Abstract
The decomposition of apple (Malus domestica) leaf litters has a pivotal role in nutrient release in orchard ecosystems. We studied the decomposition rate and subsequent release of nitrogen (N), phosphorus (P), and potassium (K) nutrients over 24-months using the litterbags method, from three types of litters sampled from an apple orchard which received different rates of N, P, K fertilization (control, CK; low fertilizer rate, LF; high fertilizer rate, HF). The patterns of mass loss of all three litters were quite similar following the exponential decay model. However, the amount of mass loss was greater for HF and LF litters than that for CK litter over the first six months. During the initial six months, concentrations of N and P in the leaf litters increased while that of K declined. Subsequently, concentrations of all elements decreased during 24-months of incubation. The release of N and P from leaf litter was positively correlated to the respective concentrations in the leaf litter at the beginning of decomposition. The amounts of N and P release from the litter were greater for that collected from the trees which received HF than that under CK and LF treatments during the course of decomposition in the two years. The rapid release of K occurred during the early stage across all litters and was not influenced by varying levels of leaf nutritional status. At the end of 24 months of decomposition, the relative release of nutrients from litters followed the order: K > N > P. The mean quantities of N, P, and K released during the first and second year following the application of 10, 6, 58% and 63, 6, 8% of recommended rates at respective nutrients for young apple orchards in Loess Plateau. Therefore, the results suggest that nutrient release from leaf litter in an apple orchard system can contribute a significant portion of the nutrient requirement for apple production. Accounting for this nutrient contribution in the annual fertilizer management is important to avoid excess application, optimize net returns, and maintain sustainable production systems.
Introduction
Loess Plateau is a major apple production region in China with 963,000 hm2. However, seasonal drought and low nutrient use efficiency in orchard soil are the main constraints for achieving high yield and quality of apple in this region (FAO Citation2009). In apple orchards, the annual input of vegetative portion of the tree, such as abscised leaves, pruning wood, and decaying roots, returns substantial amounts of nutrients to the soil. Recycling of nutrients from these tree residues contributes to the total nutrient requirements for the subsequent year (Mazzoleni et al. Citation2007; Parton et al. Citation2007; Tagliavini et al. Citation2007; Tonon et al. Citation2007). Estimation of amount and rate of plant available nutrients from orchard residues, and factors influencing the above transformation are important to determine the optimal nutrient requirement for sustainable apple production (Alva et al. Citation1998; Tagliavini et al. Citation2007; Tutua et al. Citation2002).
Decomposition of leaf litter and subsequent nutrient mineralization depends on climatic factor and characteristics of the litter, such as: concentrations of carbon (C) and nitrogen (N), the C/N ratio, and water soluble components (Whitmore and Handayanto Citation1997; Magill and Aber Citation1998; Teklay and Malmer Citation2004; Tagliavini et al. Citation2007; Berg and McClaugherty Citation2008; Cornwell et al., Citation2008). The litter decomposition process also depends on regional and seasonal differences in tree growth as well as fertilization regimes of the orchard (Cornwell et al. Citation2008). Knorr et al. (Citation2005) reported that litter decay rate was significantly influenced by fertilization rate, site-specific ambient N-deposition level, and litter quality, fertilizer type, litterbag mesh size, and climatic factors had no significant influence. Most of the research on litter decomposition and nutrient release, in the past, were conducted in forest ecosystems (Magill and Aber Citation1998; Teklay and Malmer Citation2004) or with residues of several annual crops (Chaves et al. Citation2004; Berg and McClaugherty Citation2008). Tagliavini et al. (Citation2007) and Ventura et al. (Citation2010) studied the release of nutrients during the decomposition of apple and peach (Prunus persica L.) abscised leaves, respectively in northern Italy. However, investigations are severely lacking on the nutrients released from leaf litters of apple trees under different N, phosphorus (P) and potassium (K) fertilization regimes in the Loess Plateau area (Dou et al. Citation1997; Alva et al. Citation1998; Tutua et al. Citation2002; Tagliavini et al. Citation2007; Tonon et al. Citation2007; Berg and McClaugherty Citation2008; Ventura et al. Citation2010).
The present study was part of a long-term project to investigate the effects of different fertilization rates on the growth and production of apple trees (Liu et al. Citation2008a). Apple trees receiving adequate rates of fertilizers produced greater amount of leaf litter, with greater N and P concentrations than those which were not fertilized (Liu et al. Citation2008b). In this study, the decomposition rates of apple leaf litters from trees subjected to different fertillizer rates were compared. Also the amount of N, P, and K released from the apple leaf litters over 24 months was estimated. This study helps to support the theoretical basis for rational fertilization and sustainable development of apple production in the Loess Plateau area of China.
Materials and Methods
Site description
This experiment was carried out in an apple (Malus domestica, Borkh.) orchard on a clay loam soil, at the experimental farm of Northwest A & F University, in Fufeng area in Loess Plateau, northern China (34°20′N, 108°24′E) at an elevation of 900 m. The mean annual temperature was 12.2°C, while maximum and minimum mean monthly temperature for this experiment site were 24.1°C and 2.1°C during July/August and January/February, respectively. The region has a temperate climate with cumulative rainfall of 700 and 552 mm in 2006 and 2007, respectively. Trees were planted in the winter 1996/1997 at 2 m × 3 m spacing. Soil in orchard was Inceptisols (Lou soil in Chinese soil taxonomy, a typical manural loessial soil) (Gong and Chen Citation1999). Soil was sampled from unfertilized plots. The characteristics of the soil in 0–40 cm depth were found using the procedures described by Miller and Keeney (Citation1982): pH 8.13; organic matter = 14.3 g kg−1 (Potassium Dichromate Volume Method); available N = 88.8 mg kg−1 [2 mol L−1 potassium chloride (KCl) Extraction, Automated Chemistry Analyzer]; available P = 49.9 mg kg−1 [0.5 mol L−1 sodium bicarbonate (NaHCO3), molybdenum Blue method]; available K = 183.1 mg kg−1 [l mol L−1 ammonium acetate (NH4OAc) Extraction, Flame Spectrometry]. During 2002–2004, trees were subjected to the following fertilization regimes: (1) control (CK), no fertilizer application; (2) low fertilizer rate (LF): 30 kg ha−1 year−1 N, 15 kg ha−1 year−1 P, 30 kg ha−1 year−1 K; (3) high fertilizer rate (HF): 70 kg ha−1 year−1 N, 35 kg ha−1 year−1 P, 70 kg ha−1 year−1 K. A mixed fertilizer containing N, P, K was broadcasted on 15 October between the rows. No fertilizers were supplied to the trees during the decomposition study, which began in 2005. The plant parameters in the experimental orchard were: plant height, 280–300 cm; crown diameter, 280–290 cm; fruit yield, 122–203 kg plot−1; leaf litterfall amount, 14.2–26.6 kg plot−1 (nine trees per plot, size of 54 m2). Plant density was 1665 plant ha−1. Mean oven-dry weights of litters from the trees receiving CK, LF and HF fertilization regimes were 1.582, 2.123 and 2.956 kg plant−1, respectively. These data were calculated from nine randomly chosen trees (three replicates) per treatment in fall 2005. The trees chosen for litter collection had the ground area around the trunk under the canopy covered with a nylon net for ease of complete collection of the litter per tree basis.
Preparation and deposition of litter bags
The decomposition of leaf litter was determined using the nylon mesh bag technique (Tagliavini et al. Citation2007). In fall 2005, fresh leaf litter was collected on nylon screens surrounding three randomly chosen trees from three fertilizer rate treatments, i.e. CK, LF and HF. No rain occurred during the period of leaf litter collection. The fresh and oven dry weights of the leaf litter from each fertilizer treatment were recorded. One millimeter mesh nylon window screen was used to prepare 30 cm × 30 cm bags according to Magill and Aber (Citation1998). A weighed quantity of air-dried leaf litter (equivalent to 25.16 g of oven-dried litter) was placed in each nylon bag. This amount represented the leaf litter present in the row space of adjacent trees after leaf abscission was completed. Within the experimental area nine randomized sub-plots (represented by a single tree) were chosen for placement of litterbags with three fertilizer rate treatments described earlier (CK, LF, HF). The decomposition of the litter collected from each fertilizer rate trees was studied under three uniform trees as a replicate. At each sub-plot, 12 litterbags were placed close to each other around the trunk. One-hundred and eight bags with litter, as per treatment, in total were prepared and placed at the beginning of the experiment (10 December 2005) on the soil surface by pinning them to the ground, below the selected trees. One litter bag each from sub-plot was sampled for analysis (total of nine litter bags at each sampling) following 1, 3, 4, 5, 6, 8, 10, 12, 15, 18, 21 and 24 months of incubation.
Determination of mineral nutrients
The leaf litters from each sub-plot were air dried and kept at room temperature prior to analysis. Litter water content was determined following oven drying until constant dry weight. The litter sample was removed from the sample bag, brushed gently to remove soil, dried at 85°C for 48 h, weighed and ground to particle size ≤ 0.2 mm mesh, and stored for chemical analysis.
Total N was determined by the micro Kjeldahl method (Miller and Keeney Citation1982). Concentration of P was determined by the ascorbic acid method and that of K by atomic absorption spectrometry (Watanabe and Olsen Citation1965).
Estimation of nitrogen, phosphorus, and potassium released from litters after 24 months of decomposition
The following equations were used:(1) where RRN is the return rate of nutrient (in g kg−1); IAN is the initial amount of nutrient in the litter at the time of placement in the soil (in g bag−1); RAN is the remaining amount of nutrient in the litter at the end of 24-month incubation (in g bag−1); IDW is the initial dry weight of litter per bag (in g bag−1).
(2) where REAN is the return amount of nutrient at the end of 12- and 24-month incubation (in kg ha−1); PD is the plant density (in plant ha−1); MDW is the mean dry weight of litter per plant (in kg plant−1).
(3) where PRF is the nutrient released from leaf litter as a percent of nutrient applied as fertilizer (%); FAA is the rate of nutrient actual applied as fertilizer (in kg ha−1).
(4) where MPRF is the mean percentage of quantities of nutrients released to recommended fertilization; RFR is the recommended N, P, K fertilization (N, 40; P, 30; K, 45 kg ha−1 year−1).
In the present experiment, initial oven-dried weight of all litter type per bag was 25.16 g.
Statistical analyses of the data
Mass and nutrients remaining were calculated by the residual litter at each sampling. The decay rate was described by a negative exponential decay model Xi/X 0 = e− kTi , where Xi is the percentage of initial mass (X 0 = 100%) of each bag remaining at each time interval i and Ti is the time in months. Values of K (decay constant, month−1) were computed for the whole period of decomposition as well as for single years or phases (Olson Citation1963). At each sampling time, the mean value of residual leaf litter was compared by analysis of variance (ANOVA) using SAS (SAS Institute Inc. Citation1996). The significance of the treatment effect was determined using F-test. Significant difference between the means was evaluated using Duncan's multiple range test at 5% probability level.
RESULTS
Mass loss during the litter decomposition
The exponential decay model showed a good fit for litter mass loss. The mass loss during litter decomposition was greater for HF and LF litters than that for CK litter during the first six months period, as evident from greater decay constants of 0.043 for HF and 0.039 for LF as compared to 0.032 for CK. However, there was no significant difference in decomposition rates among the three litters during 12 and 24 months (; and 2). The residual litter masses at the end of 24 month incubation were 33.2, 32.9, and 32.2% of the initial weights for CK, LF, and HF samples, respectively.
Figure 1. Changes in mass during 24-month incubation for three different litters sampled from trees: control, unfertilized (CK), which received low rate fertilizer (LF), and high rate fertilizer (HF). Values are means (n = 3) and bars are standard errors.
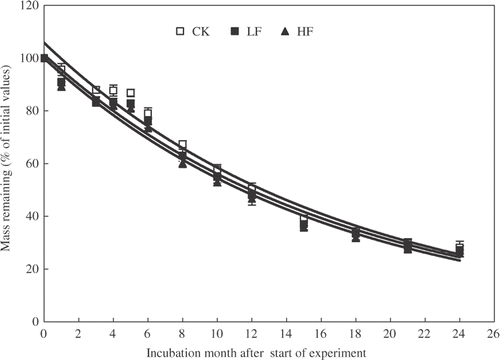
Table 1. Initial concentrations of nitrogen, phosphorus and potassium (in g kg–1) of the apple leaf litters
Dynamics of nitrogen, phosphorus and potassium nutrient concentrations in the decomposing litters
The HF leaf litter contained greater concentrations of N, P and K than those in CK and LF leaf litters (). Concentrations of N in the CK, LF and HF litters increased from 11.6 to 24.7, 12.5 to 25.4, and 14.6 to 28.1 g kg−1 respectively during the 0 to 12 months of incubation, then followed by a slight decrease towards the end of the incubation period. Concentration of N in HF litter was significantly greater than that in CK and LF leaf litters only across the first 12 months after application ().
Figure 2. Changes in concentrations of (a) nitrogen (N), (b) phosphorus (P), and (c) potassium (K) during 24-month incubation for three different litters sampled from trees: control, unfertilized (CK), which received low rate fertilizer (LF), and high rate fertilizer (HF). Values are means (n = 3) and bars are standard errors.
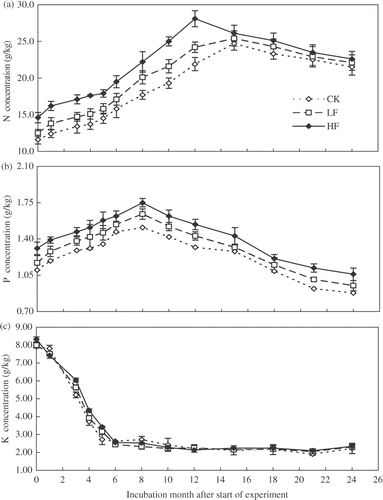
Table 2. Estimated decay rates of different types of litter mass for 6, 12 and 24 months after start of the experiment
Concentration of P was greater in HF than LF and CK litters. The concentration of P in all leaf litters increased during the initial eight months of decomposition and then decreased (). The K concentrations in all litters sharply declined during the initial five months, followed by slight to no decrease during the remaining period. The K concentrations were similar in all three litters during the entire incubation period ().
The amount of nitrogen, phosphorus and potassium nutrients remained in the leaf litters during the decomposition
The amount of N in the leaf litters decreased after 12 months of incubation. The trend of N release from leaf litters did not follow that of weight loss ( and ). The amount of N released ranged from about 4.8% in CK litter to about 10.2% in HF litter after 12 months. At the end of the 24-month incubation, almost half of the initial amount of N in all leaf litter was released (). The P release from all litters occurred after five to six months of incubation. The percentage of P released in relation to initial P mass in the leaf litters ranged from 39.5 to 45.1% across three litters during the first 12 months of decomposition, and further increased to 78–80% at the end of the 24-month incubation (). Amounts of N and P released during the first 12 months were positively correlated with the corresponding initial concentrations (r = 0.95, P < 0.01 for N; r = 0.79, P < 0.05 for P) and at the end of the 24 months (r = 0.97, P < 0.01 for N; r = 0.82, P < 0.05 for P) ().
Figure 3. Changes in amounts of (a) nitrogen (N), (b) phosphorus (P), and (c) potassium (K) remaining during 24-month incubation for three different litters sampled from trees: control, unfertilized (CK), which received low rate fertilizer (LF), and high rate fertilizer (HF). Values are means (n = 3) and bars are standard errors.
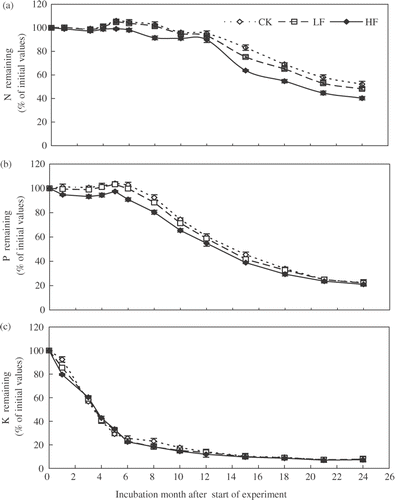
During the initial five months, K release from all litters was rapid, followed by somewhat slow release during the subsequent period. About 92–93% of initial K amounts were released after 24 months ().
The net amounts of N, P and K released from leaf litters collected from apple trees with different fertilization practices varied from 1.5 to 7.3, 1.1 to 2.9, and 17.9 to 36.1 kg ha−1 respectively at 12 months after application, and 14.6 to 43.1, 2.3 to 5.1, and 19.2 to 43.8 kg ha−1, respectively, at 24 months after application (). Thus, the recommended N, P and K rates for young apple orchards in this production region are 40, 30 and 45 kg ha−1 year−1 respectively (Liu et al. Citation2008b). Therefore, under the conditions of this study the contribution of N, P, and K from leaf litters towards the annual recommended rates of respective nutrients for young apple orchard accounts to 10, 6, and 58% respectively in the first 12 months and 63, 6 and 8% in the next 12 months following application. It is necessary to recognize that these estimates are only for leaf litters in the Loess Plateau of China.
Table 3. Estimate of nitrogen (N), phosphorus (P), potassium (K) release of different litters of apple trees under three different fertilizer regimes after 12 and 24 months of decomposition
Discussion
The mass loss of plant litters during decomposition have been described using the exponential decay model (Berg and McClaugherty Citation2008; Ventura et al. Citation2010). The decay constant (K) was calculated for comparison of litter decay rate in many studies (Ribeiroa et al. Citation2002; Tagliavini et al. Citation2007). Litter mass loss was positively correlated with initial N concentration in litter during the first year of decomposition (Cotrufo et al. Citation1995; Parton et al. Citation2007; Treseder Citation2008). Berg and McClaugherty (Citation2008) stated that the decomposition rate is greater for plant litter with greater N and P concentrations than that with lower concentrations. Nitrogen addition stimulated N release from decomposing litter although the effect was different among plant species (Whitmore and Handayanto Citation1997; Vivanco and Austin Citation2011). In the present study, however, the decomposition patterns were similar over a 24-month period regardless of litter nutrient level. Prescott (Citation1995) reported no increase in decay rates with increases in N concentration of needle and leaf litter by receiving fertilizers for several tree species. In the current study, the litter mass loss was slightly greater for HF and LF litters than that of CK litter only during the first six months (; ). In Italy, Tagliavini et al. (Citation2007) found that about 50% of original mass was lost within one year followed by about 30% mass loss in the second year in an apple orchard. Our results follow a somewhat similar trend with about 42–47% mass loss in the first year followed by 32 to 34% mass loss in the second year (). Besides litter quality, the regional N deposition and seasonal climate also affect the litter decomposition process. Knorr et al. (Citation2005) reported that litter decomposition was inhibited by N additions when ambient N deposition was high (5–10 kg N ha−1 year−1).
During the initial stage of litter decomposition, soil N is immobilized due to increased microbial activity. The litter quality, i.e. C/N ratio determines the extent of immobilization. In a peach orchard, N release during litter decomposition occurred only 44 weeks later (Ventura et al. Citation2010). As for apple litter in Italy, net N immobilization occurred during the winter–spring period and net release of these nutrients started in the second year (Tagliavini et al. Citation2007). In our study, N release patterns differ among fertilization levels: N was immobilized in the first year for CK and LF litters while N release started from the first year for HF litter (). According to the N release pattern, our results suggested that N in CK and LF litters was deficit for releasing N immediately. At the end of the 24 months incubation, all litters finally contained almost a similar range of N concentration (21.5–25.2 g kg−1) (). The effects of N release from the litter may affect soil N condition only in the first year.
During the first six months of incubation, no P release occurred from all three litters, followed by fairly rapid release during the rest of the incubation (). Polglase et al. (Citation1992) reported that the initial P release occurred by both direct leaching and through microbial decomposition, which increased with the inorganic P content of the litters by P fertilization.
Across all three litters nearly 90% of the K was released within the first year of decomposition (). Similar trend was reported by Ribeiroa et al. (Citation2002), who concluded that high release of litter K occurred from the early stage of litter decomposition, which was attributed to the fact that K was not bound to organic matter. Tagliavini et al. (Citation2007) also reported that the low K remaining in the early incubation period was consistent with the high mobility of K due to lack of incorporation of this element into organic structures. Potassium release was faster and greater than that of either N or P which occurred immediately after leaf abscission during winter and the remaining amount was constant up to the end of the experiment (Tagliavini et al. Citation2007; Ventura et al. Citation2010).
The release of N, P and K from litter decomposition is an important component of fruit tree nutrient budgets. Nitrogen mineralization from citrus tree residues under the canopy accounted for 58 to 84 kg ha−1 for the four-year-old trees and 121 to 153 kg ha−1 year−1 on the Tavares fine sandy soil, and 39 to 64 kg ha−1 for the seven-year-old trees and 121 to 126 kg ha−1 year−1 for 40-year-old trees on the Wabasso sandy soil (Dou et al. Citation1997). Tagliavini et al. (Citation2007) estimated that abscised leaves mass provided about 25 to 30 kg K ha−1 for root uptake in the spring. Net nutrients release from the leaf litters were estimated to be almost 10% for N, 8 to 11% for P, and 52 to 82% for K in the leaf litters during the first 12 months and 31 to 38% for N, 7 to 11% for P, and 31 to 43% for K after 24 months of application of leaf litter. In addition, we should consider other vegetative litters, including small twigs and roots, for nutrient sources, which would also release available nutrients through decomposition (Dou et al. Citation1997; Tagliavini et al. Citation2007).
These results suggest that nutrient release from leaf litters improves soil fertility especially for N and K in apple orchard systems. The knowledge of the dynamics of nutrient release from the decomposing litter will be a useful tool for predicting nutrient availability in apple orchards. Accurate estimation of nutrients availability from leaf litters in an apple orchard need to be accounted to adjust the nutrient requirement for any given fruit yield targets.
Acknowledgments
The research work was supported by financial support from the Agriculture Ministry of P. R. China, through National Apple Industry Technology System (CARS-28), the 948 Apple Project (2011-Z24), Program for Agricultural Sci-Tech Innovation of Shaanxi Province (2011NXC01-18) and West Light Foundation of CAS (2060299-14). The Fufeng experimental farm of Northwest A & F University contributed the apple orchard site of the experiment and technical assistance. The laboratory work was conducted at the Apple Center in Northwest A & F University. Han Ming-yu and Zhang Li-xin contributed to this work equally.
References
- Alva, AK , Paramasivam, S , and Graham, WD , 1998. Impact of nitrogen management practices on nutritional status and yield of Valencia orange trees and groundwater nitrate , J. Environ. Qual. 27 (1998), pp. 904–910.
- Berg, B , and McClaugherty, C , 2008. "Plant Litter". In: Decomposition, Humus Formation, Carbon Sequestration, . Berlin: Springer-verlag; 2008.
- Chaves, B , De Neve, S , Hofman, G , Boeckx, P , and Van, CO , 2004. Nitrogen mineralization of vegetable root residues and green manures as related to their (bio) chemical composition , Eur. J. Agron. 21 (2004), pp. 161–170.
- Cornwell, WK , Cornelissen, JHC , and Amatangelo, K , 2008. Plant species traits are the predominant control on litter decomposition rates within biomes worldwide , Ecol. Lett. 11 (2008), pp. 1065–1071.
- Cotrufo, MF , Ineson, P , and Roberts, JD , 1995. Decomposition of birch leaf litters with varying C-to-N ratios (short communication) , Soil Biol. Biochem. 27 (1995), pp. 1219–1221.
- Dou, H , Alva, AK , and Khakural, BR , 1997. Nitrogen mineralization from citrus tree residues under different production condition , Soil Sci. Soc. Am. J. 61 (1997), pp. 1226–1232.
- Food and Agriculture Organization (FAO) 2009: stat (OL). http://faostat.fao.org/.
- Gong, ZT , and Chen, ZC , 1999. Chinese Soil Taxonomy . Beijing: Science Press; 1999.
- Knorr, M , Frey, SD , and Curtis, PS , 2005. Nitrogen addition and litter decomposition: a meta-analysis , Ecology 86 (2005), pp. 3252–3257.
- Li, SX , 2007. Dryland Farming in China . Beijing: Science Press; 2007.
- Liu, CH , Han, MY , and Zhang, LX , 2008a. The effects of fertilizer application at early summer on growth, yield and quality of Fuji apple in Weibei Highland , Agri. Res. Arid Areas 16 (2008a), pp. 62–67, (in Chinese with English summary).
- Liu, RL , Tong, YA , Gao, YM , and Zhao, Y , 2008b. Study on soil nutrients in apple orchard and balanced fertilization in Shaanxi Weibei dryland , J. Northwest A & F Uni. (Nat. Sci. Ed.) 3 (2008b), pp. 135–140, (in Chinese with English summary).
- Magill, AH , and Aber, JD , 1998. Long-term effects of experimental nitrogen additions on foliar litter decay and humus formation in forest ecosystems , Plant Soil 203 (1998), pp. 301–311.
- Mazzoleni, S , Bonanomi, G , Giannino, F , Rietkerk, M , Dekker, SC , and Zucconi, F , 2007. Is plant biodiversity driven by decomposition processes? An emerging new theory on plant diversity , Community Ecol. 8 (2007), pp. 103–113.
- Miller, RH , and Keeney, DR , 1982. Methods of Soil Analysis, Part 2, . Madison, WI: Agronomy Society of America and Soil Science Society of America; 1982.
- Olson, JS , 1963. Energy storage and the balance of producers and decomposers in ecological systems , Ecology 44 (1963), pp. 322–331.
- Parton, W , Silver, WL , Burke, IC , Grassens, L , Harmon, ME , Currie, WS , King, JY , Adair, EC , Brandt, LA , Hart, SC , and Fasth, B , 2007. Global-scale similarities in nitrogen release patterns during long-term decomposition , Science 315 (2007), pp. 361–364.
- Polglase, PJ , Jokela, EJ , and Comerford, NB , 1992. Nitrogen and phosphorous release from decomposing needles of southern pine plantations , Soil Sci. Soc. Am. J. 563 (1992), pp. 914–920.
- Prescott, CE , 1995. Does nitrogen availability control rates of litter decomposition in forest , Plant Soil 168–169 (1995), pp. 83–89.
- Ribeiroa, CM , Madeira, M , and Araujo, MC , 2002. Decomposition and nutrient release from leaf litter of Eucalyptus globulus grown under different water and nutrient regimes , Forest Ecol. Manage. 171 (2002), pp. 31–41.
- SAS Institute Inc, 1996. Getting started with PROC ANOVA . Cary, NC: SAS Institute Inc; 1996.
- Tagliavini, M , Tonon, G , Scandellari, F , Quinones, A , Palmieri, S , Menarbina, G , Gioacchini, P , and Masia, A , 2007. Nutrient recycling during the decomposition of apple leaves (Malus domestica) and mowed grasses in an orchard , Agr. Ecosyst Environ. 118 (2007), pp. 191–200.
- Teklay, T , and Malmer, A , 2004. Decomposition of leaves from two indigenous trees of contrasting qualities under shaded-coffee and agricultural land-uses during the dry season at Wondo Genet, Ethiopia , Soil Biol. Biochem. 36 (2004), pp. 777–786.
- Tonon, G , Ciavatta, C , Solimando, D , Gioacchini, P , and Tagliavini, M , 2007. Fate of N-15 derived from soil decomposition of abscised leaves and pruning wood from apple (Malus domestica) trees , Soil Sci. Plant Nutr. 53 (2007), pp. 78–85.
- Treseder, KK , 2008. Nitrogen additions and microbial biomass: a meta-analysis of ecosystem studies Ecol , Lett 11 (2008), pp. 1111–1120.
- Tutua, SS , Goh, KM , and Daly, MJ , 2002. Decomposition and nitrogen release of understorey plant residues in biological and integrated apple orchards under field conditions in New Zealand , Bio. Fertil. Soils 35 (2002), pp. 277–287.
- Ventura, M , Scandellari1, F , Bonora, E , and Tagliavini, M , 2010. Nutrient release during decomposition of leaf litter in a peach (Prunus persica L.) orchard , Nutr. Cycling Agroecosyst. 87 (2010), pp. 115–125.
- Vivanco, L , and Austin, AT , 2011. Nitrogen addition stimulates forest litter decomposition and disrupts species interactions in Patagonia, Argentina , Global Change Bio. 17 (2011), pp. 1963–1974.
- Watanabe, FS , and Olsen, SR , 1965. Test of an ascorbic acid method for determining phosphorous in water and NaHCO3 extracts from soil , Soil Sci. Soc. Am. Proc. 291 (1965), pp. 677–678.
- Whitmore, AP , and Handayanto, E , 1997. "Simulation the mineralization of N crop residues in relation to residue quality". In: Cadisch, G , and Giller, KE , eds. Driven by Nature. Plant Litter Quality and Decomposition . Wallingford: CAB International; 1997. pp. 337–340.