , and Williams, M , 2009. Nitrous oxide fluxes and denitrification sensitivity to temperature in Irish pasture soils , Soil Use Manage. 25 (2009), pp. 376–388.
- Al-Kaisi, MM , Kruse, ML , and Sawyer, JE , 2008. Effect of nitrogen fertilizer application on growing season soil carbon dioxide emission in a corn–soybean rotation , J. Environ. Qual. 37 (2008), pp. 325–332.
- Amundson, RG , Trask, J , and Pendall, E , 1998. A rapid method of soil carbonate analysis using gas chromatography , Soil Sci. Soc. Am. J. 52 (1998), pp. 880–883.
- Drewry, JJ , Lowe, JAH , and Paton, RJ , 1999. Effect of sheep stocking intensity on soil physical properties and dry matter production on a Pallic Soil in Southland , N. Z. J. Agric. Res. 42 (1999), pp. 493–499.
- Edwards, AC , and Killham, K , 1986. The effect of freeze/thaw on gaseous nitrogen loss from upland soils , Soil Use Manage. 2 (1986), pp. 86–91.
- FAO-UNESCO 1988: Revised Legend, Soils Map of the World, World Soil Resources Reports 60. International Soil Reference Centre, Food and Agriculture Organization (FAO), Rome.
- Gregorich, EG , Hopkins, DW , Elberling, B , Sparrow, AD , Novis, P , Greenfield, LG , and Rochette, P , 2006. Emission of CO2, CH4 and N2O from lakeshore soils in an Antarctic dry valley , Soil Biol. Biochem. 38 (2006), pp. 3120–3129.
- Han, G , 2002. Influence of precipitation and air temperature on primary productivity of Stipa klemenzii plant community, NeiMongol , Acta Scientiarum Naturalium Universitatis Neimongol 33 (2002), pp. 83–88, (in Chinese with English summary).
- Han, G , Hao, X , Zhao, M , Wang, M , Ellert, BH , Willms, W , and Wang, M , 2008. Effect of grazing intensity on carbon and nitrogen in soil and vegetation in a meadow steppe in Inner Mongolia , Agric. Ecosyst. Environ. 125 (2008), pp. 21–32.
- Hao, X , Chang, C , Carefoot, JM , Janzen, HH , and Ellert, BH , 2001. Nitrous oxide emissions from an irrigated soil as affected by fertilizer and straw management , Nutr. Cycling Agroecosyst. 60 (2001), pp. 1–8.
- Harte, J , Torn, MS , Chang, FR , Feifarek, B , Kinzig, AP , Shaw, R , and Shen, K , 1995. Global warming and soil microclimate: results from a meadow-warming experiment , Ecol. Appl. 5 (1995), pp. 132–150.
- Holst, J , Liu, C , Bruggemann, N , Butterbach-Bahl, K , Zheng, X , Wang, Y , Han, S , Yao, Z , Yue, J , and Han, X , 2007. Microbial N turnover and N-oxide (N2O/NO/NO2) fluxes in semi-arid grassland of Inner Mongolia , Ecosystems 10 (2007), pp. 623–634.
- Hyde, BP , Hawkins, MJ , Fanning, AF , Noonan, D , Ryan, M , O’Toole, P , and Carton, OT , 2006. Nitrous oxide emissions from a fertilized and grazed grassland in the south east of Ireland , Nutr. Cycling Agroecosyst. 75 (2006), pp. 187–200.
- Intergovernmental Panel on Climate Change (IPCC) 2007: Summary for policy makers. In: Metz B, Davidson OR, Bosch PR, Dave R, Meyer LA (eds) Climate Change 2007: Mitigation. Contribution of Working Group III to the Fourth Assessment Report of the Intergovernmental Panel on Climate Change. Cambridge University Press, Cambridge.
- Kanerva, T , Regina, K , Ramo, K , Ojanpera, K , and Manninen, S , 2007. Fluxes of N2O, CH4 and CO2 in a meadow ecosystem exposed to elevated ozone and carbon dioxide for three years , Environ. Pollut. 145 (2007), pp. 818–828.
- Khan, SA , Mulvaney, RL , and Mulvaney, CS , 1997. Accelerated diffusion methods for inorganic-nitrogen analysis of soil extracts and water , Soil Sci. Soc. Am. J. 61 (1997), pp. 936–942.
- Kowalenko, CG , Ivarson, KC , and Cameron, DR , 1978. Effect of moisture content, temperature and nitrogen fertilization on carbon dioxide evolution from field soils , Soil Biol. Biochem. 10 (1978), pp. 417–423.
- Lang, M , Cai, Z , and Chang, SX , 2010. Effects of land use type and incubation temperature on greenhouse gas emissions from Chinese and Canadian soils , J Soils Sediments 11 (2010), pp. 15–24.
- Lampe, C , Dittert, K , Sattelmacher, B , Wachendorf, M , Loges, R , and Taube, F , 2006. Sources and rates of nitrous oxide emissions from grazed grassland after application of 15N-labelled mineral fertilizer and slurry , Soil Biol. Biochem. 38 (2006), pp. 2602–2613.
- Le Mer, J , and Roger, P , 2001. Production, oxidation, emission and consumption of methane by soils: a review , Eur. J. Soil Biol. 37 (2001), pp. 25–50.
- Liu, H , Zhao, P , Lu, P , Wang, YS , Lin, YB , and Rao, XQ , 2008. Greenhouse gas fluxes from soils of different land-use types in a hilly area of South China , Agric. Ecosyst. Environ. 124 (2008), pp. 125–135.
- Liu, XR , Dong, YS , Qi, YC , and Li, SG , 2010. N2O fluxes from the native and grazed semi-arid steppes and their driving factors in Inner Mongolia, China , Nutr. Cycling Agroecosyst. 86 (2010), pp. 231–240.
- Loro, PJ , Bergstrom, DW , and Beauchamp, EG , 1997. Intensity and duration of denitrification following application of manure and fertilizer to soil , J. Environ. Qual. 26 (1997), pp. 706–713.
- Lü, C , and Tian, H , 2007. Spatial and temporal patterns of nitrogen deposition in China: synthesis of observational data , J. Geophys. Res. 112 (2007), p. D22S05, doi: 10.1029/(2006)JD007990.
- Maag, M , and Vinther, FP , 1996. Nitrous oxide emission by nitrification and denitrification in different soil types and at different soil moisture content and temperatures , Appl. Soil Ecol. 4 (1996), pp. 5–14.
- Maljanen, M , Jokinen, H , Saari, A , Strommer, R , and Martikainen, PJ , 2006. Methane and nitrous oxide fluxes, and carbon dioxide production in boreal forest soil fertilized with wood ash and nitrogen , Soil Use Manage. 22 (2006), pp. 151–157.
- Moore, TR , and Dalva, M , 1993. The influence of temperature and water table position on carbon dioxide and methane emissions from laboratory columns of peatland soils , Soil Sci 44 (1993), pp. 651–664.
- Mosier, AR , 1998. Soil processes and global change , Biol. Fertil. Soils 27 (1998), pp. 221–229.
- Mosier, AR , Parton, WJ , and Phengpan, S , 1998. Long-term large N and immediate small N addition effects on trace gas fluxes in the Colorado shortgrass steppe , Biol. Fertil. Soils 28 (1998), pp. 44–50.
- Mosier, AR , Parton, WJ , Valentine, DW , Ojima, DS , Schimel, DS , and Delgado, JA , 1996. CH4 and N2O fluxes in the Colorado shortgrass steppe: 1. Impact of landscape and nitrogen addition , Global Biogeochem. Cycles 10 (1996), pp. 387–399.
- Mosier, AR , Parton, WJ , Valentine, DW , Ojima, DS , Schimel, DS , and Heinemeyeer, O , 1997. CH4 and N2O fluxes in the Colorado shortgrass steppe: 2. Long-term impact of land use change , Global Biogeochem. Cycles 11 (1997), pp. 29–42.
- Mosier, AR , Schimel, D , Valentine, D , Bronson, K , and Parton, W , 1991. Methane and nitrous oxide fluxes in native, fertilized and cultivated grasslands , Nature 350 (1991), pp. 330–332.
- Rastogi, M , Singh, S , and Pathak, H , 2002. Emission of carbon dioxide from soil , Curr. Sci. 82 (2002), pp. 510–517.
- Reay, DS , and Nedwell, DB , 2004. Methane oxidation in temperate soils: effects of inorganic N , Soil Biol. Biochem. 36 (2004), pp. 2059–2065.
- Rustad, LE , and Fernandez, IJ , 1998. Experimental soil warming effects on CO2 and CH4 flux from a low elevation spruce-fir forest soil in Maine, USA , Global Change Biol. 4 (1998), pp. 597–605.
- Schaufler, G , Kitzler, B , Schindlbacher, A , Skib, U , Sutton, MA , and Zechmeister-Boltenstern, S , 2010. Greenhouse gas emissions from European soils under different land use: effects of soil moisture and temperature , Eur. J. Soil Sci. 61 (2010), pp. 683–696.
- Schils, RLM , Verhagen, A , Aarts, HFM , and Sebek, LBJ , 2005. A farm level approach to define successful mitigation strategies for GHG emissions from ruminant livestock systems , Nutr. Cycling. Agroecosyst. 71 (2005), pp. 163–175.
- Sitaula, BK , Hansen, S , Sitaula, JIB , and Bakken, LR , 2000. Methane oxidation potentials and fluxes in agricultural soil: effects of fertilisation and soil compaction , Biogeochemistry 48 (2000), pp. 323–339.
- Ullah, S , Breitenbeck, GA , and Faulkner, SP , 2005. Denitrification and N2O emission from forested and cultivated alluvial clay soil , Biogeochemistry 73 (2005), pp. 499–513.
- Yao, Z , Wolf, B , Chen, W , Butterbach-Bahl, K , Brüggemann, N , Wiesmeier, M , Dannenmann, M , Blank, B , and Zheng, X , 2010. Spatial variability of N2O, CH4 and CO2 fluxes within the Xilin River catchment of Inner Mongolia, China: a soil core study , Plant Soil 331 (2010), pp. 341–359.
- Zavaleta, ES , Thomas, BD , Chiariello, NR , Asner, GP , Shaw, MR , and Field, CB , 2003. Plants reverse warming effect on ecosystem water balance , Proc. Natl. Acad. Sci. U.S.A. 100 (2003), pp. 9892–9893.
- Zhou, X , Sherry, RA , An, Y , Wallace, LL , and Luo, Y , 2006. Main and interactive effects of warming, clipping, and doubled precipitation on soil CO2 efflux in a grassland ecosystem , Global Biogeochem. Cycles 20 (2006), p. GB1003, doi: 10.1029/(2005)GB002526.