Abstract
We investigated the mechanism of growth reduction of dicotyledonous halophyte Salicornia bigelovii under salinity stress by growing it at 0.005 to 500 mol m−3 sodium chloride (NaCl). The optimal range for growth of S. bigelovii was between 50 and 200 mol m−3 NaCl. A significant correlation was found between growth and water content, which indicated that water deficit was an important factor in growth reduction at both suboptimal and supraoptimal salinities. Abscisic acid (ABA) concentration of the shoot was negatively related to growth and water content, which suggested that ABA induced by water deficit may inhibit growth at both the suboptimal and supraoptimal salinities. The cause of water deficit at supraoptimal salinity might be caused by nutritional imbalance and osmotic stress due to the low osmotic potential of the external solution. However, limited salt uptake may be one of the causes of water deficit under suboptimal salinity. We discuss a sodium ion (Na+) specific deficit rather than salt deficit as another possible cause of water deficit.
Introduction
Salinity is one of the major abiotic stresses that reduce plant growth and crop productivity worldwide. More than 800 million hectares of land are salt-affected (including both saline and sodic soils), equating to more than 6% of the world's total land area. Some of the most serious examples of salinity occur in arid and semi-arid regions (Arzani Citation2008). The deleterious effects of salinity on plant growth are associated with a water deficit within the plant, toxic effects of ions, nutritional imbalance and oxidative stress (Glenn and Brown Citation1999).
Halophytes are defined as plants that live in naturally saline habitats or that complete their life cycle at a salt concentration of at least 200 mol m−3 sodium chloride (NaCl) (Flowers and Colmer Citation2008). For the purpose of increasing agricultural productivity on saline soil, research on halophytes has been advanced. There is a possibility of creating salt-tolerant crops through understanding the physiological mechanisms enabling halophytes to survive and maintain productivity in a saline environment (Glenn and Brown Citation1999; Ayala et al. Citation1996) or of identifying new salt-tolerant crops (Glenn and Brown Citation1999; Glenn et al. Citation1991).
Salicornia bigelovii is a succulent, C3 annual species that is found in coastal estuaries and is, arguably, the most salt-tolerant and the most productive species under seawater irrigation (Ayala and O’Leary Citation1995; Glenn and O’Leary Citation1985; Glenn et al. Citation1991). It has been used as a model plant to study the salt tolerance of halophytes and as a gene resource to improve crop salinity tolerance. Moreover, its seed has been recognized as a new fodder or food, because its composition resembles that of soybean (Glenn et al. Citation1991).
Highly salt-tolerant dicotyledonous halophytes classified as euhalophytes, including Salicornia bigelovii, have an optimal salinity for growth (Ayala and O’Leary Citation1995; Flowers and Colmer Citation2008; Glenn and Brown Citation1999; Yeo and Flowers Citation1980). Maximum growth is obtained in the range of 100 to 200 mol m−3 NaCl, and growth is reduced beyond that range. The physiological process behind the growth response, however, is still obscure. Glenn and O’Leary (Citation1984) reported that not only relative growth rate but also water content of euhalophytes was decreased at suboptimal and supraoptimal salinities. This suggests that euhalophytes fall into water deficit and exhibit a decrease in growth rate at suboptimal and supraoptimal salinities.
Abscisic acid (ABA) is an important phytohormone for both growth and water physiology when plants are subjected to abiotic stresses. Water stress triggers the production of ABA, which in turn causes stomatal closure and induces the expression of drought stress-related genes (Pierce and Raschke Citation1980; Seki et al. Citation2007). Abscisic acid also inhibits shoot growth (Watts et al. Citation1981; Wilkinson and Davies Citation2002; Zhang and Davies Citation1990).
In the present study, we investigated the relationship between growth reduction, water relations and ABA concentration at suboptimal and supraoptimal salinities. Moreover, the effect of ionic and water relations on the growth suppression process at suboptimal and supraoptimal salinities is discussed.
Materials and methods
Plant materials
Seeds of Salicornia bigelovii in plastic pots filled with vermiculite were watered with deionized water until germination. After germination, seedlings were watered for five weeks with nutrient solution [composition in mol m−3: 2 N (NH4NO3), 0.4 P (KH2PO4), 1.6 Ca (CaCl2 · 2H2O), 2 Mg (MgSO4 · 7H2O); in g m−3: 2 Fe (FeSO4 · 7H2O), 0.5 Mn (MnSO4 · 5H2O), 0.2 B (B(OH)3), 0.1 Zn (ZnSO4 · 7H2O), 0.01 Cu (CuSO4 · 5H2O), 0.005 Mo ((NH4)6Mo7O24 · 4H2O)]. The pH of the solution was adjusted to 5.5 with a potassium hydroxide (KOH) solution. The sodium ion (Na+) concentration of nutrient solution was maintained below 0.005 mM during the period. Six-week-old plants were transferred to 4 -L plastic pots (five plants per pot) and grown in aerated nutrient solution for five weeks. The nutrient solution was replaced every week. Eleven-week-old plants were subjected to salinity treatments (nutrient solution with 0.005, 0.05, 0.5, 5, 50, 100, 200, 350, 500 mM NaCl) for four weeks. Fifteen plants were used for each treatment. At the end of the treatment, five plants per treatment were weighed and dried at 70°C for 48 h to determine dry weight (DW) and water content. Water content was calculated as:where FW is fresh weight. Another eight plants per treatment were weighed and quickly frozen with liquid nitrogen (N2) and stored at −70°C. This procedure was accomplished within 3 min. These plants were used for measurement of osmotic pressure and concentration of ABA.
Determination of abscisic acid concentration
Abscisic acid concentration was measured as described by Zeevaart and Milborrow (Citation1976). Frozen tissue was extracted by grinding with 4 mL acetone. An internal standard of 10 ng of [3′,5′,7′-2H6] ABA (Cambridge Isotope Laboratories Inc., USA) was added to each homogenate. Plant material was soaked in the extraction medium for 30 min at 4°C and centrifuged (5000 rpm, 10 min). After removing the supernatant, it was soaked again in 4 mL acetone for 15 min and centrifuged. Supernatants were mixed and dried by rotary evaporator at 35°C. The residue was dissolved in 10 mL of 0.1 M sodium sulfate (Na2SO4) solution and the pH adjusted to 2.8 with 1 M hydrogen chloride (HCl). Initial purification was achieved using a partition procedure with ethyl acetate. The ethyl acetate layer containing ABA was further purified by thin layer chromatography (TLC). After concentration to 50 µL by rotary evaporator at 35°C, the ethyl acetate layer was applied to Merck pre-coated silica gel 60 F254 plates and developed in a mobile phase of ethyl acetate, toluene and acetic acid (15/25/2, v/v). The ABA spot of the sample, located by co-chromatography with marker spots confirmed by ultraviolet (UV) (254 nm), was removed from the TLC plate by elution with 0.5 mL acetone. Purified ABA was methylated by addition of 0.2 mL ethereal diazomethane (Monson Citation1971). The sample was dried by vacuum desiccation and dissolved with 10 µL acetone. Abscisic acid was quantified by gas chromatography-mass spectrometry (GC-MS) (GC-17, Shimadzu, Kyoto, Japan; JMS-AMSUN, JEOL, Tokyo, Japan). The peak area ratio of m/z 190 to m/z 194 detected by the electron impact selected ion monitoring (EI-SIM) method (Rajasekaran et al. Citation1982) was used for calculation of ABA content.
Determination of minerals and inorganic anions
To determine sodium (Na), potassium (K), magnesium (Mg) and calcium (Ca) concentrations, dried shoots and roots were decomposed by sulfuric acid (H2SO4) and hydrogen peroxide (H2O2) at 200°C and diluted with deionized water. To prevent chemical interference, lanthanum (La) chloride (1000 ppm La) was added to Mg2+ and Ca2+ samples. Inorganic cations were quantitatively analyzed by atomic absorption spectrometry (Polarization Zeeman Atomic Absorption Spectrophotometer Z-6100, Hitachi, Tokyo, Japan). The total mineral concentration was calculated as the sum of the Na, K, Mg and Ca concentrations (Glenn and O’Leary Citation1984). Chloride (Cl−), nitrate (), sulfate (
), and phosphate (
) were extracted from dried plants by water at 80°C for 2 h. After filtration, ions were quantitated by ion chromatography (HIC-10Asuper, Shimadzu, Kyoto, Japan).
Determination of osmotic potential
Frozen samples were ground and centrifuged (10,000 rpm, 1 min). The osmotic potential of the supernatants was measured by a vapor pressure osmometer (VAPRO Osmometer 5520, Wescor Inc., UT, USA). The osmotic potential of culture solutions containing NaCl was measured directly by a vapor pressure osmometer.
Statistical analysis
Statistical analyses were carried out using PASW Statistics 18.0.0 software (SPSS Inc., IL, USA). Correlations between different variables were assessed by calculating Pearson correlation coefficients.
Results
Plant growth, water content and abscisic acid concentration
Growth was maximum in the range of 50 to 200 mol m−3 NaCl, and was reduced beyond this range (). We defined the salinity ranges of 0.005 to 5, 50 to 200 and 350 to 500 mol m−3 NaCl as suboptimal, optimal and supraoptimal salinities, respectively. At 0.005 mol m−3 NaCl, six of the 15 plants died during the salinity treatment period, which was a higher mortality than that of the other treatments (zero to two of the 15 plants), and the DW of shoots and roots was the smallest of all treatments. These results mean that low salinity is stressful for Salicornia bigelovii.
Figure 1 (a) Fresh weight (FW), (b) dry weight (DW) and (c) water content of shoots and roots of Salicornia bigelovii plants subjected to salt (NaCl) treatment for 28 days. (d) Relationship between DW and water content. Data points represent mean ± standard errors (n = 9–13 for FW, n = 5 for DW and water content). The correlation coefficient was significant according to Pearson's test at p < 0.01(**).
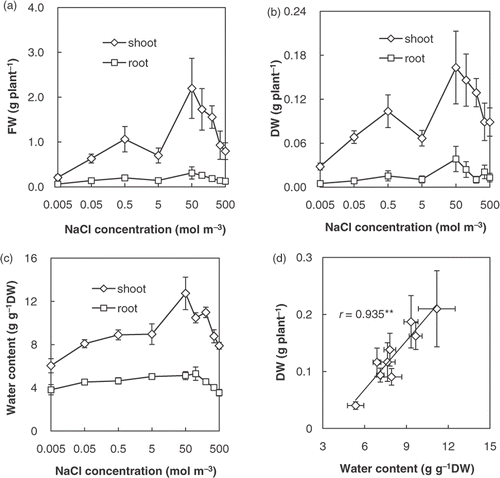
Water content was higher at optimal salinity than at suboptimal and supraoptimal salinities (). There was a positive relationship between DW and water content (). This suggests that Salicornia bigelovii was under water deficit at suboptimal and supraoptimal salinities, and we assumed that water deficit was the cause of the growth reduction. To confirm this assumption, we measured the ABA concentration of shoots. Abscisic acid concentration at optimal salinity was lower than at suboptimal and supraoptimal salinities (). The ABA concentration of shoots decreased rapidly with a rise in salinity in the range of 0.005 to 0.5 mol m−3 NaCl. Abscisic acid concentration was negatively correlated with growth and water content (). These results support the interpretation that S. bigelovii was under water deficit at suboptimal and supraoptimal salinities and that water deficit was the cause of growth reduction.
Figure 2. (a) Endogenous abscisic acid (ABA) concentration of shoots of Salicornia bigelovii subjected to salt (NaCl) treatment for 28 days. Data points represent mean ± standard errors (n = 3). (b) Relationship between dry weight (DW) and ABA concentration in shoot. (c) Relationship between water content and ABA concentration in shoot. The correlation coefficients were significant according to Pearson's test at p < 0.01(**).
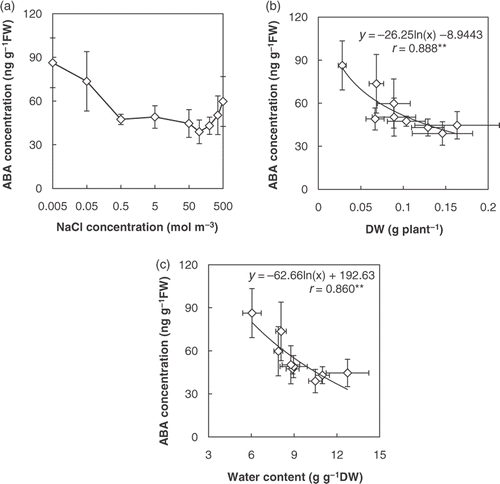
Minerals and inorganic anions
The primary mineral in shoots was K in the treatment range of 0.005 to 5 mol m−3 NaCl and was Na in the range of 50 to 500 mol m−3 NaCl (). With increasing salinity, the Na concentration of shoots increased and the K, Ca and Mg concentrations of shoots decreased at supraoptimal salinity. The total mineral concentration of shoots increased rapidly with a rise in salinity in the range of 50 to 500 mol m−3 NaCl (). The primary inorganic anion was Cl− at all ranges of salinity (). The and
concentrations of shoots decreased at supraoptimal salinity.
Figure 3 (a) Mineral concentrations [sodium (Na), potassium (K), magnesium (Mg), calcium (Ca)], (b) total mineral concentration and (c) inorganic anion concentrations [chloride (Cl−), nitrate (), sulfate (
), and phosphate (
)] of shoots of Salicornia bigelovii plants subjected to salt (NaCl) treatment for 28 days. Data points represent mean ± standard errors (n = 5).
![Figure 3 (a) Mineral concentrations [sodium (Na), potassium (K), magnesium (Mg), calcium (Ca)], (b) total mineral concentration and (c) inorganic anion concentrations [chloride (Cl−), nitrate (), sulfate (), and phosphate ()] of shoots of Salicornia bigelovii plants subjected to salt (NaCl) treatment for 28 days. Data points represent mean ± standard errors (n = 5).](/cms/asset/42753e32-ee5b-49a4-878d-541f70699d46/tssp_a_597036_f0003.gif)
Osmotic potential
The osmotic potential of roots was lower than that of the nutrient solutions containing 0.005 to 200 mol m−3 NaCl, but there was almost no difference at supraoptimal salinity (). This observation suggested that roots were affected by osmotic stress at supraoptimal salinity.
Discussion
Growth reduction and abscisic acid accumulation
Salicornia bigelovii has suboptimal, optimal and supraoptimal salinities on its growth. Similar trends have been reported for many dicotyledonous halophytes (Ayala and O’Leary Citation1995; Glenn and O’Leary Citation1984; Yeo and Flowers Citation1980). We found a positive relationship between growth and water content (). This result suggests that S. bigelovii was under water deficit at suboptimal and supraoptimal salinities, and we assumed water deficit was the cause of the growth reduction. This idea was supported by the negative correlation between ABA concentration and growth or water content ().
Although ABA inhibits shoot growth (Watts et al. Citation1981; Wilkinson and Davies Citation2002; Zhang and Davies Citation1990), the general growth control process affected by ABA accumulation has not been sufficiently clarified. One possible cause of the growth suppression by ABA is the inhibition of photosynthesis (Montero et al. Citation1998; Sibole et al. Citation1998). Abscisic induced by water deficit brings about stomatal closure, decreases carbon dioxide (CO2) concentration in the leaf, and restricts photosynthesis. However, in Salicornia bigelovii, the photosynthesis rate did not decrease at either suboptimal or supraoptimal salinity compared with optimal salinity (Ayala and O’Leary Citation1995). This indicates that growth suppression caused by ABA may not result from inhibition of photosynthesis.
In many glycophytes, high salinity increased ABA concentrations (Cramer and Quarrie 2002; Kefu et al. Citation1991; Montero et al. Citation1998). However, ABA accumulation under low salt conditions has also been reported in the Chenopodiaceous halophytes Atriplex spongiosa (Kefu et al. Citation1991), Suaeda maritima (Clipson et al. Citation1988) and salt tolerant barley (Veselov et al. Citation2008). Abscisic acid accumulation under low salt conditions is a unique property of halophytes and a part of the salt tolerant glycophytes. Cramer and Quarrie (2002) discussed that the steady-state-ABA concentrations were lowest under optimal salinity for growth (dependent upon species). These suggest that ABA accumulation under suboptimal salinity may be related to salt tolerance in these plant species.
Water deficit and abscisic acid accumulation
Abscisic acid accumulation is induced by turgor reduction, which is caused by water deficit (Pierce and Raschke Citation1980; Veselov et al. Citation2008). At supraoptimal salinity, decreasing turgor of root cells caused by a low osmotic potential of the nutrient solution may trigger ABA synthesis. Abscisic acid synthesized in roots may be transported in the xylem and accumulate in shoots (Kefu et al. Citation1991; Wilkinson and Davies Citation2002). Under supraoptimal salinity, one cause of ABA accumulation might be osmotic stress from the external solution. At suboptimal salinity, because roots were not subjected to osmotic stress, ABA accumulation in shoots might be brought about by other causes. We assume ABA was synthesized in response to water deficit in shoots and accumulated there.
Role of abscisic acid at suboptimal salinity
Abscisic acid is an important phytohormone related to abiotic stresses including salt stress. Abscisic acid accumulation causes stomatal closure, induces the expression of drought stress-related genes (Pierce and Raschke Citation1980; Seki et al. Citation2007) and inhibits shoot growth (Watts et al. Citation1981; Wilkinson and Davies Citation2002; Zhang and Davies Citation1990). These responses against ABA are understandable for supraoptimal salinity, but it is unclear that the same responses are induced by ABA at suboptimal salinity. Recently, Cabot et al. (Citation2009) reported that ABA decreases leaf Na+ exclusion and accumulates Na+ in leaf in salt-treated Phaseolus vulgaris. Because of the importance of Na+ for maintaining osmotic potential of Salicornia bigelovii low, it was possible that accumulated ABA could lead to lower shoot Na+ exclusion at suboptimal salinity.
Cause of water deficit at supraoptimal salinity
At supraoptimal salinity, one of the factors suppressing growth is water deficit caused by osmotic stress (). However, Ayala and O’Leary (Citation1995) concluded that excessive Na, which decreases K, Mg and Ca uptake, is one of the causes of growth reduction. In fact, Na concentration increased drastically and K, Mg, Ca, and
decreased with increasing salinity (, Ayala and O’Leary 1995). These observations suggest that multiple factors, including water deficit and ion uptake, might bring about growth reduction at supraoptimal salinity.
Cause of water deficit at suboptimal salinity
Ayala and O’Leary (Citation1995) found a decrease in leaf turgor and reduction in stem diameter caused by a decrease in cell size at suboptimal salinity. We found a decrease in water content and accumulation of ABA at suboptimal salinity ( and ). These observations suggest that Salicornia bigelovii was under water deficit at suboptimal salinity. In spite of the total mineral concentration at suboptimal salinity being much lower than those at optimal salinity (), the osmotic potential was unchanged (). This is the result of a decrease in water content () maintaining osmotic potential. Glenn and O’Leary (Citation1984) reported that the water content of halophytes adjusted passively to salt concentration to keep the osmotic potential low. We agree with their conclusions, and the limited salt uptake at an insufficient salt concentration in external solution is one of the causes of water deficit under suboptimal salinity.
Another possibility causing a water deficit at suboptimal salinity is a Na+ specific deficit rather than a salt deficit. In highly salt-tolerant dicotyledonous halophytes, Na+ is accumulated positively into the vacuole by a H+-ATPase and Na+/H+ antiporter located in the tonoplast (Ayala et al. Citation1996; Debez et al. Citation2006; Kefu et al. Citation2003; Parks et al. Citation2002). By sequestering Na+ (and also Cl−) into the vacuole, the low osmotic potential of the vacuole is maintained, and Na+ sensitive metabolic reactions in the cytoplasm are protected (Glenn and Brown Citation1999). Moreover, the growth stimulation by Na+ cannot be entirely substituted by K+ (Yeo and Flowers Citation1980). These observations suggest that Na+ has specific functions in halophytes and that Na+ deficit may suppress growth, as shown by Na+ being accumulated at the growing upper part of shoots of Salicornia bigelovii (Kudo et al. Citation2010).
Acknowledgments
The authors gratefully thank the Japan Society for the Promotion of Science for supporting the study through its Global Center of Excellence for Dryland Science program.
References
- Arzani, A , 2008. Improving salinity tolerance in crop plants: a biotechnological view , Vitro Cell. Dev. Biol.-plant. 44 (2008), pp. 373–383.
- Ayala, F , and O’Leary, JW , 1995. Growth and physiology of Salicornia bigelovii Torr. at suboptimal salinity , Int. J. Plant Sci. 156 (1995), pp. 197–205.
- Ayala, F , O’Leary, JW , and Schumaker, KS , 1996. Increased vacuolar and plasma membrane H+-ATPase activities in Salicornia bigelovii Torr. in response to NaCl , J. Exp. Bot. 47 (1996), pp. 25–32.
- Cabot, C , Sibole, JV , Barcelo, J , and Poschenrieder, C , 2009. Abscisic acid decreases leaf Na+ exclution in salt-treated Phaseolus vulgaris L , J. Plant Regul. 28 (2009), pp. 187–192.
- Clipson, NJW , Lachno, DR , and Flowers, TJ , 1988. Salt tolerance in the halophyte Suaeda maritima L Dum.: abscisic acid concentrations in response to constant and altered salinity , J. Exp. Bot. 207 (1988), pp. 1381–1388.
- Cramer, GR , and Quarrie, SA , 2002. Abscisic acid is correlated with the leaf growth inhibition of four genotypes of maize differing in their response to salinity , Funct. Plant Biol. 29 (2002), pp. 111–115.
- Debez, A , Saadaoui, D , Ramani, B , Ouerghi, Z , Koyro, HW , Huchzermeyer, B , and Abdelly, C , 2006. Leaf H+-ATPase activity and photosynthetic capacity of Cakile maritima under increasing salinity , Environ. Exp. Bot. 57 (2006), pp. 285–295.
- Flowers, TJ , and Colmer, TD , 2008. Salinity tolerance in halophytes , New Phytol. 179 (2008), pp. 945–963.
- Glenn, EP , and Brown, JJ , 1999. Salt tolerance and crop potential of halophytes , Crit. Rev. Plant Sci. 18 (2) (1999), pp. 227–255.
- Glenn, EP , and O’Leary, JW , 1984. Relationship between salt accumulation and water content of dicotyledonous halophytes , Plant Cell Environ. 7 (1984), pp. 253–261.
- Glenn, EP , and O’Leary, JW , 1985. Productivity and irrigation requirements of halophytes grown with seawater in the Sonoran Desert , J. Arid Environ. 9 (1985), pp. 81–91.
- Glenn, EP , O’Leary, JW , Watson, MC , Thompson, TL , and Kuehl, RO , 1991. Salicornia bigelovii Torr.: an oilseed halophyte for seawater irrigation , Science 251 (1991), pp. 1065–1067.
- Kefu, Z , Hai, F , San, Z , and Jiea, S , 2003. Study on the salt and drought tolerance of Suaeda salsa and Kalanchoe claigremontiana under iso-osmotic salt and water stress , Plant Sci. 165 (2003), pp. 837–844.
- Kefu, Z , Munns, R , and King, RW , 1991. Abscisic acid levels in NaCl-treated barley, cotton and saltbush , Aust. J. Plant Physiol. 18 (1991), pp. 17–24.
- Kudo, N , Sugino, T , Oka, M , and Fujiyama, H , 2010. Sodium tolerance of plants in relation to ionic balance and the absorption ability of microelements , Soil Sci. Plant Nutr. 56 (2010), pp. 225–233.
- Monson, RS , 1971. Advanced Organic Synthesis . London: Academic Press; 1971. pp. 155–156.
- Montero, E , Cabot, C , Poschenrieder, CH , and Barcelo, J , 1998. Relative importance of osmotic-stress and ion-specific effects on ABA-mediated inhibition of leaf expansion growth in Phaseolus vulgaris , Plant Cell Environ. 21 (1998), pp. 54–62.
- Parks, GE , Dietrich, MA , and Schumaker, KS , 2002. Increased vacuolar Na+/H+ exchange activity in Salicornia bigelovii Torr. in response to NaCl , J. Exp. Bot. 53 (2002), pp. 1055–1065.
- Pierce, M , and Raschke, K , 1980. Correlation between loss of turgor and accumulation of abscisic acid in detached leaves , Planta 148 (1980), pp. 174–182.
- Rajasekaran, K , Vine, J , and Mullins, MG , 1982. Dormancy in stomatic embryos and seeds of Vitis: changes in endogeneous abscisic acid during embryogeny and germination , Planta 154 (1982), pp. 139–144.
- Seki, M , Umezawa, T , Urano, K , and Shinozaki, K , 2007. Regulatory metabolic networks in drought stress responses , Curr. Opin. Plant Biol. 10 (2007), pp. 296–302.
- Sibole, JV , Montero, E , Cabot, C , Poschenrieder, CH , and Barcelo, J , 1998. Role of sodium in the ABA-mediated long-term growth response of bean to salt stress , Physiol Plant 104 (1998), pp. 299–305.
- Veselov, DS , Sharipova, GV , Veselov, SU , and Kudoyarova, GR , 2008. The effects of NaCl treatment on water relations, growth, and ABA content in barley cultivars differing in drought tolerance , J. Plant Regul. 27 (2008), pp. 380–386.
- Watts, S , Rodriguez, JL , Evans, SE , and Davies, WJ , 1981. Root and shoot grows of plants treated with abscisic acid , Ann. Bot. 47 (1981), pp. 595–602.
- Wilkinson, S , and Davies, WJ , 2002. ABA-based chemical signalling: the co-ordination of responses to stress in plants , Plant Cell Environ. 25 (2002), pp. 195–210.
- Yeo, AR , and Flowers, TJ , 1980. Salt tolerance in the halophyte Suaeda maritima L. Dum.: evaluation of the effect of salinity upon growth , J. Exp. Bot. 31 (1980), pp. 1171–1183.
- Zeevaart, JAD , and Milborrow, BV , 1976. Metabolism of abscisic acid and the occurrence of epi-dihydrophaseic acid in Phaseolus-vulgaris , Phytochemistry 15 (4) (1976), pp. 493–500.
- Zhang, J , and Davies, WJ , 1990. Changes in the concentration of ABA in xylem sap as a function of changing soil water status can account for changes in leaf conductance and growth , Plant Cell Environ. 13 (1990), pp. 277–285.