Abstract
High boron (B) tolerance is an important trait for crop production in high-B soils. We previously reported that overexpression of BOR4, an Arabidopsis thaliana B exporter, conferred high B tolerance in A. thaliana. This improvement appeared to be mainly due to the decreased B concentrations in roots through BOR4-mediated exclusion of B. In the present study, we describe a novel role of overexpressed BOR4 in shoots for high B tolerance. We compared shoot growth of wild type A. thaliana and transgenic plants moderately overexpressing BOR4 in relation to B concentration in shoots. It was found that growths and chlorophyll accumulation of shoots containing similar levels of B are better in the transgenic plants than the wild type. This finding established that BOR4-overexpressing plants are more capable of expanding leaves and accumulating chlorophyll in the presence of high B in shoot tissues. BOR4-GFP was found to be localized to plasma membrane in leaf epidermis cells. We suggest that overexpressed BOR4 alters B distribution in leaves by exporting B from the cytoplasm to the apoplast, leading to enhancement of high B tolerance in shoots.
Introduction
Boron (B) is an essential micronutrient but supply of excess B hampers plant growth, causing necrosis in leaf margins and inhibition of root elongation (Nable et al. Citation1997). High B accumulation occurs both naturally and artificially. Evaporation of soil solutions and usage of groundwater containing high levels of B for irrigation elevates B contents in soils. Reduction of agricultural production is reported in such high-B soils. High-B soils are distributed over semi-arid areas of the world including southern Australia, west Asia and North Africa, North America (California) and South America (Chile) (Gupta et al. Citation1995; Nable et al. Citation1997). According to an early study by Cartwright et al. (Citation1984), up to 17 % of yield loss of barley was estimated to result from B toxicity in southern Australia. Therefore, breeding programs have been conducted, including physiological comparison among cultivars and QTL mapping, particularly for barley (Hordeum vulgare) (Jefferies et al., Citation1999; Karakousis et al. Citation2003), wheat (Triticum aestivum) (Jefferies et al. Citation2000) and rice (Oryza sativa) (Ochiai et al. Citation2008), leading to the identification of four, three and one genetic loci controlling B tolerance, respectively.
In general, plants have protection systems against toxic substances such as high salts and heavy metals. Exclusion, detoxification (e.g., formation of conjugate), and compartmentation (e.g., isolation into vacuoles) are among the well-known mechanisms. For high-B resistance, tolerant cultivars tend to show lower B concentration in shoots compared to susceptible cultivars in pea (Pisum sativum) (Bagheri et al. Citation1992) and Brassica napus (Kaur et al. Citation2006), possibly due to reduced uptake and/or different allocation in the plant body. Hayes and Reid (Citation2004) proposed that borate efflux from roots was a B-tolerant mechanism in barley, and Nable (Citation1988) and Reid (Citation2007) clearly demonstrated the positive links between B concentrations of roots and shoots in barley and wheat. These reports suggested the presence of efflux-type borate transporter(s) in roots, responsible for B tolerance in plants.
The first B transporter, BOR1, was identified from Arabidopsis thaliana (Takano et al. Citation2002). BOR1 is an efflux-type B transporter for efficient translocation of B from roots to shoots under B limitation, and is capable of reducing B concentration when expressed in yeasts. Overexpression of AtBOR1 or its yeast homolog Bor1p in yeast conferred high B tolerance by decreasing B concentration in cells (Nozawa et al. Citation2006; Takano et al. Citation2007). Overexpression of AtBOR1 in plants, however, did not confer high B tolerance; instead, it conferred low B tolerance (Miwa et al., Citation2006). This was attributed to the degradation of BOR1 under high B supply, and enhancement of the root-to-shoot translocation of B under low B conditions (Takano et al. Citation2005; Miwa et al. Citation2006). To confer high B tolerance to plants, a B-efflux transporter that is stable in the presence of high B should be used. Among the six BOR1-like genes in the A. thaliana genome (Nakagawa et al. Citation2007), BOR4 was found to be stably expressed under high B (Miwa et al. Citation2007). Indeed, BOR4-overexpressing A. thaliana plants are highly tolerant to high-B conditions (Miwa et al. Citation2007). B concentrations of roots and shoots in the highly BOR4-expressing plant line (Line 4) were decreased threefold under toxic levels of B supply compared to the wild type plants. Reduction of root and shoot B concentrations is considered to be a major reason for improved growth of roots and shoots. Expression of BOR4 decreased steady state levels of B concentrations in roots (Miwa et al. Citation2007) and this likely leads to the reduction of B concentrations in shoots. Therefore, it is suggested that efflux of B in roots is a primary process for high B tolerance in the transgenic plants.
Similarly, Sutton et al. (Citation2007) performed QTL mapping between the two barley cultivars for B tolerance and found the mapped region contained BOR1 homolog Bot1. The levels of Bot1 mRNA were significantly higher in the tolerant cultivar than in the susceptible cultivar. Reid (Citation2007) also cloned the BOR1 homologs HvBor2 and TaBor2 from barley and wheat, respectively. High levels of mRNA were detected in roots of barley and wheat, and positive correlation was described between the mRNA levels, reduction of B concentrations in roots and plant growth among the cultivars. These results on BOR1 homologs further support their roles for reduction of root B concentrations and high B tolerance.
In barley and wheat, mRNA levels of Bor2 were lower in shoots than in roots. Reduction in root B concentrations was considered to account for the differences in high B tolerance; therefore less attention was paid to the possible roles of BORs expressed in shoots. In the present study, it is revealed that overexpressed BOR4 in the shoots contributed to the detoxification of high concentrations of B, possibly due to enhanced excretion of B into the apoplast.
Materials and Methods
Plant materials and culture
Arabidopsis thaliana (L.) Heynh. (Col-0) is from our laboratory stock. The transgenic A. thaliana lines carrying Pro35S:BOR4-GFP were generated as described in Miwa et al. (Citation2007). In the present study, transgenic lines expressing BOR4 at a moderate levels (Lines 1 and 5) were selected and T3 seeds of the transgenic lines homozygous for T-DNA insertion were used. In Lines 1 and 5, GFP expression and growth improvement were moderate among the seven transgenic lines tested (Miwa et al. Citation2007). Since B concentrations in shoots varied greatly in response to supplies of 1 to 10 mM boric acid in Lines 1 and 5 compared to the other lines, these lines were selected to characterize the relationships between the B concentrations and growth properties in shoot tissues. To observe vegetative growth, surface-sterilized seeds were sown on the MRGL solid media (Fujiwara et al. Citation1992) containing 2% sucrose, 1.5% gellan gum and various concentrations of boric acid. The plates were vertically placed and incubated at 22°C under a 16-h light / 8-h dark condition.
GFP imaging
Rosette leaves of 14-d old plants grown under 30 µM boric acid supply were subjected to fluorescence microscopy (Olympus BZ53) to observe GFP fluorescence. It was confirmed that fluorescence was not observed in the wild type plants under the same conditions.
Growth measurement
Plants were grown in solid media containing 0.03, 1, 2, 3, 4, 5, 6, 7, 8, 9 and 10 mM boric acid for 18 d. Aerial portions of the plants were harvested and subjected to chlorophyll measurement and determination of B concentrations (n = 4–16 for shoot fresh weight; n = 6–8 for chlorophyll accumulation; n = 6–8 for B concentrations at 0.03, 3 and 6 mM; n = 2 for B concentrations at 1, 2, 4, 5, 7, 8, 9, and 10 mM). For dry weight of roots, 3 plants at 0.03 mM and 4 plants at 3 mM were combined and dry weight was determined (n = 4–6).
Chlorophyll measurement
Chlorophyll was measured as described by Ritchie (Citation2006). Aerial portions of the plants were homogenized in ice-cold ethanol saturated with calcium carbonate and centrifuged, and the supernatant was collected. Absorption at 649 nm (A649) and 665 nm (A665) was determined, and chlorophyll contents (a+b) were converted from the following equations:
Determination of B concentration in shoots
Plant seedlings were dried at 60°C for 48 h, and then dry weights were measured. Plant samples were placed in 8 mL Teflon tubes and 1 mL of concentrated nitric acid was added. The samples were digested at 130°C for 2 h and the pellets were dissolved with 3 mL of 0.08N nitric acid containing 5 ppb Be as an internal standard. B concentrations were determined by ICP-MS (SPQ9000, SII, Tokyo, Japan).
Isolation of protoplasts from A. thaliana leaves and B treatment
Plants were grown in solid media containing 0.03 mM boric acid for 18 d. Rosette leaves were cut into 0.1- to 1-mm strips and incubated in a mannitol solution (400 mm mannitol, 10 mM CaCl2, 5mM MES-KOH (pH 5.7)) containing 1% cellulase onozuka RS (Yakult, Tokyo) and 0.1% pectolyase Y-23 (Kyowa chemical products, Osaka) with gentle shaking at 40 rpm for 2 h at 25°C. Then the protoplasts were filtered with 200 µm nylon mesh, and centrifuged at 180 g for 3 min. After washing with the mannitol solution, they were washed and re-suspended with the betaine solution (500 mM betaine, 10 mM CaCl2, 5mM MES-KOH (pH 5.7)), and cell number was counted. For B treatment, the method described in Reid and Fitzpatrick (Citation2009) was used. The samples were incubated with the betaine solution containing 10 mM boric acid for 20 min, and centrifuged to collect the protoplasts. They were washed with the betaine solution twice and boiled with water at 95°C for 30 min. They were centrifuged to collect the supernatant.
Determination of B concentration in protoplasts
B concentrations in the protoplasts were determined by the Azomethine-H method. For Azomethine-H solution, 1.0 g Azomethine-H and 3.0 g L(+)-ascorbic acid were dissolved in water to total 100 mL. For the buffer, 50 g ammonium acetate, 3 mL sulfuric acid, 1 mL phosphoric acid, 0.2 g citrate monohydrate, and 2.0 g EDTA-Na2 were dissolved in water to total 120 mL. After mixing the Azomethine-H solution and the buffer (1 : 1), the mixed Azomethine-H solution was added to the sample solution (1 : 4). They were incubated at room temperature for 60 min and the absorbance at 405 nm was measured. The measurement was linear in a concentration range of 0 to 0.25 mM boric acid.
Results
BOR4-GFP was localized to plasma membrane of rosette leaves
Expression of BOR1-GFP was detected in both roots and shoots of the transgenic plants carrying Pro35S:BOR4-GFP (Miwa et al. Citation2007). BOR4 was localized to the plasma membrane of the distal side of the epidermal cells in roots of transgenic plants carrying ProBOR4:BOR4-GFP (Miwa et al. Citation2007) and Pro35S:BOR4-GFP (Langowski et al. Citation2010). To elucidate subcellular localization of BOR4-GFP in rosette leaves, GFP fluorescence was observed. Intense GFP fluorescence was detected at the periphery of all epidermal cell types in the adaxial side of leaves (), suggesting that BOR4-GFP expressed in leaves is localized to the plasma membrane of the cells.
Growth improvement of the shoots of the transgenic lines overexpressing BOR4 under a range of toxic levels of B
We first examined growth properties of transgenic plants in a wide range of B concentration in the media. When the wild type plants and the transgenic plants were grown in the solid media containing various concentrations of boric acid, fresh weights of the aerial portion of the plants were decreased with increasing B concentrations in the media ().
Figure 2. Growth of transgenic A. thaliana overexpressing BOR4 under a range of boron (B) concentrations. Fresh weights of aerial portion of the plants were determined. Wild type (Col-0) and transgenic lines 1 and 5 were grown with solid media containing high (1, 2, 3, 4, 5, 6, 7, 8, 9 and 10 mM boric acid) for 18 days and growth was compared with that using the control media (0.03 mM boric acid). Wild type plants did not grow on media containing 7 mM or more boron and growth was not determined. Means ± SE are shown (n = 4–16). Compared to the control condition, significant differences were found at 1 mM and more in Col-0, at 4 mM and over in Line1, and at 5 mM and more in Line 5 (p < 0.05, Student's t-test for comparison with the control condition of the same plant line). Significant differences were detected at all the conditions in Line 1, and at 1 mM and more in Line 5, compared with Col-0 plants (p < 0.05, Student's t-test for comparison with Col-0 plants under the same condition).
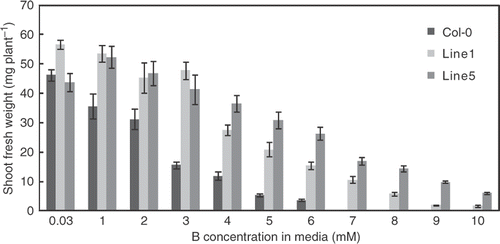
In wild type plants, a supply of 7 mM boric acid was almost lethal. Shoot fresh weights of wild type were reduced to 34% of those at the control condition (0.03 mM) under 3 mM. By contrast, the mean root dry weight of one plant was 1.03 mg at 0.03 mM and 0.65 mg (63%) at 3 mM. Since the extent of growth reduction was greater in shoots than in roots under this condition, the growth reduction of shoots is likely not due to root growth retardation, but rather to high accumulation of B in the leaves.
Growths of the transgenic plants were similar to the wild type under the control condition (0.03 mM) and better than the wild type under the conditions tested (1–10 mM). The growths of transgenic plants were reduced to about 50% of the control condition by the exposure to 5–6 mM boric acid. Significant growth was observed in the presence of 7 mM or higher concentration of boric acid. These observations establish that the growth of the transgenic plants is improved over a wide range of toxic B concentrations in the media.
To examine the growth of the transgenic plants from the different perspectives, chlorophyll contents were determined after exposure to a range of B concentration in the media. Chlorosis is one of the typical symptoms of B toxicity. Chlorophyll contents were also reduced by exposure to high levels of B (). The extent of the reduction of chlorophyll contents was less severe in the transgenic lines (Lines 1 and 5) than in the wild type plants, establishing that the transgenic plants are tolerant to high B in terms of chlorophyll contents.
Figure 3. Chlorophyll contents of transgenic Arabidopsis thaliana overexpressing BOR4 under a range of boron (B) concentrations. Chlorophyll contents of the aerial portion of the plants were determined after grown in solid media containing 0.03, 1, 2, 3, 4, 5, 6, 7, 8, 9 and 10 mM boric acid for 18 days. Means ± SE are shown (n = 3–8). FW = Fresh weight. Values of Line 1 at 9 and 10 mM were not determined. Compared to the control condition, significant differences were found at 4 mM and more in Col-0, at 3 mM and more in Line1, and at 6 mM and more in Line 5 (p < 0.05, Student's t-test for comparison with the control condition of the same plant line). Significant differences from Col-0 plants were detected at 4 mM in Line 1, and 3 mM and over in Line 5 (p < 0.05, Student's t-test for comparison with Col-0 plants under the same condition).
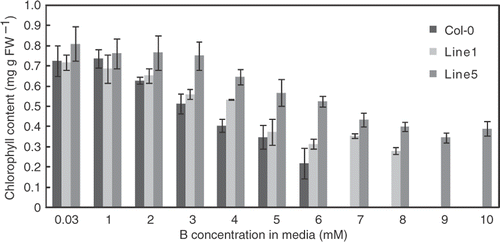
B concentration in rosette leaves of the transgenic plants
We reported that B concentration in both roots and shoots was reduced in transgenic plants that highly express BOR4 (Miwa et al. Citation2007). Here we determined B concentrations in the rosette leaves of transgenic plants moderately expressing BOR4 and the wild type plants grown in the media with 0.03, 3 and 6 mM boric acid (). At 0.03 mM B supply, significant differences were not observed between the wild type plants and the transgenic plants of Line 1 or Line 5, respectively (p > 0.05, Student's t-test). At 3 mM, B concentrations in both transgenic plants were 31% lower than the wild type. At 6 mM, B concentration in Line 1 was similar to the wild type and that in Line 5 was 33% lower than the wild type. These results established that B concentration in shoots also tends to be lower in the BOR4-moderately expressing transgenic plants than in the wild type plants. However, the reduction of B concentration was less extensive than in BOR4 highly expressing transgenic plants (65% reduction at 3 mM in Line 4, Miwa et al. Citation2007).
Figure 4. Boron (B) concentrations of transgenic plants. Boron concentrations of the aerial portion of the plants were determined after they were grown in solid media containing 0.03, 3 and 6 mM boric acid for 18 days. Means ± SE are shown (n = 6–8). DW = dry weight. The label of the longitudinal axis on the left is for values at 0.03 mM, and that in the right is for values at 3 and 6 mM. Significant differences were found at 3 mM in Line 1 and at 3 and 6 mM in Line 5, compared with Col-0 under the same conditions (p < 0.05, Student's t-test).
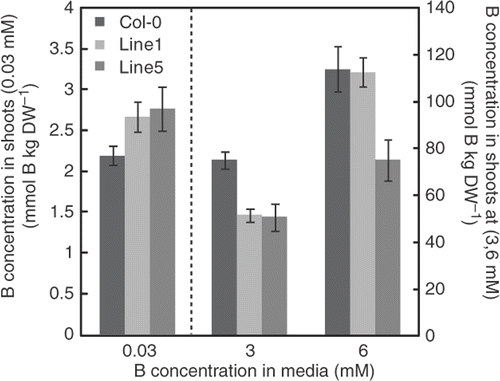
The B concentrations in shoots were similar between Line 1 and the wild type at 6 mM (). However, growth was significantly improved in the transgenic lines compared to the wild type at this condition (). The B concentration in Line 5 at 6 mM and the wild type at 3 mM were similar (), while growth was significantly better in the transgenic lines at 6 mM than in the wild type at 3 mM (). It is apparent that growth improvement in shoots cannot be explained merely by reduction of B concentration in shoots.
Relationships between physiological parameters and B concentrations in the shoot tissues
To obtain insights into the possible mechanisms of high B tolerance in leaves of BOR4-expressing plants, we examined the relationships between B concentrations in shoot tissues, and fresh weights () and total chlorophyll accumulations in shoots (), grown under a wide range of B levels. Fresh weights and total chlorophyll contents were negatively correlated with B concentrations in the shoot tissues both in the wild type plants and the transgenic lines. The transgenic Lines 1 and 5 exhibited higher fresh weights and total chlorophyll contents than the wild type plants with shoot tissues containing similar levels of B. These analyses established that transgenic plants are capable of withstanding high B concentration in shoot tissues.
Figure 5. Correlation between boron (B) concentration and growth. Means of B concentrations (X-axis) and fresh weight (Y-axis) of shoots of the plants grown under 1–10 mM boric acid for 18 days were plotted (n = 4–16 for shoot fresh weight, n = 2–8 for B concentration).
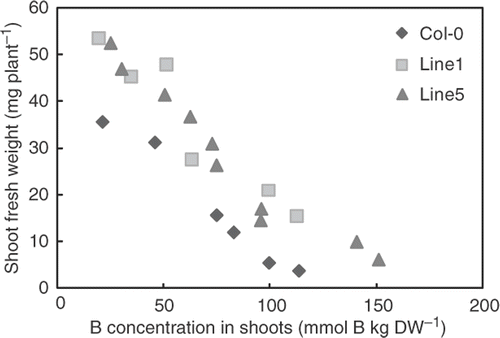
Figure 6. Correlation between boron (B) concentration and chlorophyll accumulation. Means of B concentrations (X-axis) and chlorophyll accumulation (Y-axis) of shoots of the plants grown under 1–10 mM boric acid for 18 days were plotted (n = 6–8 for chlorophyll accumulation, n = 2–8 for B concentration).
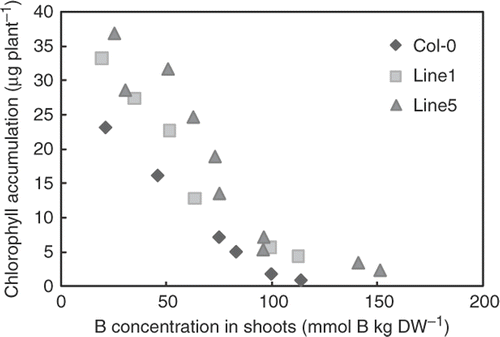
B contents in protoplasts isolated from A. thaliana rosette leaves
To examine whether overexpression of BOR4 alters B distribution between cytoplasm and apoplasm, the protoplasts from rosette leaves of wild type and the transgenic Line 5 were isolated. They were then incubated with the medium containing10 mM boric acid, and the B contents were determined. It was found that the B content in the protoplasts of the transgenic Line 5 was 83% of that of the wild type (). This result suggests that the intracellular B contents are lower in the transgenic plants than in the wild type.
Figure 7. Boron (B) contents of the protoplasts isolated from Arabidopsis thaliana leaves. The protoplasts isolated from A. thaliana rosette leaves of Col-0 and the transgenic Line 5 were exposed to 10 mM boric acid, and then the B contents were determined. Means ± SE are shown (n = 4). A significant difference was found between Col-0 and the transgenic Line 5 (p < 0.05, Student's t-test).
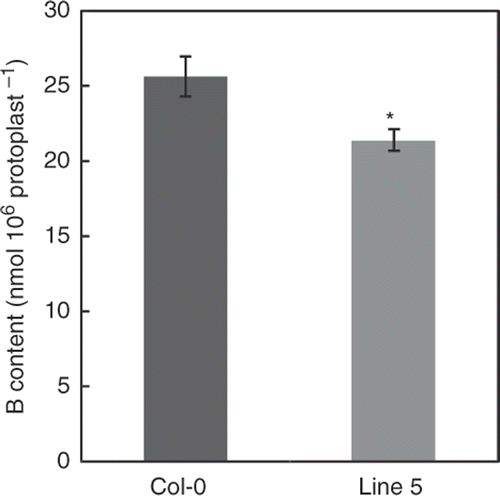
Discussion
We have previously reported that transgenic plants overexpressing BOR4 show improved growth and decreased B concentrations in roots and shoots through BOR4-mediated exclusion of B in roots under high B conditions. In the present study, we established that overexpressed BOR4 in leaves further conferred high B tolerance, possibly through alteration of B distribution.
The molecular mechanism underlying B toxicity in plant cell growth remains mostly unknown. In tobacco (Nicotiana tabacum) cultured cell under excess B supply (10 and 20 mM boric acid), more than 70% of total B was present in the water-soluble fraction, and B concentration of both the water-soluble and the insoluble fraction was decreased in the isolated high B-tolerant cell line (Ghanati et al. Citation2001). In sunflower (Helianthus annuus), excess B supply (1,000 µM) in short time increased soluble B concentration but not the insoluble B concentration presumably present in cell walls, and B concentration of cell sap is higher than that of apoplastic fluid under normal to toxic levels of B supply (1–1,000 µM) (Dannel et al. Citation1999). These studies established that under high B conditions, B is mostly present in the symplast. It is reasonable to assume that high intracellular B concentration negatively affects cellular processes under toxic levels of B supply. This view coincides with the observation that expression of AtBOR1 and Bor1p in yeast cells decreased intracellular B concentrations and improved B tolerance (Nozawa et al. Citation2006; Takano et al. Citation2007).
In the present study, based on the relationship between the growth parameters and B concentrations in the shoot tissues ( and ), it was established that shoot growth was enhanced in the transgenic Lines 1 and 5 compared to wild type plants when shoot tissues contained similar levels of B. This effect was common in two independently generated transgenic plants, suggesting that this is caused by overexpression of BOR4. Based on the assumption that high intracellular B concentration negatively affects cellular activities including chlorophyll contents and cell growth, the improvement in growth and chlorophyll accumulation resulted from lower intracellular B concentration in the transgenic plants (). It is likely that expression of efflux B transporter BOR4 increased accumulation of B in apoplasm and reduced it in cytoplasm.
Different sensitivity to high B accumulation in leaves has been reported in barley and wheat. Reid and Fitzpatrick (Citation2009) found that B concentrations in necrotic parts of leaves are higher in the tolerant cultivar than those in the sensitive cultivar in both barley and wheat. This observation also indicates that high B tolerance does not necessarily correlate with the tissue B concentration and that shoot tissues have tolerance mechanisms against B toxicity. The tolerant barley cultivar was shown to have higher Bor2 mRNA levels and lower B concentrations in the protoplasts in leaves (Reid and Fitzpatrick, Citation2009). These results are consistent with our present observations that the transgenic plants expressing BOR4 tolerate higher levels of B in leaf tissues than do the wild type plants. In our case, in contrast to the case reported by Reid and Fitzpatrick (Citation2009), it is clear that the effect is caused by expression of BOR4, an efflux transporter of B. In addition, our study demonstrates that expressed BOR4 is localized to the plasma membrane (), strongly suggesting that BOR4 contributes to exporting B from cytoplasm into apoplasm for B tolerance. In our present study, although B content in the protoplasts was determined (), B concentration in the apoplasts of leaves of A. thaliana is not determined. We tried to collect apoplastic fluid from leaves of A. thaliana without success, possibly due to the small and fragile nature of the leaves. We acknowledge the importance of determining B distribution in leaves in the future.
Excluded B does not seem to form a stabilized complex in apoplasm or bind to cell walls for detoxification, if we assume that insoluble B was not increased with the increase of B supply as was the case with sunflower (Dannel et al. Citation1999). Considering the high permeability of the lipid bilayer to boric acid, constitutive efflux of boric acid into apoplasm by active transporters is likely energy-consuming but effective for reduction of cytotoxicity.
In the present study, we provide genetic evidence that expression of a B exporter is responsible not only for primary efflux of B in roots but also for high-B tolerance in leaves. Further, the B-tolerant mechanisms of distribution of B into apoplasm in shoot tissues are shown to be effective in both monocotyledonous and dicotyledonous plants. Our study also provides a novel technology for generating plants tolerant to high B conditions.
Acknowledgments
We thank Ms Mie Chiba for technical assistance and Dr Junpei Takano for critical reading. We appreciate the JSPS Research Fellowship for young scientists and the Hokkaido University leader development system in basic interdisciplinary research, granted to KM. This research was supported in part by a grant (Kiban S) from the Japanese Society for the Promotion of Science, and a Grant-in-Aid for Scientific Research on Priority Areas from the Ministry of Education, Culture, Sports, Science, and Technology of Japan, from the Ministry of Agriculture, Forestry, and Fisheries of Japan (Genomics for Agricultural Innovation Grant IPG-0005) to TF.
References
- Bagheri, A , Paull, JG , Rathjen, AJ , Ali, SM , and Moody, DB , 1992. Genetic variation in the response of pea (Pisum sativum L.) to high soil concentrations of boron , Plant Soil. 146 (1992), pp. 261–269.
- Cartwright, B , Zarcinas, BA , and Mayfield, AH , 1984. Toxic concentrations of boron in a red-brown earth at Gladstone, South Australia , Aust. J. Soil. Res. 22 (1984), pp. 261–272.
- Dannel, F , Pfeffer, H , and Römheld, V , 1999. Distribution within the plant or compartmentation does not contribute substantially to the detoxification of excess boron in sunflower (Helianthus annuus) , Aust. J. Plant Physiol. 26 (1999), pp. 95–99.
- Fujiwara, T , Hirai, MY , Chino, M , Komeda, Y , and Naito, S , 1992. Effects of sulfur nutrition on expression of the soybean seed storage protein genes in transgenic petunia , Plant Physiol. 99 (1992), pp. 263–268.
- Ghanati, F , Morita, A , and Yokota, H , 2001. Selection and partial characterization of a boron-tolerant tobacco cell line , Soil Sci. Plant Nutr. 47 (2001), pp. 405–410.
- Gupta, UC , Jame, YM , Campbell, CA , Leyshon, AJ , and Nicholaichuk, W , 1995. Boron toxicity and deficiency: a review , Can. J. Soil Sci. 65 (1995), pp. 381–409.
- Hayes, JE , and Reid, RJ , 2004. Boron tolerance in barley is mediated by efflux of boron from the roots , Plant Physiol. 136 (2004), pp. 3376–3382.
- Jefferies, SP , Barr, AR , Karakousis, A , Kretschmer, JM , Manning, S , Chalmers, KJ , Nelson, JC , Islam, AKMR , and Langridge, P , 1999. Mapping of chromosome regions conferring boron toxicity tolerance in barley (Hordeum vulgare L.) , Theor. Appl. Genet. 98 (1999), pp. 1293–1303.
- Jefferies, SP , Pallotta, MA , Paull, JG , Karakousis, A , Kretschmer, JM , Manning, S , Islam, AKMR , Langridge, P , and Chalmers, KJ , 2000. Mapping and validation of chromosome regions conferring boron toxicity tolerance in wheat (Triticum aestivum) , Theor. Appl. Genet. 101 (2000), pp. 767–777.
- Karakousis, A , Barr, AR , Kretschmer, JM , Manning, S , Jefferies, SP , Chalmers, KJ , Islam, AKM , and Langridge, P , 2003. Mapping and QTL analysis of the barley population Clipper x Sahara , Aust. J. Agric. Res. 54 (2003), pp. 1137–1140.
- Kaur, S , Nicolas, ME , Ford, R , Norton, RM , and Taylor, PWJ , 2006. Selection of Brassica rapa genotypes for tolerance to boron toxicity , Plant Soil. 285 (2006), pp. 115–123.
- Langowski, L , Růzicka, K , Naramoto, S , Kleine-Vehn, J , and Friml, J , 2010. Trafficking to the outer polar domain defines the root-soil interface , Curr Biol. 20 (2010), pp. 904–908.
- Miwa, K , Takano, J , and Fujiwara, T , 2006. Improvement of seed yields under boron-limiting conditions through overexpression of BOR1, a boron transporter for xylem loading, in Arabidopsis thaliana , Plant J. 46 (2006), pp. 1084–1091.
- Miwa, K , Takano, J , Omori, H , Seki, M , Shinozaki, K , and Fujiwara, T , 2007. Plants tolerant of high boron levels , Science. 318 (2007), p. 1417.
- Nable, RO , 1988. Resistance to boron toxicity amongst several barley and wheat cultivars – a preliminary examination of the resistance mechanism , Plant Soil. 112 (1988), pp. 45–52.
- Nable, RO , Bañuelos, GS , and Paull, JG , 1997. Boron toxicity , Plant Soil. 193 (1997), pp. 181–198.
- Nakagawa, Y , Hanaoka, H , Kobayashi, M , Miyoshi, K , Miwa, K , and Fujiwara, T , 2007. Cell-type specificity of the expression of Os BOR1, a rice efflux boron transporter gene, is regulated in response to boron availability for efficient boron uptake and xylem loading , Plant Cell. 19 (2007), pp. 2624–2635.
- Nozawa, A , Takano, J , Kobayashi, M , von Wirén, N , and Fujiwara, T , 2006. Roles of BOR1, DUR3, and FPS1 in boron transport and tolerance in Saccharomyces cerevisiae , FEMS Microbiol. Lett. 262 (2006), pp. 216–222.
- Ochiai, K , Uemura, S , Shimizu, A , Okumoto, Y , and Matoh, T , 2008. Boron toxicity in rice (Oryza sativa L.). I. Quantitative trait locus (QTL) analysis of tolerance to boron toxicity , Theor. Appl. Genet. 117 (2008), pp. 125–133.
- Reid, R , 2007. Identification of boron transporter genes likely to be responsible for tolerance to boron toxicity in wheat and barley , Plant Cell Physiol. 48 (2007), pp. 1673–1678.
- Reid, R , and Fitzpatrick, K , 2009. Influence of leaf tolerance mechanisms and rain on boron toxicity in barley and wheat , Plant Physiol. 151 (2009), pp. 413–420.
- Ritchie, RJ , 2006. Consistent sets of spectrophotometric chlorophyll equations for acetone, methanol and ethanol solvents , Photosynth. Res. 89 (2006), pp. 27–41.
- Sutton, T , Baumann, U , Hayes, J , et al., 2007. Boron-toxicity tolerance in barley arising from efflux transporter amplification , Science. 318 (2007), pp. 1446–1449.
- Takano, J , Kobayashi, M , Noda, Y , and Fujiwara, T , 2007. Saccharomyces cerevisiae Bor1p is a boron exporter and a key determinant of boron tolerance , FEMS Microbiol. Lett. 267 (2007), pp. 230–235.
- Takano, J , Miwa, K , Yuan, L , von Wirén, N , and Fujiwara, T , 2005. Endocytosis and degradation of BOR1, a boron transporter of Arabidopsis thaliana, regulated by boron availability , Proc. Natl. Acad. Sci. USA. 102 (2005), pp. 12276–12281.
- Takano, J , Noguchi, K , Yasumori, M , Kobayashi, M , Gajdos, Z , Miwa, K , Hayashi, H , Yoneyama, T , and Fujiwara, T , 2002. Arabidopsis boron transporter for xylem loading , Nature 420 (2002), pp. 337–340.