Abstract
This study was conducted to investigate the relationship between available phosphorus (P) in granitic regosols and the population and composition of phosphate solubilizing bacteria (PSB) enhanced by compost amendments. Enrichment of soil with rock phosphate (RP) or no enrichment was combined with poultry manure (PM), cattle manure (CM), sewage sludge (SS), or P-adjusted sawdust (PSD) compost amendments at a rate equivalent to 5 mg P kg−1 soil, or without compost amendment. Soil was enriched with RP at 0.25 or 0.5 g kg−1 soil in the pot experiment and 0.5 g kg−1 soil in the incubation experiment. The amended soils were used to grow African millet (Eleusine coracana Gaertn, cv. Yukijirushi) for 30 days in Wagner's pots (200 cm2). Plant P uptake in RP-enriched soil at 0.25 g RP kg−1 soil was 2- 3-fold greater with compost addition than without compost. The results of the incubation experiment showed that compost amendments significantly enhanced microbial biomass P and population density of PSB, which showed significant positive correlation with the available P in soil. The dominant isolates of PSB in all the compost treatments were closest to Burkholderia fungorum. The treatment-specific isolates of PSB with the type of compost amendment were closely related to Burkholderia phytofirmans in PM treatment, Burkholderia caledonica or Arthrobacter ramosus in SS treatment and Burkholderia phenazinium in PSD treatment. The enhanced available P in RP-enriched treatments could be related to specific isolates of PSB in each compost treatment.
Introduction
Phosphate availability to plants is often low due to poor solubility of most phosphorus (P) compounds and high P fixation capacity of soils, although the total amount of P is high in many soils (Hoberg et al. Citation2005; Khan et al. Citation2006). The amount of available P in soil is maintained by a continuous supply of P fertilizer which leads to a buildup of high soil P levels over time. Efficient utilization of P fertilizer and recycling of accumulated soil P is important because known phosphate deposits are a finite resource (Mengel Citation1997).
Ayaga et al. (Citation2006) found that crop yield was significantly increased by combined use of organic and inorganic fertilizers in the low-input cropping system. Organic amendments prevent rapid fixation of available P in soil by blocking P adsorption sites (Haynes and Mokolobate Citation2001) resulting in greater concentration of available P in soil. Wu et al. (Citation2007) reported that organic amendments enhanced microbial biomass P and transformation of P. Therefore the adsorption or fixation of easily soluble P is prevented by enhanced microbial biomass (Khan and Joergensen Citation2009). The results of Kouno et al. (Citation2002) illustrated the large potential of microbial biomass P turnover as a source of P for plants, which explains efficient utilization of P fertilizer with organic amendments to the soil.
Enhanced P uptake by plants in rock phosphate (RP)-enriched soils with organic amendments was reported by Hellal et al. (Citation2009). The addition of organic matter enhances the density of microorganisms (Nishio and Kusano Citation1980) including phosphate-solubilizing microorganisms, which mobilize the non-labile P by secretion of organic acids such as citric acid, gluconic acid or lactic acid (Chen et al. Citation2006). The increased population density of phosphate-solubilizing microorganisms by organic amendments may be partially responsible for an increased level of P uptake by plants. The commonly isolated phosphate solubilizing bacteria (PSB) generally belong to the genera Bacillus or Pseudomonas (Peix et al. Citation2004; Chen et al. Citation2006). In addition, a number of fungal species solubilize P; these species generally belong to the genera Aspergillus or Penicillium (Reyes et al. Citation1999; Vazquez et al. Citation2000). The significant positive correlation between utilization of RP by plants and the population density of PSB enhanced by compost amendments in Andosols was shown by Wickramatilake et al. (Citation2010).
Compost is a microbiologically active product characterized by a particular endogenous microflora (Saison et al. Citation2006). The techniques of molecular biology offer new opportunities for analyzing changes in soil microbial communities (Dilly et al. Citation2004). However, little information is available on how compost amendments, especially the compost-borne microorganisms, influence the relationship between soil P bioavailability and the composition of soil microbial communities.
Granitic regosols (Classification Committee of Cultivated Soils Citation1996) have relatively low microbial biomass content (Herai et al. Citation2006) and microbial activity (Lukito et al. Citation1997) due to their very low levels of organic matter (Egashira et al. Citation1985) and plant nutrients. This soil is widely distributed in the Chugoku district of Japan (Lukito et al. Citation1998; Chowdhury et al. Citation1999) and is amended with chemical phosphate fertilizer in excess of crop requirements, because of its very low P levels. Application of P in excess of crop requirement leads to an accumulation in the plough layer of soil. The availability of accumulated P in soil could be increased by enhanced biological properties of soil by means of compost amendments. In this study, virgin soil was enriched with RP instead of accumulated P to eliminate the effects of organic P mineralization.
With an emphasis on the use of soil microorganisms to improve the availability of accumulated soil P or enhance fertilizer use efficiency, this study was conducted to investigate the effects of compost amendment of soil on plant utilization of RP and to identify the relationship between available P in RP-enriched soil and the population and composition of the PSB enhanced by compost amendments.
Materials and Methods
Soil and soil amendments
Granitic regosols that had never received any P fertilizer amendment were collected from Fukuyama City, Hiroshima Prefecture, Japan. The soil is classified as a Regosol (IUSS Working Group WRB Citation2006). The chemical and physical properties of the soil are listed in .
Table 1. Chemical and physical properties of soil used in the experiment
The four types of compost added to the soil were poultry manure compost (PM), cattle manure compost (CM), sewage sludge compost (SS), and P-adjusted sawdust compost (PSD). Total P content was extremely low in sawdust compost compared with PM, CM or SS. Therefore, the weight of sawdust compost incorporated into the soil was controlled by mixing it with autoclaved crab waste at a ratio of 1:1 by weight, because compost was amended to the soil at a fixed rate of P by each type of compost. Crab waste is a P-rich organic amendment and commercially available in the Chugoku district of Japan. Composts were crushed to a particle size of <2 mm before application. The chemical and biological properties of the various composts are presented in .
Table 2. Chemical and biological properties of compost used as compost treatments in the experiment
The various types of compost were obtained from composting centers located in Hiroshima Prefecture. Basically, these were produced by composting in heaps that were turned periodically during 2–3 months until they reached maturity. PM compost was made from poultry manure and poultry litter. The CM compost was produced from dairy cattle manure and straw bedding. The stabilized sewage sludge manure was the product of a municipal wastewater treatment facility and the sawdust compost was produced from the residue of culture media for Maitake [Grifola frondosa (Dicks.: Fr.) SF Gray] mushrooms.
RP was obtained from China and was ground to a particle size of <1 mm before application. The total P content in the RP was 150 g P kg−1 RP and the citric acid-soluble P content was 17.4 g P kg−1 RP.
Treatments and experimental design
Pot experiment
In the pot experiment, the four compost-amendment treatments (PM, CM, SS or PSD) or no-compost treatment (NC) were combined with RP enrichment (RP+) or without RP enrichment (RP−). RP was added to the soil at rates of 0.25 RP kg−1 soil (L1) or 0.5 g RP kg−1 soil (L2). Each type of compost was added to the soil at a rate equivalent to 5 mg P kg−1 soil (0.16 g of PM, 0.49 g of CM, 0.29 g of SS, or 0.37 g of PSD kg−1 of soil). Each of the treatments was supplemented with dry coffee residue (CR) containing 49% carbon (C) and 0.08% P w/w as a C source at a rate of 0.6 g C kg−1 soil for enhancing soil microorganisms. Compost was amended to the soil as a source of microorganisms at a very low rate (less than 0.05% w/w) to minimize plant P uptake from compost. Each Wagner's pot (200 cm2) was filled with an amount of soil equivalent to 4 kg of dry soil. The experiment was conducted with 3 replicates for each treatment. To ensure that other nutrients were not limiting, the soil in each pot was supplemented with N as (NH4)2SO4 (0.08 g N kg−1 soil), K as K2SO4 (0.03 g K kg−1 soil) and dolomite (0.3 g dolomite kg−1 soil). African millet (Eleusine coracana Gaertn, cv. Yukijirushi) was sown at a rate of 100 seeds per pot. The pots were kept in a glasshouse at 25°C from June 26 to July 26, 2007.
Plant shoots and roots were sampled separately, dried at 80°C for at least 48 hours, weighed, and stored for chemical analysis. Soil samples taken at the completion of the pot experiments were sieved (<2 mm) after sampling and were air dried for chemical analysis.
Incubation experiment
In the incubation experiment, the four compost-amendment treatments (PM, CM, SS or PSD) or NC were combined with RP+ or RP−. RP was added to the soil at a rate of L2 (0.5 g RP kg−1 soil). Composts were added to the soil at the rate of 5 mg P kg−1 of soil (the same rate of application as in the pot experiment). The soil was also amended with a source of C (2000 mg C kg−1 of soil; glucose) together with an aliquot of N as (NH4)2SO4 (0.08 g N kg−1 of soil) and of K as K2SO4 (0.03 g K kg−1 of soil). The soil water content was adjusted to 50% of the water-holding capacity (WHC) by adding deionized water. The WHC was determined as explained by Bremner and Shaw (Citation1958). The experiment was conducted with 3 replicates for each treatment.
Portions of soil equivalent to 100 g on an oven-dried basis were placed in 120-mL glass bottles, and each bottle was placed in a 1-L jar containing 10 mL of distilled water, and incubated in darkness at 25°C. The CO2 evolved by soil microorganisms during the incubation was trapped by 1 M aqueous NaOH placed inside the jar. Soil samples were taken before incubation and on days 3, 7, 15, and 30 of incubation. Samples were subsequently stored either at 4°C for soil biological and chemical analyses, or at −20°C for the analysis of the composition of the bacterial community.
Chemical analysis
Plant samples
For P determination, plant samples (0.3 g) were digested until clear with a 1:1 mixture of concentrated H2SO4 and concentrated HNO3 (10 mL) in a Kjeldahl flask.
Soil, compost, and rock phosphate samples
Soil, compost, and RP samples (0.2 g each) were digested with concentrated HNO3 (8 mL) for 1 hour in a microwave digester system (Start D, Milestone, Sorisole, Italy) for chemical analysis. Available P from the soil was extracted with 0.5 M aqueous NaHCO3 (pH 8.5) (Olsen et al. Citation1954). One gram of soil sample was sequentially extracted with 1 M NH4Cl and 25 g L−1 acetic acid for calcium-bound P (Ca-P), 1 M NH4F for aluminum-bound P (Al-P), and 0.1 M NaOH for iron-bound P (Fe-P) (Hirata et al. Citation1999). Wet compost (2 g dry basis) was extracted with water (100 mL) for analysis of water-soluble P (Kleinman et al. Citation2007). RP (1 g) was extracted with 0.1 M citric acid (100 mL) for 30 minutes for the determination of citric acid-soluble P (Braithwaite et al. Citation1989). The P contents of the extracts were then determined spectrophotometrically (UVmini-1240, Shimadzu, Kyoto, Japan) by the ammonium molybdate–ascorbic acid method (Murphy and Riley Citation1962).
The total C and N contents of soil and compost samples were determined by a combustion method with a CN analyzer (Yanaco, MT-700, Kyoto, Japan).
Calculations
Within each of the five compost treatments, the plant RP utilization was calculated as follows:
The RP utilization efficiency was calculated as the proportion of RP utilization by plants relative to the total amount of P added to the soil as RP according to the following equation:
Biological analysis
Microbial biomass phosphorus
Microbial biomass P was extracted from the soil samples collected in the incubation experiment. Biomass P was measured as described by Brookes et al. (Citation1982). Briefly, soil samples were weighed separately into three 50-mL glass vials. The first set was extracted with Olsen reagent and the second set with Olsen reagent spiked with P added as KH2PO4 equivalent to 25 µg P g−1 oven-dry soil. The third set was fumigated with ethanol-free chloroform for 24 h at 25°C. The fumigant was removed, and the sample was extracted with Olsen reagent. A correction for P fixation during extraction was made by calculating the recovery of added P using the sample spiked with P. All extractions were performed after fumigation by using a 1:20 soil:Olsen reagent ratio and shaking at 150 rpm in an orbital incubator for 30 min at 25°C. Biomass P was estimated as the difference between the inorganic P of the fumigated and non-fumigated soil.
Analysis of microbial density
To determine the populations of PSB, serially diluted samples of compost and soil were plated onto National Botanical Research Institute phosphate (NBRIP) growth medium (Nautiyal Citation1999), and those bacterial colonies that were surrounded by clear zones were counted as PSB. The NBRIP agar medium (1 L) consisted of 10 g of glucose, 5 g of Ca3(PO4)2, 5 g of MgCl2 · 6H2O, 0.25 g of MgSO4 · 7H2O, 0.2 g of KCl, 0.1 g of (NH4)2SO4 and 20 g of agar. The pH of the media was adjusted to 7.0 before autoclaving. The NBRIP medium was supplemented with 100 µg mL−1 of cycloheximide (Sigma-Aldrich, St. Louis, MO, USA) (Jorquera et al. Citation2008) to inhibit growth of fungi. To determine the size of the total bacterial population, serially diluted compost samples were plated onto a nutrient agar made from a nutrient broth (Sigma-Aldrich, St. Louis, MO, USA).
Analysis of microbial community structure
The structures of the microbial communities in compost and in compost-amended soil at day 3 of soil incubation were determined by amplification of the 16S rDNA of the total bacterial community by the PCR, followed by denaturing gradient gel electrophoresis (DGGE).
DNA was extracted from 0.5 g of soil or 0.2 g of compost by using an ISOIL for Beads Beating Kit (Nippon Gene Co. Ltd., Tokyo, Japan), and samples were purified according to the manufacturer's instructions. Bacterial 16S rDNA was amplified from extracted DNA by the PCR technique. The primer pair 341F (GC) and 518R (Muyzer et al. Citation1993) was used to amplify the variable V3 region. The thermal cycle for the PCR was as follows: initial activation of polymerase at 95°C for 9 min, followed by 1 cycle of denaturing at 94°C for 1 min, annealing at 65°C for 1 min, and extension at 72°C for 3 min. Nineteen cycles were performed with a decrease in the annealing temperature of 1°C every second cycle until a temperature of 55°C was reached. Ten additional cycles were carried out at 55°C, and extension of the last cycle was at 72°C for 10 min. The mixtures (50 µL) for the PCR reaction consisted of 0.5 µM of each primer, 200 µM of each dNTP, 2.5 mM of MgCl2, 5 µL of 10 × PCR buffer, 1.25 units of AmpliTaq Gold (Applied Biosystems, Foster City, CA, USA), and 1 µL of DNA template. The analyses were conducted with two replicates for each sample.
DGGE was conducted using a DCode System (Bio-Rad Laboratories, Hercules, CA, USA) with an 8% (w/v) polyacrylamide gel (acrylamide/bisacrylamide, 37.5:1) in 1 × TAE (Tris base/acetic acid/EDTA) buffer. A denaturing gradient ranging from 30% to 70% was produced with 80% denaturant solution containing 5.6 M urea and 32% formamide. After each lane of the gel was loaded with 20 µL of the PCR products, electrophoresis was performed at a constant temperature of 60°C and a constant voltage of 100 V for 16 h. After electrophoresis, the gels were stained with SYBR Gold nucleic acid gel stain (Molecular Probes, Eugene, OR, USA). Gel images and photographs were recorded by using an UV transillumination system with a Print-graph AE-6932GXCF (Atto Corporation, Tokyo, Japan). The DGGE bands were visualized using the Safe Imager™ blue light transilluminator (Invitrogen, Carlsbad, CA, USA) and selected specific bands appearing on the gel were excised for sequencing.
The excised pieces of DGGE gels were washed twice with 1 mL sterilized distilled water in a 1.5-mL tube. A portion of the gel piece (<1 mm3) was used as the direct template for a second PCR. The condition for the second PCR was the same as for the first PCR except that the forward primer had no GC clamp attached. The DNA fragments were sequenced using the both 341F and 518R primers.
Identification of dominant PSB populations
The dominant culturable PSB population was determined for samples on day 3 of soil incubation. The serially diluted samples were plated onto NBRIP medium and then 10 colonies of bacteria surrounded by clear zones were randomly picked using a sterile micropipette. Bacterial 16S rDNA was amplified from each colony of PSB by the PCR technique using the primer pair 984F and 1378R (Heuer et al. Citation1997). The thermal cycle for the PCR was as follows: initial activation of polymerase at 94°C for 5 min, followed by 30 cycles of denaturing at 94°C for 30 sec, annealing at 52°C for 1 min, extension at 72°C for 2 min, and extension of the last cycle at 72°C for 4 min. The mixtures (25 µL) for the PCR reaction consisted of 2 µM of each primer, 12.5 µL 2x Quick Taq (Toyobo, Osaka, Japan) and each colony of PSB as a template of DNA. The DNA fragments were sequenced using the 984F primer.
All the PCR amplification was carried out with a Gene-Amp PCR System 2700 (Applied Biosystems). PCR products were verified and quantified by agarose gel electrophoresis with a standard in the gel (Loading Quick, Toyobo) and were purified using QIAquick PCR purification kit (Qiagen, Hilden, Germany).
Phylogenetic analysis
The DNA fragments were sequenced by Basic Research and Development Center of Natural Sciences, Hiroshima University. The nucleotide sequences were evaluated using the BLAST program through the National Center for Biotechnology Information (Altschul et al. Citation1990) and Seqmatch program through the Ribosomal Database Project (Maidak et al. Citation1994). These sequence data have been submitted to the DDBJ/EMBL/GenBank databases under the accession numbers AB642595 to AB642599 and JF944814 to JF944818.
Statistical analysis
Data were statistically analyzed by using Minitab, release 14 (Minitab Inc., State College, PA, USA). The effect on shoots and roots dry weight of plants, uptake of P and RP utilization by plants were tested by two-way ANOVA with compost treatments and rate of RP as main factors and their interactions. One-way ANOVA was used to determine within-treatment differences at each rate of RP in the pot experiment or to determine within-treatment differences during the period of incubation in soil incubation experiment. Fisher's least significant difference method was used to identify treatments for which the mean values differed from one another at a 5% level of significance (p < 0.05).
Results
Plant dry matter and phosphorus uptake
The total plant (shoots and roots) dry matter increased as RP application rate increased. The increase in dry matter was 2.1 g pot−1 at the RP application rate of L1 and was 1.1- to 2.3-fold greater with than without compost treatments (NC treatment) ().
Table 3. Shoots and roots dry weight of plants, uptake of phosphorus (P), rock phosphate (RP) utilization and RP utilization efficiency by African millet (Eleusine coracana) grown with four compost amendment treatments and a non-compost control (NC) with RP addition (RP+) or without (RP−)
Table 4. Soil Available phosphorus (P) with four compost treatments and a non-compost control (NC) with rock phosphate addition (RP+) or without (RP−) during soil incubation
Shoots and roots P concentration also increased with RP application rate. P concentration in shoots ranged from 0.11% to 0.15% (w/w) without RP enrichment and increased to 0.24–0.29% and 0.28–0.34% at RP application rates of L1 and L2, respectively.
P uptake increased as RP application rate increased (), and the amount of P uptake increased (RP utilization) 1.4- to 2.1-fold more with than without compost amendment at the L1 RP application rate ().
RP utilization efficiency
RP utilization efficiency was 5.8% at the L1 RP application rate, and was greatly improved in the compost-amended soil; it was 12.1% with PM or CM treatments, 11.6% with SS treatment, and 8.1% with PSD treatment (). At the L2 RP application rate, utilization efficiency declined in compost-amended treatments and ranged from 7.8% to 9.1% with or without compost amendments.
Microbial biomass phosphorus and available phosphorus
The amount of microbial biomass P increased sharply from the start to day 3 of soil incubation in compost-amended treatments and increased gradually until day 15 in the NC treatment (). Biomass P in the PM, CM and SS treatments decreased after day 3 and increased again from days 7 to 15, especially in the RP-enriched treatments. Biomass P in all the treatments decreased thereafter. At day 15, soil microbial biomass P was significantly greater in each treatment with RP than in the treatment without RP. Total biomass P in RP-enriched treatments was significantly higher in the SS or the PSD treatments than in the NC treatment. However, the levels of microbial biomass P increase in RP-enriched soil with PM or CM during the 7th through 15th days of soil incubation was significantly higher than with NC treatment.
Figure 1. Changes in soil microbial biomass phosphorus (P) of the soil treatments during the soil incubation period. Soil treatments consisted of four compost-amended treatments and a no-compost control (×) with rock phosphate (RP) addition (solid line) or without (broken line). Compost-amended treatments: (□) poultry manure; (○) cattle manure; (△), sewage sludge; (⋄) phosphorus-adjusted sawdust. Statistically homogeneous values of biomass P at each sampling day are marked by same letter (one-way ANOVA, P < 0.05, Fisher's test). Values are mean of three replicates.
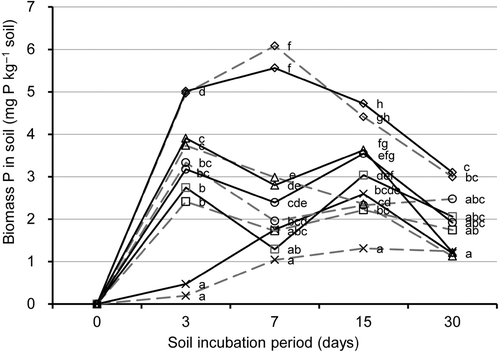
The amount of available P (Olsen) in all treatments decreased sharply from the start to day 3 of soil incubation and showed no drastic variations from days 3 to 15 (). Available P increased after day 15. Among the RP-enriched soils, available P was significantly greater in PM, CM or PSD treatments than in the NC treatment at day 15.
Population density of phosphate-solubilizing bacteria
The population density of PSB increased from the start to day 3 of soil incubation and declined sharply from days 3 to 7 (). The density of PSB slightly increased from days 7 to 15. RP enrichment with compost significantly enhanced density of soil PSB, but RP enrichment without compost did not (NC treatment). The density of PSB in RP-enriched soil was 1.3- to 3.3-fold, 4- to 13-fold, and 1.1- to 1.4-fold greater with than without compost at days 3, 7 and 15 of soil incubation, respectively.
Figure 2. Changes in population density of phosphate solubilizing bacteria (PSB) of the soil treatments during the soil incubation period. Soil treatments consisted of four compost-amended treatments and a no-compost control (×) with rock phosphate (RP) addition (solid line) or without (broken line). Compost-amended treatments: (□) poultry manure; (○) cattle manure; (△), sewage sludge; (⋄) phosphorus-adjusted sawdust. Statistically homogeneous values of population density of PSB at each sampling day are marked by same letter (one-way ANOVA, P < 0.05, Fisher's test). Values are mean of three replicates.
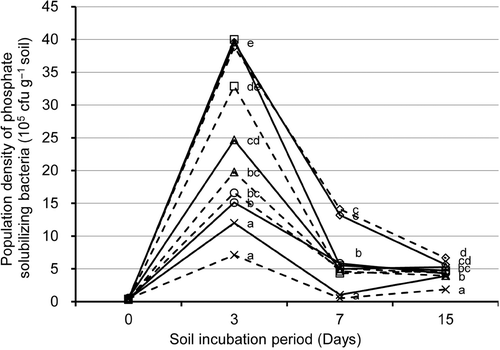
Bacterial community structure analysis with PCR-DGGE
The band positions of the DGGE profiles generated by the various types of compost showed differences in the composition of the bacterial communities present in the various composts (). The DGGE profiles developed for RP-enriched soil with PM, SS or PSD amendment treatments showed specific bands which did not appear in RP-enriched soil without compost amendment (). Bands B1 and B2 that appeared in RP-enriched soil of the PM treatment were closely related to Paenibacillus spp. (AB576894.1) (100%) and Paenibacillus alginolyticus (HQ236042.1) (96.2%), respectively. Bands B3 and B4 that appeared in RP-enriched soil of the SS treatment were most closely related to the uncultured bacterium (HM035995.1) (93.4–94.2%). Band B5 of the same treatment was also most closely related to the uncultured bacterium (EF174283.1) (95.5%). Comparison of partial 16S rDNA sequences with sequences held in the Ribosomal Database Project showed that the bands B3, B4 and B5 belonged to the genera Chitinophaga, Chitinophaga and Filimonas, respectively.
Figure 3. Bacterial 16S rDNA denaturing gradient gel electrophoresis profiles of the soil treatments on day 3 of soil incubation and four compost types used as soil amendments. Soil treatments consisted of four compost-amendment treatments and a no-compost control (NC) with rock phosphate addition (RP+) or without (RP−). Compost-amended treatments: PM, poultry manure; CM, cattle manure; SS, sewage sludge; PSD, phosphorus-adjusted sawdust. Arrows show specific bands that appeared in RP+ treatment with compost-amendment treatments. Bands B1 and B2 were closely related to the Paenibacillus spp. (AB576894.1) (100%) and Paenibacillus alginolyticus (HQ236042.1) (96.2%), respectively. Bands B3 and B4 were most closely related to the uncultured bacterium (HM035995.1) (93.4-94.2%). Band B5 was also most closely related to the uncultured bacterium (EF174283.1) (95.5%).
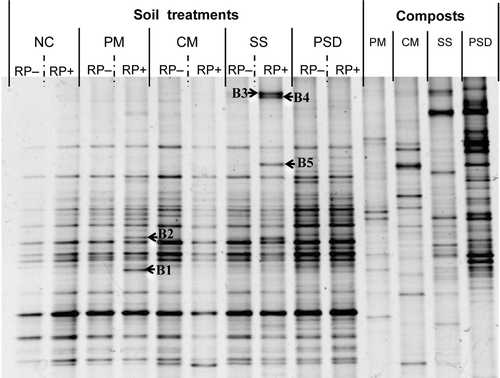
The dominant isolates of PSB
All random isolates from the NC or CM treatments showed almost the same sequence and were closely related to Burkholderia fungorum (AB091192.1) (99.7%), which was also the dominant isolate of PSB for the other compost-amendment treatments. Colonies that deviated from the dominant group were a single colony of the PM treatment, Burkholderia phytofirmans (AY836218.1) (99.7%); 2 colonies of the SS treatment, Burkholderia caledonica (GQ181032.1) (100%) and Arthrobacter ramosus (AM039435.1) (99.5%), and 2 colonies of the PSD treatment that showed the same sequence, Burkholderia phenazinium (GQ181022.1) (99.7%).
Discussion
Plant P uptake was low in the NC treatment without RP enrichment, and was inhibited by the limited availability of soil P (). Plant P uptake increased with increasing rate of RP application, showing that plant P uptake is effectively enhanced by RP enrichment of soil.
The increase of P uptake by RP enrichment was found to be greater in compost-amended soils than in unamended soils (). The increase differed significantly among the various compost treatments. The differences in RP utilization by plants at 2 rates of application were compared by calculating RP utilization efficiency.
Rock phosphate utilization efficiency
RP utilization efficiency was highest, and similar, in the PM and CM treatments, followed by SS and then by PSD treatments at the L1 RP application rate (). RP utilization efficiency declined at a higher RP application rate (L2) in the compost-amended treatments. This result is supported by the findings of Bolland and Barrow (Citation1988), who found that the relative effectiveness of RP declined as its rate of application increased.
Biologically mediated processes largely control the bioavailability of soil P (Zou et al. Citation1992). For example, the mobilization of mineral phosphate (Khan et al. Citation2006) and chelation of the cations associated with P (Iyamuremye et al. Citation1996) by organic acid secretions of microorganisms, or the temporary immobilization of P into microbial biomass which prevents fixation of labile P into non-labile P (Nishio Citation1996) could both enhance mobility of soil P.
A significant interaction between the compost treatments and the RP treatments on the plant P uptake was observed. We also observed that there were no significant differences (data not shown) in the initial soil pH values (1:2.5 of H2O) of compost-amended soils in the incubation experiment. We therefore assumed that the biological properties enhanced by combined use of RP with compost had significant effects on the variations in plant utilization of RP.
The relationship between the available P of RP-enriched soil and soil biological properties in relation to soil microbial biomass P, population densities of PSB, and composition of PSB community are discussed using the incubation experiment results.
Relationship between microbial biomass phosphorus and available phosphorus
The increase of microbial biomass P from the start through the 3rd day of soil incubation was mainly due to the P released by compost, because biomass P at day 3 was not significantly different between each pair of treatments with and without RP. However, the biomass P in each RP-enriched treatment was significantly higher compared to the treatment without RP at day 15. This result showed that the P from the RP-enriched soil was immobilized by the soil microbial biomass during the 7th through the 15th days of soil incubation ().
The strong positive correlation between RP utilization by plants and the microbial biomass P was shown by a previous study using Andosols (Wickramatilake et al. Citation2010). The results of this study show that the microbial biomass P at day 15 has a strong positive correlation with available P at day 30 of soil incubation (r = 0.71; p < 0.01) (). This shows that the biomass P is an important component of available P in soil. Soil microbial biomass is a major source of plant available P in soil (Kwabiah et al. Citation2003).
Figure 4. Relationships between the available phosphorus (P) (Olsen P) and soil biological properties: (4a) the microbial biomass P (r = 0.71, n = 30), (4b) the population density of phosphate-solubilizing bacteria (PSB) (r = 0.74, n = 30). The relationships were examined by means of an incubation experiment at day 30 for available P or day 15 for biological properties of soil.
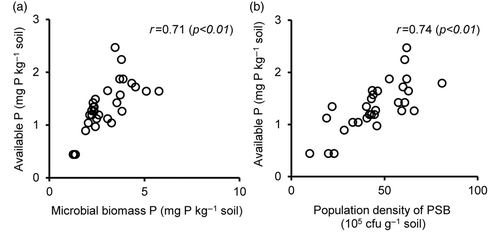
Relationship between population density of PSB and available phosphorus in soils
The addition of organic matter enhances the population density of PSB and then the solubilized P is utilized by the general microbial community (Nishio Citation1996). Soil incubation results showed that enriching compost-amended soil with RP significantly enhanced population density of PSB between days 7 to 15 in compost-amended soil but not in unamended soil (). Similarly, microbial biomass P sharply increased in the RP-enriched soil between days 7 and 15 especially in compost-amended soil. We can therefore say that P from RP is efficiently immobilized by microorganisms in the compost-amended treatments.
Available P among the RP-enriched soils was found to be significantly greater with PM, CM or PSD treatments at day 15 (). The differences in PSB population density during day 15 were positively correlated with available P at day 30 of soil incubation (r = 0.74, p < 0.01) (). This result is supported by the findings of previous study, which showed a similar relationship using Andosols (Wickramatilake et al. Citation2010).
Relationship between rock phosphate utilization and composition of PSB community
The banding patterns produced by the DGGE profiles showed that the soil bacterial community structure differed slightly between the RP-enriched soil and the RP-enriched soil with PM, SS, or PSD compost, which differed in bacterial community (). Bands that appeared in the PM treatment were closely related to the genus Paenibacillus. The phosphate-solubilizing ability of Paenibacillus spp. has been reported by Hu et al. (Citation2006) and Vazquez et al. (Citation2000). RP utilization was greater in the PM treatment. These results suggest that the newly appeared bands in the PM treatment might be related to available P of RP-enriched soil. The bands related to the genus Chitinophaga might be incorporated from amendment with sewage sludge compost, because the DGGE profile of the sewage sludge compost also showed the bands that were identified as Chitinophaga in the SS treatment.
Soil incubation results show that the dominant isolate of PSB in the soil with or without compost amendments was closely related to B. fungorum. Phosphate solubilization ability of B. fungorum was reported by Mailloux et al. (Citation2009), who found that apatite was solubilized by B. fungorum. A few colonies that deviated from the dominant isolate in the PM, SS, or PSD treatments were also closely related to Burkholderia spp. Burkholderia is one of the genera that has many species reported to be able to solubilize inorganic phosphate compounds such as tricalcium phosphate or rock phosphate (Rodriguez and Fraga Citation1999). Purnomo et al. (Citation2005) reported that a Burkholderia spp. is a common P-solubilising bacterial species in the rhizosphere of different rice varieties.
The present work showed that RP enrichment of soil enhanced plant P uptake. Plant utilization of RP was further enhanced by composts amendment of soil. Compost amendments significantly enhanced biological properties of the soil by increasing microbial biomass P and population density of PSB, which showed significant positive correlation with the available P in soil. There is a significant interaction between the compost treatments and the RP treatments on the available P in soil ().
The differences of DGGE profile in some compost amendments with and without RP enrichment suggest that there is an interaction between the available P of RP-enriched soil and soil microbial community altered by compost treatments, especially with PM amendment. The enhanced utilization rate of RP showed by compost treatment could be related to B. phytofirmans in PM treatment, B. caledonica and A. ramosus in SS treatment and B. phenazinium in PSD treatment, which were identified as specific isolates of PSB in each compost treatments.
In conclusion, the increased available P in soil by combined use of RP with compost is strongly related to the enhanced microbial biomass P and population density of PSB of soil, which is dominated by Burkholderia spp. The enhanced utilization rate of RP showed by compost treatment could be related to specific isolates of PSB in each compost treatment.
Acknowledgments
The authors would like to acknowledge the financial support of the Ministry of Education, Sports, Culture, Science and Technology, Japan.
References
- Altschul, SF , Gish, W , Miller, W , Myers, EW , and Lipman, DJ , 1990. Basic local alignment search tool , J. Mol. Biol. 215 (1990), pp. 403–410.
- Ayaga, G , Todd, A , and Brookes, PC , 2006. Enhanced biological cycling of phosphorus increases its availability to crops in low-input sub-Saharan farming systems , Soil Biol. Biochem. 38 (2006), pp. 81–90.
- Bolland, MDA , and Barrow, NJ , 1988. Effect of level of application on the relative effectiveness of rock phosphate , Fertil. Res. 15 (1988), pp. 181–192.
- Braithwaite, AC , Eaton, AC , and Groom, PS , 1989. Some factors associated with the use of the extractants 2% citric acid and 2% formic acid as estimator of available phosphorus in fertilizer products , Fertil. Res. 19 (1989), pp. 175–181.
- Bremner, JM , and Shaw, K , 1958. Denitrification in soil. I. Methods of investigation , J. Agric. Sci. Camb. 51 (1958), pp. 22–39.
- Brookes, PC , Powlson, DS , and Jenkinson, DS , 1982. Measurement of microbial biomass phosphorous in soil , Soil Biol. Biochem. 14 (1982), pp. 319–329.
- Chen, YP , Rekha, PD , Arun, AB , Shen, FT , Lai, WA , and Young, CC , 2006. Phosphate solubilizing bacteria from subtropical soils and their tricalcium solubilizing abilities , Appl. Soil Ecol. 34 (2006), pp. 33–41.
- Chowdhury, MAH , Kouno, K , and Ando, T , 1999. Correlation among microbial biomass S, soil properties, and other biomass nutrients , Soil Sci. Plant Nutr. 45 (1999), pp. 175–186.
- Classification Committee of Cultivated Soils 1996: Terrestrial Regosols. In Classification of Cultivated Soils in Japan: Third approximation, Ed. Hamazaki T, pp. 10–11. National Institute for Agro-Environmental Science, Tsukuba, Japan.
- Dilly, O , Bloem, J , Vos, A , and Munch, JC , 2004. Bacterial diversity in agricultural soils during litter decomposition , Appl. Environ. Microbiol. 70 (2004), pp. 468–474.
- Egashira, K , Tsuda, S , and Takuma, K , 1985. Relation between soil properties and the erodibility of Masa soils (Granitic soils) , Soil Sci. Plant Nutr. 31 (1985), pp. 105–111.
- Haynes, RJ , and Mokolobate, MS , 2001. Amelioration of Al toxicity and P deficiency in acid soils by addition of organic residues: a critical review of the phenomenon and mechanisms involved , Nutr. Cycl. Agroecosys. 59 (2001), pp. 47–63.
- Hellal, FA , El-Hady, MA , and Ragab, AAM , 2009. Influence of organic amendments on nutrient availability and uptake by faba bean plants fertilized by rock phosphate and feldspar , Am.-Eurasian J. Sustain. Agric. 6 (2009), pp. 271–279.
- Herai, Y , Kouno, K , Hashimoto, M , and Nagaoka, T , 2006. Relationships between microbial biomass nitrogen, nitrate leaching and nitrogen uptake by corn in a compost and chemical fertilizer amended regosol , Soil Sci. Plant Nutr. 52 (2006), pp. 186–194.
- Heuer, H , Krsek, M , Baker, P , Smalla, K , and Wellington, EMH , 1997. Analysis of Actinomycete communities by specific amplification of genes encoding 16S rRNA and Gel-Electophoretic separation in denaturing gradients , Appl. Environ. Microbiol. 63 (1997), pp. 3233–3241.
- Hirata, H , Watanabe, K , Fukushima, K , Aoki, M , Imamura, R , and Takahashi, M , 1999. Effect of continues application of farm yard manure and inorganic fertilizer for 9 years on changes in phosphorus compounds in plow layer of an upland Andosol , Soil Sci. Plant Nutr. 45 (1999), pp. 577–590.
- Hoberg, E , Marschner, P , and Lieberei, R , 2005. Organic acid exudation and pH changes by Gordonia sp. and Pseudomonas fluorescens grown with P adsorbed to goethite , Microbiol, Res. 160 (2005), pp. 177–187.
- Hu, X , Chen, J , and Guo, J , 2006. Two phosphate- and potassium-solubilizing bacteria isolated from Tianmu Mountain, Zhejiang, China , World J. Microb. Biot. 22 (2006), pp. 983–990.
- IUSS Working Group WRB 2006., , World Reference Base for Soil Resources 2006. World Soil Resources Reports 103, FAO, Rome.
- Iyamuremye, F , Dick, RP , and Baham, J , 1996. Organic amendments and phosphorus dynamics: III. Phosphorus speciation , Soil Sci. 161 (1996), pp. 444–451.
- Jorquera, MA , Hernandez, MT , Rengel, Z , Marschner, P , and Mora, ML , 2008. Isolation of culturable phosphobacteria with both phytate-mineralization and phosphate-solubilization activity from the rhizosphere of plants grown in a volcanic soil , Biol. Fertil. Soils 44 (2008), pp. 1025–1034.
- Khan, KS , and Joergensen, RG , 2009. Changes in microbial biomass and P fractions in biogenic household waste compost amended with inorganic P fertilizers , Soil Biol. Biochem. 100 (2009), pp. 303–309.
- Khan, MS , Zaidi, A , and Wani, PA , 2006. Role of phosphate solubilizing microorganisms in sustainable agriculture – A review , Agron. Sustain. 26 (2006), pp. 1–15.
- Kleinman, P , Sullivan, D , Wolf, A , et al., 2007. Selection of water extractable phosphorus test for manures and biosolids as an indicator of runoff loss potential. Technical report: waste management , J. Environ. Qual. 36 (2007), pp. 1357–1367.
- Kouno, K , Wu, J , and Brookes, PC , 2002. Turnover of biomass C and P in soil following incorporation of glucose and ryegrass , Soil Biol. Biochem. 34 (2002), pp. 617–622.
- Kwabiah, AB , Palm, CA , Stoskopf, NC , and Voroney, RP , 2003. Response of soil microbial biomass dynamics to quality of plant materials with emphasis on P availability , Soil Biol. Biochem. 35 (2003), pp. 207–216.
- Lukito, HP , Kouno, K , and Ando, T , 1997. Microbial biomass in soils as influenced by different nutrients , J. Fac. Appl. Biol. Sci. 36 (1997), pp. 131–137.
- Lukito, HP , Kouno, K , and Ando, T , 1998. Phosphorus requirements of microbial biomass in a Regosol and an Andosol , Soil Biol. Biochem. 30 (1998), pp. 865–872.
- Maidak, BL , Larsen, N , McCaughey, J , Overbeek, R , Olsen, GJ , Fogel, K , Blandy, J , and Woese, CR , 1994. The ribosomal database project , Nucleic Acids Res. 22 (1994), pp. 3485–3487.
- Mailloux, BJ , Alexandrova, E , Keimowitz, AR , et al., 2009. Microbial mineral weathering for nutrient acquisition releases Arsenic , Appl. Environ. Microbiol. 75 (2009), pp. 2558–2565.
- Mengel, K , 1997. Agronomic measures for better utilization of soil and fertilizer phosphates , Eur. J. Agron. 7 (1997), pp. 221–233.
- Murphy, J , and Riley, JP , 1962. A modified single solution method for the determination of phosphate in natural waters , Anal. Chim. Acta. 27 (1962), pp. 31–36.
- Muyzer, G , Waal, EC , and Uitterlinden, AG , 1993. Profiling of complex microbial populations by denaturing gradient gel electrophoresis analysis of polymerase chain reaction-amplified genes coding for 16S rRNA , Appl. Environ. Microbiol. 59 (1993), pp. 695–700.
- Nautiyal, CS , 1999. An efficient microbial growth medium for screening phosphate solubilizing microorganisms , FEMS Microbiol. Lett. 170 (1999), pp. 265–270.
- Nishio, M , , 1996: Microbial fertilizers in Japan. FFTC-Extension Bulletins 1–12. National Institute of Agro-Environmental Science, Ibaraki, Japan.
- Nishio, M , and Kusano, S , 1980. Fluctuation patterns of microbial numbers in soil applied with compost , Soil Sci. Plant Nutr. 26 (1980), pp. 581–593.
- Olsen, SR , Cole, CV , Watanabe, FS , and Dean, LA , 1954. Estimation of available phosphorus in soils by extraction with sodium bicarbonate. USDA Circulation No. 939 . Washington: Government Printing Office; 1954.
- Peix, A , Rivas, R , Santa-Regina, I , Mateos, PF , Martinez-Molina, E , Rodriguez-Barrueco, C , and Velazquz, E , 2004. Pseudomonas lutea sp. nov., a novel phosphate-solubilizing bacterium isolated from the rhizosphere of grasses , Int. J. Syst. Evol. Microbiol. 54 (2004), pp. 847–850.
- Purnomo, E , Mursyid, A , Syarwani, M , Jumberi, A , Hashidoko, Y , Hasegawa, T , Honma, S , and Osaki, M , 2005. Phosphorus solubilizing microorganisms in the rhizosphere of local rice varieties grown without fertilizer on acid sulfate soils , Soil Sci. Plant Nutr. 51 (2005), pp. 679–681.
- Reyes, I , Bernier, L , Simard, RR , and Antoun, H , 1999. Effect of nitrogen source on the solubilization of different inorganic phosphates by an isolate of Penicillium rugulosum and two UV-induced mutants, FEMS Microb , Ecol. 28 (1999), pp. 281–290.
- Rodriguez, H , and Fraga, R , 1999. Phosphate solubilizing bacteria and their role in plant growth promotion , Biotech. Adv. 17 (1999), pp. 319–339.
- Saison, C , Degrange, V , Oliver, R , Millard, P , Commeaux, C , Montange, D , and Roux, XL , 2006. Alteration and resilience of the soil microbial community following compost amendment: Effects of compost level and compost-borne microbial community , Environ. Microbiol. 8 (2006), pp. 247–257.
- Sekiya, K , 1970. "Phosphoric acid (in Japanese)". In: S, Ishizawa , ed. Analysis methods for measuring soil fertility . Tokyo: Yokendo co. Ltd.; 1970. pp. 251–253.
- Vazquez, P , Holguin, G , Puente, ME , Lopez-Cortes, A , and Bashan, Y , 2000. Phosphate solubilizing microorganisms associated with the rhizosphere of mangroves in a semiarid coastal lagoon , Biol. Fertil. Soils 30 (2000), pp. 460–468.
- Wickramatilake, ARP , Kouno, K , and Nagaoka, T , 2010. Compost amendment enhances the biological properties of Andosols and improves phosphorus utilization from added rock phosphate , Soil Sci. Plant Nutr. 56 (2010), pp. 607–616.
- Wu, J , Huang, M , Xiao, HA , Su, YR , Tong, CL , Huang, DY , and Syers, JK , 2007. Dynamics in microbial immobilization and transformation of phosphorus in highly weathered subtropical soil following organic amendments , Plant Soil 290 (2007), pp. 333–342.
- Zou, X , Binkley, D , and Doxtader, KG , 1992. A new method for estimating gross phosphorus mineralization and immobilization rates of soil , Plant Soil 147 (1992), pp. 243–250.