Abstract
The System of Rice Intensification (SRI) is based on a set of practices such as transplanting young seedlings and aerobic soil management during the vegetative stage without using costly external input, which attracted rice researchers because of higher yields compared with conventional flooded practices. This review assesses some field data for SRI performance in light of current knowledge about certain agronomic practices, focusing particularly on nitrate production in aerobic paddy soil and the possible effect of differences in nitrate uptake on rice growth, as this could help explain some part of rice performance with SRI. Aerobic soil environments realized with SRI management might be favorable for nitrification as well as for the expansion of rhizosphere area, which could enhance nitrate uptake and boost the yield potential of rice. The effect of nitrate uptake and assimilation under SRI practice on dry matter production should be studied comprehensively to explore the possibility of breaking the yield ceiling of rice.
Introduction
There is no question about the need to increase the yield potential of rice in response to global population increases and arable land decreases. One of the approaches to meeting this goal is breeding new varieties. Many high-yielding varieties have been released, and the introduction of novel genes has made significant progress (Matsuoka et al. Citation2001; Surridge Citation2002), yet the practical impacts are limited. Some gains have come from a crop management approach, with site-specific crop management (SSCM) being introduced to attain better yields with reduced by optimized fertilizer input (Dobermann et al. Citation2002). Dealing with water scarcity is also a challenge for the future in rice-growing regions. Alternate wetting and drying (AWD) rice cultivation and aerobic rice cultivation have been developed to address this issue (Bouman et al. Citation2007). However, yields of rice under such management have remained mostly unchanged or decreased slightly. Development of innovative management technologies that will boost rice yield as well as increase water productivity, in particular reducing water requirements, remains to be achieved.
To elucidate the conditions that must be satisfied to raise rice yields up to their potential is an important research goal. For example, Horie et al. (Citation2005) suggested – based on their review of past scientific knowledge and their research data (Takai et al. Citation2006) – that a higher crop growth rate (CGR) at the late reproductive stage (2 weeks before heading) would be the most effective change for realizing higher yield. This concept implies that a high CGR can sustain an increase in spikelet numbers by controlling their degeneration together with high dry matter production which will contribute to boosting yield potential. However, research on crop management systems to achieve high yields closer to the yield potential in a stable manner is not sufficient. This is one reason why grass-root techniques such as System of Rice Intensification (SRI) have attracted researchers’ attention.
SRI was originally developed in the 1980s in Madagascar by Father Henri de Laulanié, who had a background in agronomy, working in close collaboration with farmers (Surridge, Citation2004). SRI consists of several management components applied in an integrated manner; namely, transplanting single young seedlings carefully in a square pattern with wide spacing, weeding by mechanical weeder from the early vegetative stage, and alternate wetting and drying (AWD) water management during the vegetative stage followed by shallow submergence after the panicle initiation (PI) stage (Uphoff and Randriamiharisoa Citation2002; Uphoff et al. Citation2002; Satyanarayana et al. Citation2007).
SRI drew attention in the 2000s when earlier reports showed extremely high yields such as 15 t ha−1, as well as when controversy flared between proponents of SRI and skeptics among some leading rice researchers (Stoop et al. Citation2002; Uphoff and Randriamharisona Citation2002; Dobermann Citation2004; Sheehy et al. Citation2004; Sinclair and Cassman Citation2004; Stoop and Kassam Citation2005; McDonald et al. Citation2006, Citation2008; Uphoff and Kassam Citation2008), some of which was reported as commentary in a major scientific journal, Nature (Surridge Citation2004). However, the principle of the high yield of SRI has not been fully elucidated scientifically, and this is why SRI remains a good subject of study for researchers.
Despite the dispute within the academic community, SRI has been disseminated to farmers in more than 40 countries, most in South and Southeast Asia. Although the exact area of adoption has not been officially reported, there is an estimate that SRI has been adopted in 750,000 ha in India, and 17,000 ha in Indonesia (Uphoff and Kassam Citation2009). A compilation of results from 11 surveys in 8 countries, including 16,000 SRI farmers, has shown, on average, a 47% yield increase, 40% water savings, 23% lower production costs, and 68% increase in farmer income, compared to conventional rice cultivation (Sato and Uphoff Citation2007; Africare et al. Citation2010).
Growth of Rice Plant Under Sri Management
The management practices of system of rice intensification (SRI) include the following points: (1) the age of seedlings at transplantation is less than 15 days (two-to-three-leaf stage); (2) seedlings are transplanted with wide spacing, and one seedling per hill; (3) water management is undertaken to maintain paddy soils in mostly aerobic condition, e.g., by small daily applications of water with no flooding, AWD, shallow water management from PI to harvest, and also active soil aeration through mechanical weeding, and application of organic matter for improving soil structure and function as well as nutrient availability (Dobermann Citation2004; Tsujimoto et al. Citation2009; Thakur Citation2010; Thakur et al. Citation2010b). Although these practices might affect the synergistic effects on the growth and yield of the rice plant, each factor should be verified by scientific methods. Yet, little information is available to evaluate the effect of single factors on the growth performance and/or yield of the rice plant.
Young seedlings
Young seedlings used in SRI can be classified as “nursling seedlings”, which are defined as seedlings at the two-to-three leaf age including incomplete leaves (Sasaki and Hoshikawa Citation1997a; Sasaki Citation2004). There are developmental changes in terms of energy dependence at this stage of plant growth, moving from reliance on nutrients from the endosperm to benefiting from photosynthesis at the leaf age of 2.4 (Sasaki and Hoshikawa Citation1997a; Sasaki Citation2004), when one-quarter of the endosperm nutrients still remain in nursling seedlings at that leaf age. This could be a basis for rapid rooting and development after transplanting. This ability of rooting and development was shown to be attributable to the crown roots from the first and coleoptile nodes (Sasaki and Hoskikawa Citation1997b; Sasaki Citation2004). They also reported an advantage of nursling seedlings of leaf age 2.3 to 2.5 in terms of tolerance of submergence () (Sasaki Citation2000, Citation2004). These reports suggest that transplanting nursling seedlings could help them develop rapidly and hence the tillering begins from the lower nodes compared to transplanted older seedlings, which brings out the tillering potential of rice plant more fully (Nemoto et al. Citation1995). A shallow depth for transplanting nursling seedlings could also be favorable for more tillering. Also, biological potential of rice seedlings and their improvement in an oxic nursery environment are suggested for young seedlings (12d old) even they are transplanted to flooded paddy field (Mishra and Salokhe Citation2008).
Figure 1. Diagrammatic representations of the developmental changes in rice nursling seedlings and the ideal plant ages of nursling seedlings for transplantation (Sasaki Citation2004).
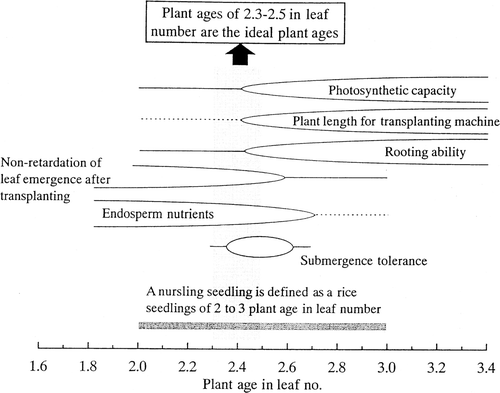
Plant spacing
Plant spacing or plant density is one of the key techniques of SRI as mentioned before. Nguu and De Datta (Citation1979) reported for conventional rice cultivation that a relatively short-statured variety with moderate tillering capacity must have close spacing for maximum yield; i.e., plant spacing of 15 × 15 cm gives significantly greater yields than 20 × 20 and 25 × 25 cm. Thakur et al. (Citation2010a) reported that SRI practices through optimum spacing attempt to minimize competition among rice plants for the various growth factors. Although chlorophyll content of the flag leaves and third leaves decreased with ripening, the rate of decrease is different among planting densities, and chlorophyll content was higher with wider spacing (30 by 30) compared to narrow spacing (20 by 20) (Mishra and Salokhe Citation2010). This high chlorophyll content with wider spacing was attributed to higher root-oxidizing activity of more widely-spaced rice plants (Mishra and Salokhe Citation2010). The SPAD value of the flag leaf of hybrid rice with lower plant density grown under SRI management was higher than with higher plant density at the flowering stage (Lin et al. Citation2009). Plants grown under wider spacing had a higher photosynthetic rate in their leaves than did those with closer spacing regardless of water management during the ripening stage of the rice plants (Thakur et al. Citation2010a). These reports indicated that wider spacing was more profitable for producing carbohydrate by rice in the later growth stages of rice plants. SPAD value, chlorophyll content or photosynthetic rate will reflect the intensity factor of production of carbohydrate.
Total amount of carbohydrate produced by rice plants per unit area is regulated by the intensity factor, the capacity factor (leaf area per unit area), and light intensity inside of the canopy (canopy structure). Leaf area index (LAI) of rice plants planted at 13.5 plants m−2 was the highest among other plant densities evaluated (7.5 or 19.5 plants m−2) at 20 days after heading (Lin et al. Citation2005). Although the largest leaf area per hill was found with wide plant spacing (30 by 30 cm) at flowering (Thakur et al. Citation2010a), medium plant spacing (20 by 20 cm) recorded the highest LAI among treatments at 20 days after heading (Lin et al. Citation2009; Thakur et al. Citation2010a). The wider spacing between plants under SRI management leads to a more prolonged, open crop canopy (Stoop Citation2005). Although carbohydrate production by rice plants with wide spacing during the ripening stage was high, no difference in total biomass production per unit area (dry matter yield of above ground) among spacings was observed under SRI (Stoop Citation2005). Rice yield under SRI management with different plant spacings was evaluated in the Philippines (Miyazato et al. Citation2010). In some cases, the highest rice yield was obtained with even wider spacing (40 by 40 cm) among the field trials; however, plants with middle spacing (25 by 25 cm) gave higher yields than those with wider spacing (35 by 35 cm) at Hernanie Domingo, Philippines (Miyazato et al. Citation2010). These reports indicated that suitable plant spacing does not exist among fields a priori even though grown under SRI management. It may be different according to the soil fertility level.
Water management
One of the basic changes in management that Fr. Laulanié introduced was to move from flooded fields to AWD management, because he realized that the growth of rice was superior in paddy soils that were mostly aerobic (Surridge Citation2004). There are actually several water management practices that can achieve this objective, i.e., (1) intermittent irrigation before PI, and shallow water management from PI to maturity (Thakur et al. Citation2010a); (2) shallow water depth (10–20 mm) from transplanting to early tillering stage, and 60–80% of the saturated moisture content (SMC) in tillering stage, then shallow water depth (10–30 mm) from elongation to flowering, 80% of SMC during milk ripening stage, and dry field from yellow ripening stage (Lin et al. Citation2005); (3) AWD throughout the crop cycle (Satyanarayana et al. Citation2007); and so on. The main purposes of those variants in water management are water saving and improving root development (Zhang et al. Citation2009).
In some experiments, there was no difference in the yield of rice between conventional and SRI irrigation, and in some reports, rice yield under SRI management was higher than with conventional practices, but most references reported that total water used in conventional irrigation was higher than SRI management. For example, total water used in conventional practices was 2 times higher than modified SRI irrigation in India (Satyanarayana et al. Citation2007) and 1.4 times higher in Japan (Chapagain and Yamaji Citation2010). Consequently, water productivity in SRI irrigation was higher than in conventional irrigation. Satyanarayana et al. (Citation2007) summarized the previous reports about water savings of SRI and concluded that SRI practice in different environments could save substantial water, accompanied by significant gains in rice production and profitability.
Root length density of rice plants grown under SRI (intermittent flooding) was higher than under continuously flooded management, especially in the middle and late ripening growth stages of the rice plants (Mishra and Salokhe Citation2010). Significantly higher root dry weight per hill and per unit area with SRI compared to recommended management practices (RMP; kept flooded to maintain a ponded water of 5–8 cm depth during the entire vegetative stage of rice plant) was observed during the early ripening stage of the rice plants. In addition, the amount of xylem exudates and the exudation rate per hill were significantly higher in SRI than RMP (Thakur et al. Citation2010a,Citationb). Those reports indicate that root biomass and the viability of roots were increased by SRI practices compared to the conventional irrigation method.
What kind of effects will be brought about by drainage during the vegetative growth stage? Drainage produces an oxidative environment in the soil layer which suppresses the production of toxic substances in the rhizosphere of rice, which may otherwise be produced in reduced conditions. The oxic environment thus prepared will enhance the nitrification of ammonium in plow-layer soil. Greater root dry weight and deeper rooting is another important effect of drainage as mentioned above (Thakur et al. Citation2010a,Citationb). As the rhizosphere of the rice plant is a favorable place for ammonium-oxidizing bacteria (AOB) (Briones et al. Citation2003), the expansion of the rhizosphere volume will benefit rice plants to take up more N, not only as ammonium but also as nitrate, which may be produced in the rhizosphere and may contribute as a signal to enhance ammonium uptake (Zhao et al. Citation2008) as well as a possible N reserve (Bloom Citation1997) for dry matter production in the reproductive growth stage. This working hypothesis will be explained later.
Nitrogen application rate
Few reports were available about the effect of N application rate on the growth and yield of rice under SRI (Lin et al. Citation2009; Zhao et al. Citation2009), because N fertilizer was not widely referred to SRI practices (Thakur et al. Citation2010b) and more organic matter was commonly applied to SRI fields than to conventional practice fields (e.g., Tsujimoto et al. Citation2009). The application of N at the rate of greater than 180 kg ha−1 (which included compost as an N source) recorded a maximum yield of 9.5 t ha−1 for hybrid rice grown under SRI, while the yield of hybrid rice grown conventionally with the same N dose by chemical N fertilizer, was 7.7 t ha−1 and yield increased with more N (Lin et al. Citation2009). Optimum application rate of fertilizer N should be investigated under SRI management condition with inbred rice.
Weeding and soil churning
Weeding is imperative for SRI farmers, because keeping fields mostly unflooded is an essential part of SRI management, and this is conducive to greater weed growth. As weeding is laborious, farmers usually use a mechanical weeder (rotary hoe) which plows weeds into the soil. During this weeding work, soil churning is carried out simultaneously. It is reported that weeding by mechanical weeder raised the yield by 0.5–1.0 tons ha−1 per weeding (Satyanarayana et al. Citation2007). The effect of churning soil could be understood as aeration into the soil. In addition, the effect of returning the soil surface to its initial condition might be beneficial for biological nitrogen fixation by algae which proliferate on the puddled soil surface (Roger and Kulasooriya Citation1980). Kondo and Yasuda (Citation2003) reported that the amount of biologically fixed nitrogen would be 1.6–2.0 g m−2 in one cropping season for paddy fields in Japan under conventional management. Moreover, total N in the soil surface layer significantly increased compared with conventional water treatment when an early drained condition was introduced to a clayey paddy field, and algae proliferation was suggested on such a drained moist soil surface (Kobayashi et al. Citation2000). Therefore, early drainage plus soil churning would be beneficial not only for weeding but also for increasing the organic nitrogen input by N2 fixation via algae.
As mentioned above, SRI is an integrated management system and it is not easy to show the effects of individual practices on yield. However, it is important to elucidate the individual contributions to the increase in N uptake and dry matter production as well as to know the synergistic effect of these individual components.
Yield of Rice Under Sri Compared To Conventional Practices
Yields in farmers’ fields
Without comparison to conventional cultural practices on the same soils, the grain yield of SRI was reported to be in the range of 15.0 to 23.4 t ha−1 in famers’ fields in the highland areas of Madagascar (Rafaralahy Citation2002). These yields were considerably higher than the maximum yield estimated to apply to rice plants in the tropics (growth duration was 110 days) and in cooler environments (growth duration 150–160 days), which were 12.5 t ha−1 and 18 t ha−1, respectively, according to calculations of photosynthesis and radiation use efficiency of C3 rice (Sheehy Citation2000). Fernandes and Uphoff (Citation2002) summarized SRI reports from 17 countries and found that SRI yields averaged 6.8 t ha−1, while that from conventional cultural practices (recommended conventional irrigated rice management) was 3.9 t ha−1. On-farm trials were conducted in India with all kinds of soils and irrigation methods, 19 cultivar varieties and different climate conditions. Results are shown in (Satyanarayana et al. Citation2007). This table showed that a higher yield was obtained under SRI than under farmers’ present practices. Although the reports did not describe experimental details, they suggested that drier upland Rayalseema districts gave the best response to SRI methods rather than the low-lying coastal districts where soils are more saturated. Tsujimoto et al. (Citation2009) investigated the factors for high yield (7.0–9.9 t ha−1) of an SRI farmer's plot by comparing it with the conventional farmers’ plot (2.6–5.0 t ha−1). Average plowing depth of SRI and conventional management fields was 28.8 cm and 12.5 cm, respectively. The average amount of mineralizable N was 116.8 mg kg−1 at the SRI field, while it was 65.6 mg kg−1 at the conventional field. They concluded that soil fertility, represented by the soil available N, was the main factor for the higher yield (Tsujimoto et al. Citation2009).
Table 1. Summary of results of on-farm comparison trials, supervised by ANGRAU extension staff, by region, kharif (summer) season, 2003 (Satyanarayana et al. Citation2007)
Yields in experimental fields
SRI plots produced significantly larger grain yields than recommended practices in the experimental field (Thakur et al. Citation2010a,Citationb). Plant spacing affected the yield regardless of cultural practices; i.e., SRI and recommended practice. The highest yield was found at 20 by 20 cm spacing regardless of water management, and the yield of SRI (628 g m−2) was higher than that of conventional practice (448 g m−2) with 20 by 20 cm plant spacing (Thakur et al. Citation2010a). Lin et al. (Citation2005) reported no significant difference in the yield of rice grown under shallow water with wetting and drying (SWD) among plant density of 7.5, 13.5 and 19.5 plants m−2. In addition, lower yields of rice plants (with plant density of 19.5 plants m−2) grown under conventional water management than under SWD with same plant density were observed.
Wang et al. (Citation2002) reported that responses of normal indica rice and hybrid rice to SRI were different. The yield of the normal indica rice variety grown under the SRI method, but not all SRI practices, was distinctively lower than when grown under conventional practices, but the yield of the hybrid rice variety grown under SRI methods was close to that grown under conventional practices. This fact was attributed to the strong tillering ability and vegetative growth advantage of the hybrid rice variety (Wang et al. Citation2002). Stoop (Citation2005) pointed out that intermediate and late-maturing types were generally more responsive to SRI than the early types because of the increased tillering and rooting of the former.
On the other hand, SWD with N fertilizer (application rate was 180 kg ha−1) had enhanced sink size and grain yield of rice plants compared to conventional water management (Lin et al. Citation2005). SRI was based on a set of practices to manage plants, soil, water and nutrients, and increased yields of rice grown under SRI were found only when several SRI components were used in good combination (Chapagain and Yamaji Citation2010). They conducted the combination experiment and the highest yield (8.1 t ha−1) was obtained in the treatment with younger seedlings (14 days old) transplanted singly at wider spacing (30 by 30 cm) under flooded conditions, followed by normal seedlings (21 days with 4 seedling hill−1) transplanted at wider spacing. The lower yield in the SRI plot may be attributed to fewer tillers per area, which might be an unfavorable condition for tillering under intermittent conditions in temperate areas of Japan, where low temperatures were recognized during the transplanting season. WARDA conducted a series of exploratory field experiments to verify the potential possible adaptation of SRI to West African conditions. The results showed grain yields of rice grown under irrigated conditions were higher than those grown under SRI (Stoop Citation2005). Those reports indicated that it was difficult to say which cultural practices, SRI or conventional, were beneficial to get high yields of rice plants, even though a scientific experiment was conducted. The relations between experimental conditions and results should be reviewed in detail under some hypothesis to elucidate these discrepancies.
Relationship Between N Uptake and Biomass and Yield of Rice Plant
N uptake and spacing
Nitrogen is one of the major nutrients for crops, and generally speaking, the yield of rice was regulated by the N uptake pattern and the amount of N absorbed by the rice plant. However, few reports were available to identify the N use efficiency or N dynamics in paddy fields under SRI conditions. Plant density affects the N dynamics in paddy fields. The amount of exchangeable ammonium N disappeared earlier with narrow spacing than with wider spacing when basal N fertilizer was applied (Takahashi et al. Citation1973). A greater amount of N was absorbed by rice plants grown under narrow spacing than wider spacing during the early tillering stage of rice plants; during the middle and late growth stages of rice plants a lesser amount of N was absorbed in narrow spacing than wider spacing under conventional cultural practices (Takahashi et al. Citation1973). In addition, no significant difference in recovery rate of basal N and amount of N in rice plants at harvest between narrow and wide spacing was seen (Takahashi et al. Citation1973). These findings indicated that plant spacing could contribute to the N uptake pattern but could not regulate the amount of absorbed N in rice plants until harvest.
N uptake and depth of water management, depth of plow layer
A field experiment was conducted at Yamagata University in 2009 (general cultural practices; application rate of compost: 5 t ha−1, application rate of basal fertilizer: 45 kgN ha−1, 5 seedlings (21 days old) hill−1, treatment: spacing (wider spacing: 30 by 30 cm, narrow spacing: 15 by 30 cm) and water management (shallow water management: 1–2 cm of flooded water, conventional water management: 5–7 cm of flooded water)). Earlier disappearance of ammonium was observed under narrow spacing, the same as in conventional water management. However, a higher amount of exchangeable ammonium N in the soil was observed in shallow water management than in conventional water management. Comparison between plant spacings revealed quick N uptake by rice plants in narrow spacing. The total amount of N in rice plants at harvest between narrow and wide spacing was almost the same under shallow water management, but a lesser amount of N was found under conventional water management with wide spacing (data not shown). As this was a preliminary experiment with no replications and was conducted for just one year, we could not conclude the fate of N in paddy fields under shallow water management, but it might be said that the dynamics of N were not affected by shallow vs. deep water management.
On the other hand, there was a significant difference in the amount of N in rice plants between SRI and traditional flooding (TF) plots. At a lower N rate (less than 80 kg N ha−1), rice in SRI take up more N than those in TF; however, at a higher N rate (more than 160 kgN ha−1), N uptake was higher in TF, though yields in SRI were always higher than in TF. (Zhao et al. Citation2009). The SRI fields in Madagascar have been continuously plowed to a depth of 25 to 30 cm by double-digging since the adoption of organic matter application in the early 1990s, while conventional fields were plowed 10 to 20 cm (Tsujimoto et al. Citation2009). The depth of the plowed layer was not reported in other references, but the management practices of SRI often include application of compost or other organic amendments (Thakur et al. Citation2010b). Tsujimoto et al. (Citation2009) reported that average mineralizable N (at depths of 0–30 cm) was linearly related to rice grain yield irrespective of management practices. The winners of rice-yield contests in Japan in the 1950 s and 1960 s also applied organic matter combined with deep plowing (Tsuno Citation1991). Those reports indicated that to obtain higher yields of rice plants soil N fertility was crucially important.
N uptake and biomass production or grain yield
Generally, a linear relationship between the amount of N in rice plants and yield under conventional practices was recognized in a wide range of brown rice yields from 3 t ha−1 to 9 t ha−1 in Japan (Ando Citation2006). The relationship between amounts of N in rice plants at harvest and grain yields is shown in (data from Lin et al. Citation2009). There was a linear relationship between the two parameters, regardless of whether SRI or conventional practices were used, in the various plant density levels. This data suggests that there is no specific effect on NUE by SRI and the higher N uptake might be the result of using manure in the SRI plot. Also, there was no significant difference in the relationship between yield and amount of N in rice plants grown under SRI and conventional practices in the experiment conducted in Yamagata University (data not shown). The harvest index (HI) of rice plants under SRI (0.44–0.51) was higher than under traditional flooding (0.38–0.45) (Zhao et al. Citation2009). Thakur et al. (Citation2010b) reported that biomass production of SRI was the same as that with RMP, but the grain yield of SRI was significantly higher than RMP. This difference was attributed to the difference in HI. HI of SRI (0.47) was significantly higher than that of RMP (0.33). Normally, HI of high-yield varieties grown in irrigated fields range between 0.4 and 0.5 (Chang and Li Citation1991). Therefore, the HIs of Zhao et al. (Citation2009) and Thakur et al. (Citation2010b) obtained in conventionally flooded practices were a little bit lower than the normal value. There is no consistent result for biomass production and HI compared between SRI and conventional practices. The reason for these discrepancies should be studied.
Figure 2. Relationship between N uptake at harvest and grain yield of rice cultivated by SRI (New rice management) and Control (Standard rice management). (Graphed from the data of Table 2 and Table 3 of Lin et al. Citation2009.) Treatment: Total fertilizer nitrogen applied for both SRI and control plots was 120,150,180,210 kgN ha−1. In SRI management, basal applied N was rapeseed compost and urea was applied as topdressing, 5 days and 40 days after transplanting. In control, basal applied N was urea with the same amount of nitrogen as SRI plot and topdressings were same as SRI. Transplanting density was 15, 18, and 21 plants per m2 as shown in parentheses.
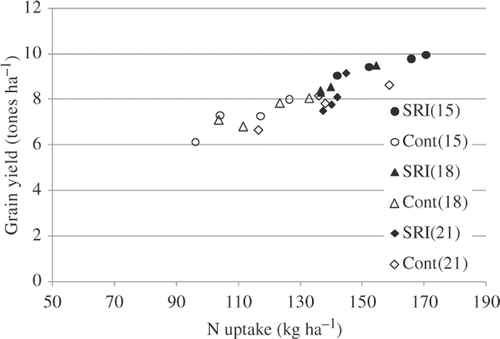
Effect of non-flooded soil environment on growth of rice
Generally, paddy fields are submerged and develop an oxidized surface soil layer and a reduced plow soil layer (Patrick and Reddy Citation1976; Hasebe et al. Citation1987). Lowland rice has evolved to adapt to a submerged anoxic soil environment by developing aerenchyma in the plant roots through which air diffuses into the soil and makes the vicinity of the root into an oxic environment (Jackson and Armstrong Citation1999). Behavior of inorganic nitrogen in paddy soils can be categorized in terms of two reactions: one is in a reduced soil environment, where soil organic nitrogen is mineralized to NH4 and adsorbed by cation exchange sites such as clay. On the other hand, at oxidized sites, nitrification by ammonium-oxidizing bacteria (AOB) and nitrite-oxidizing bacteria could proliferate if a substantial amount of NH4 is supplied (Patrick and Reddy Citation1976). The rhizosphere is a favorable site for nitrification (Kirk Citation2003), and model simulation has shown that NO3 accounts for 15 to nearly 40% of N uptake by rice plants even under submerged conditions (Kirk and Kronzucker Citation2005). So, what will happen when such submerged soil becomes drained and aerated? The soil conditions could be more favorable for nitrification, and it must be a key to understanding the role of water management in SRI.
Effect of drainage on rice
Midseason drainage and enhancement of percolation of flooded water together with deep plowing with organic matter application are indispensable practices for farmers who achieved 8 to 10 t ha−1 of yield in rice-yield contests which were held from 1948 to 1968 (Honya Citation1989). Under the conditions of high N application rate, Tanaka (Citation1970) examined the effect of intermittent irrigation during the vegetative stage followed by submergence after panicle initiation on rice plants; this study revealed that N uptake was slightly enhanced and the number of spikelets increased about 10% compared to continuously flooded plots (CF). Ceesay et al. (Citation2006) compared the rice growth between an SRI plot with AWD water management during the vegetative stage, and a conventional (CF) plot. AWD water management increased the effective tiller ratio from 30–40% (with CF) to 70% (SRI) and the yield increased from 2.5 t ha−1 (CF) to 7.3 t ha−1. Although they attributed the effect of AWD to the enhanced nitrogen supply caused by drying and rewetting of soil (Birch Citation1958; Inubushi and Wada Citation1987), so-called soil-drying effects occur only after the soils are dried to about −1500 kPa (pF4) (Toriyama and Sekiya Citation1991). Different mechanisms might work for this increased N supply under AWD conditions (Seneviratne and Wild Citation1985; Schmidt-Rohr et al. Citation2004). Thakur et al. (Citation2010 Citationb) suggested there is enhanced growth of roots under saturated (not flooded) soil conditions. Yang et al. (Citation2004) showed higher root biomass and root activity as well as higher N and P uptake under the alternate wetting and drying treatment rather than continuous flooding. Drained management of soil during the vegetative stage seems to be essential for yield increase. Sato et al. (Citation2011) shows that the yield of SRI management of individual small areas within a paddy field correlates with the elevation of each location; a 0.5 t ha−1 yield increase was observed for each 1cm increase in elevation. Although this could be supportive data for drainage effect on yield, the possibility of increasing the depth of plow layer soil should be assessed quantitatively.
N transformation in an oxic rhizosphere
Numbers as well as diversity of AOB on root surfaces of different rice varieties were measured by the molecular biological method (PCR-DGGE), and these were larger on the root surfaces than in the bulk soil (Briones et al. Citation2003). Hybrid rice, accompanied by a larger number of AOB as compared to the conventional rice, could take up 12 to 30% more nitrogen from 15N labeled NH4 in the soil. This suggests a synergistic effect of co-provision of NH4 and NO3 nitrified on the root surface (Briones et al. Citation2003). Li et al. (Citation2008) measured AOB and nitrate reductase activity (NRA) of rhizosphere soil and bulk soil by using rice varieties with different O2-supplying activity from roots. Both AOB and NRA were measured in the order rhizosphere > bulk soil > root surface, and there was a close correlation between rice growth (N uptake, dry weight) and those factors which related to nitrification and nitrate assimilation (AOB, nitrification activity, leaf NRA), and they suggested high nitrogen use efficiency (NUE) of rice owing to nitrification in the rhizosphere soil. Chu et al. (Citation2009) measured AOB of temporary reconverted paddy field by PCR-DGGE methods and reported the great impact on population size and community structure of soil AOB in paddy fields, which depends strongly on the crop type used for upland conversion. Kaneta (Citation1993) showed the extremely high yield in a paddy field after reconversion from paddy-upland rotation, and attributed it to the uptake of subsoil N by penetration of roots through cracks in the soil formed during the rotation. However, an oxic rhizosphere could also be a cause of a high yield, because vigorous nitrification in the oxic rhizosphere soil might occur, as suggested by the AOB study above (Chu et al. Citation2009).
As mentioned above, drained oxic soil together with higher nitrate supply to the rice plants could enhance the N uptake by the plants. Therefore, the physiological effect of nitrate acquisition will be discussed in the following section.
Nitrate, Its Uptake and Transport, and Effect On Growth of Rice Plants
Characteristics of seedlings with nitrate supply
Yamasaki and Seino (Citation1965) examined the characteristics of seedlings that received nitrate fertilizer grown under either upland or submerged conditions, and showed that the nitrate content of rice seedlings was increased by nitrate fertilizer, and the oxidative activity as measured by α-naphtylamine and the ability for rooting were superior to seedlings without nitrate application. Moreover, the high nitrate content was maintained for more than one month after transplanting and showed the translocation of NO3 into new roots in the field. Mishra and Salokhe (Citation2008) examined the effect of different water regimes before and after transplanting and showed that nursery water management affected the rate of development after transplanting. They also showed that a dry seedbed was better than a wet seedbed, as the former helps to shorten the phyllochron (period of leaf emergence) and helps produce seedlings with better rooting. Both reports emphasized the slow but lasting effect of aerobically-grown seedlings on growth of transplanted rice.
Effect of nitrate supply during the vegetative stage
Although the supply of nitrate during the vegetative stage did not increase the NUE for dry matter production during the vegetative stage, the efficiency for spikelet formation was increased (Seino Citation1969). This suggested a different physiological role of nitrate as compared to ammonium. The growth pattern obtained by nitrate uptake during the vegetative stage resembled the growth patterns shown in the case of high yield, and cation uptake accompanied by nitrate was also suggested to contribute to the yield increase (Seino Citation1969). Yamamuro (Citation1975) compared the effect of nitrate and ammonium on rice growth in field experiments by taking cautions to make an almost equal amount of N uptake. The effect of nitrate uptake in the vegetative stage was not fast, but it resulted in dry matter increase in the following reproductive stage, which was similar to Seino's speculation; therefore Yamamuro (Citation1975) examined the translocation of nitrogen taken up at vegetative growth stages by using a 15N tracer, and realized that NO3 has a tendency to accumulate in the leaf sheath more than NH4. This suggested that once taken up, NO3 in the vegetative stage could contribute to the photosynthesis of the following growth stage. Recently, some supporting results for this phenomenon have been reported by Fan et al. (Citation2007). They measured leaf nitrate reductase activity (NRA) by nitrate withdrawal from the culture solution by using rice varieties with different NUE. They measured less decrease of leaf NRA after nitrate withdrawal in rice varieties with high NUE, together with a faster decrease of nitrate in the vacuoles of epidermal cells of roots and leaves. Kronzucker et al. (Citation2000) examined the flow of nitrate and ammonium taken up by rice roots by using a short time 13N tracer, and found there was more efficient behavior of nitrate than ammonium such that in the total N uptake, 24% of NH4 taken up was transported to the stem, while 37% of NO3 went there; and there was 24% efflux loss for NH4 and less than 10% for NO3. Hayashi et al. (Citation1997) measured the assimilation of NO3 by using 15N or 13N and reported their rapid (within 10 min) assimilation and translocation. These results suggest an important role for nitrate assimilation in rice plants, and the effect of nitrate uptake on dry matter production in the longer term (on the order of a few days) should be examined.
Mechanisms of nitrate uptake
It is known that nitrogen uptake can be characterized by using Michaelis–Menten kinetics with two parameters, Km and Vmax, which show the affinity of the uptake sites for the nutrient and the maximum uptake rate, respectively. Youngdahl et al. (Citation1982) showed that nitrate obeys the Michaelis–Menten kinetics, which suggests the connection of nitrate uptake to metabolism. Crawford and Glass (Citation1998) showed that nitrate is taken up by two transporters, with high and low substrate affinities.
The Km value for the high affinity nitrate transporter was measured as 26 µmol L−1, while the Km for the ammonium transporter was 51 µmol L−1 (Kronzucker et al. Citation2000). This means a higher affinity for nitrate transporters than for ammonium.
An interesting phenomenon that has been known for a long time is that the co-provision of NH4 and NO3 enhances the uptake of total nitrogen (Ta and Ohira Citation1981). Recent works (Kronzucker et al. Citation1999; Kirk Citation2001; Duan et al. Citation2006, Citation2007a, Citation2007b; Zhao et al. Citation2008) have revealed a part of this mechanism, which suggests the synergistic effect of NO3 on NH4 uptake in lowland rice (Kronzucker et al. Citation1999). Duan et al. (Citation2007a, Citation2007b) showed that there is no change in Km for NH4, while there is a large increase in Vmax for NH4, which means transporters for NH4 increase under the co-provision of NH4 and NO3. This hypothesis was verified by using a molecular biological approach (quantitative real time PCR) showing NO3 can enhance the expression of genes for NH4 transporters as well as genes for its assimilation; however, post-transcriptional processes need to be investigated (Zhao et al. Citation2008).
Yoneyama and Kumazawa (Citation1972) showed, using rice seedlings, that NO3 assimilation in leaves decreased more than that of NH4 under dark conditions. Similarly, Mengel and Viro (Citation1978) showed, using the 15N tracer method and 9-week-old rice plants, that uptake and assimilation of NO3 were affected by light intensity more than those of NH4. Ta and Ohira (Citation1981) showed that light intensity affects dry matter production under NO3 nutrition more than with ammonium. These findings suggest that NO3 assimilation requires photosynthetic energy.
It is suggested from these pieces of evidence that nitrate taken up by roots moves to the stem, and some part of it could be stored until assimilated in the stem or leaves when the energy status is enough to supply abundant NAD(P)H to the nitrate reductase in the leaf, as suggested by Bloom (1997).
Varietal differences exist in NO3 uptake (Luo et al. Citation1993; Yang et al. Citation1999; Duan et al. Citation2006, Citation2007a, Citation2007b; Fan et al. Citation2007; Senoo et al. Citation2007). Similarly, there is a varietal difference in NRA, e.g., indica has higher activity than japonica (Barlaan and Ichii Citation1996). Duan et al. (Citation2007b) suggested that varieties with high NUE showed an increase in NH4 uptake under the co-provision of NO3, and the relevant genes for NH4 transporter and transporter level also increased. The temporal change of NO3 content in the shoots of hybrid rice and a conventional variety grown under field conditions were compared, and it was shown that hybrid rice with higher NUE contains more NO3 than the conventional variety during the growth stage between late tillering to heading (Yang et al. Citation1999). In addition, dense superficial roots of hybrid rice distributed in the surface soil may be one of the causes of this high NO3 uptake (Yang et al. Citation1999).
Rice yield potential under aerobic soil conditions
Submerged paddy field conditions have been believed to show the higher yield potential of lowland rice than upland conditions. However, recent investigations by Kato et al. (Citation2009) and Katsura et al. (Citation2010) have suggested the possibility that upland conditions without severe water stress could be more appropriate to show the potential ability of rice plants. Katsura et al. (Citation2010) showed significantly higher radiation use efficiency (RUE) as well as a higher fraction of radiation intercepted under upland conditions (water potential was between ca.−5 kPa and ca.−80 kPa) compared to submerged ones. When Thakur et al. (Citation2010b) assessed the physiological effects of saturated water management (SRI) practice with submerged soil conditions (recommended management practice), this showed higher intercepted radiation during the reproductive stage as well as increase of rooting depth and RUE for SRI management. These findings suggest the involvement of nitrate uptake for such improvement of RUE. Nemoto et al. (Citation2010) conducted a preliminary examination of NUE by using genetic analysis (QTL analysis) under the condition of co-provision of NH4 and NO3 and suggested the involvement of the gene for ferredoxin-NADP-reductase (FNR). FNR is known to be responsible for nitrate assimilation as well as photorespiration. These findings also suggest that nitrate uptake in SRI might be favorable for high productivity.
Hypothetical scheme to explain the possible high yield by SRI management
To investigate various aspects of the possibility to increase rice yield by SRI management, a hypothetical scheme is presented based on the above review ().
Concluding Remarks – Research To Be Addressed
The authors conducted some surveys on SRI in Indonesia to learn the actual state of SRI cultivation by farmers. We were impressed that some of them attain very high yields (like 10 t ha−1) only by changing their cultivation practices from conventional to SRI management, without using costly external inputs. In particular, the introduction of non-submerging or alternate wetting and drying water management seemed to be related to higher yields (Toriyama et al. Citation2010). Therefore, the characteristics of non-submerged paddy soil and transformation of inorganic nitrogen in such environment as well as the effect of nitrate uptake on dry matter production were highlighted. However, the reported results of field trials of SRI treatment are variable. One of the reasons for the discrepancies might be the inexact expression of soil moisture conditions for SRI treatment plots. It is important to develop a suitable index to express the moisture and aerobic condition of paddy soil. The use of a measurable index such as soil water potential (kPa), degree of saturation (%), etc., in scientific data acquisition will be essential to scientific discussion as well as to generalize SRI practice. From a practical point of view, the applicability of SRI based on soil characteristics and topography should also be studied further (Belder et al. Citation2007), together with direct seeding of SRI to reduce the labor of transplanting. In addition, adverse effects of drainage and aerobic management on paddy field soil should be assessed, because there is concern about a possible decrease of organic matter in the long run, or some biotic stress in aerobic soil environments (Sasaki et al. Citation2010).
Research on SRI has been diverse in terms of physicochemical and microbiological study (Randriamiharisoa et al. Citation2006) of aerobic soil environment, to the physiology and molecular biology of rice plants. High plasticity of rice plants, which may lead to the breeding of more suitable varieties for SRI management based on molecular biology, is also suggested. From both environmental and production aspects, SRI related research would also be a good example for improving nitrogen use efficiency in agricultural ecosystems (Dawson et al. Citation2008) to meet the demand of more food with less input in developing countries.
Acknowledgments
The authors express our thanks to Prof. Norman Uphoff, Cornell International Institute for Food, Agriculture and Development, Ithaca, USA for reviewing the draft of this manuscript and offering valuable comments.
References
- Africare, Oxfarm America, WWF-ICRISAT Project 2010: More rice for people, more water for planet. WWF-ICRISAT Project, Hyderabad, India
- Ando , H . 2006 . “ Absorption of N and Si by high yielding rice in relation to soil properties ” . In Improvement of productivity and quality of rice in relation to nutrition and physiology , Edited by: Makino , A . 55 – 82 . Tokyo : Hakuyusha . (in Japanese)
- Barlaan , EA and Ichii , M . 1996 . Genotypic variation in nitrate and nitrite reductase activities in rice (Oryza sativa L.) . Breeding Sci. , 46 : 125 – 131 .
- Belder , P , Bouman , BAM and Spiertz , JHJ . 2007 . Exploring options for water savings in lowland rice using a modeling approach . Agr. Sys. , 92 : 91 – 114 .
- Birch , HE . 1958 . The effect of soil drying on humus decomposition and nitrogen . Plant Soil , 10 : 9 – 31 .
- Bloom , AJ . 1997 . “ Nitrogen as a limiting factor: crop acquisition of ammonium and nitrate ” . In Ecology in Agriculture , Edited by: LE , Jackson . 145 – 172 . London : Academic Press . 1st edition, ISBN-10:0123782600
- Bouman , BAM , Humphreys , E , Tuong , TP and Barker , R . 2007 . Rice and water . Advances in Agronomy , 92 : 187 – 237 .
- Briones , AM , Okabe , S , Umemiya , Y , Ramsing , NB , Reichardt , W and Okuyama , H . 2003 . Ammonia-oxidizing bacteria on root biofilms and their possible contribution to N use efficiency of different rice cultivars . Plant Soil , 250 : 335 – 348 .
- Ceesay , M , Reid , WS , Fernandes , ECM and Uphoff , N . 2006 . Effects of repeated soil wetting and drying on lowland rice yield with system of rice intensification (SRI) methods . Int. J. Agric Sust. , 4 : 5 – 14 .
- Chang , TT and Li , CC . 1991 . “ Genetics and breeding ” . In Rice, Volume 1: Production , Edited by: Luh , Bor S . 23 – 101 . New York : Van Nostrand Reinhold .
- Chapagain , T and Yamaji , E . 2010 . The effects of irrigation method, age of seedling and spacing on crop performance, productivity and water-wise rice production in Japan . Paddy Water Environ. , 8 : 81 – 90 .
- Chu , H , Morimoto , S , Fujii , T , Yagi , K and Nishimura , S . 2009 . Soil ammonia-oxidizing bacterial communities in paddy rice fields as affected by upland conversion history . Soil Sci. Soc. Amer. J. , 73 : 2026 – 2031 .
- Crawford , NM and Glass , ADM . 1998 . Molecular and physiological aspects of nitrate uptake by plants . Trends Plant Sci. , 3 : 389 – 395 .
- Dawson , JC , Huggins , DR and Jones , SS . 2008 . Characterizing nitrogen use efficiency in natural and agricultural ecosystems to improve the performance of cereal crops in low-input and organic Agricultural Systems . Field Crops Res. , 107 : 89 – 101 .
- Dobermann , A . 2004 . A critical assessment of the system of rice intensification (SRI) . Agr. Sys. , 79 : 261 – 281 .
- Dobermann , A , Witt , C and Dawe , D . 2002 . Site-specific nutrient management for intensive rice cropping systems in Asia . Field Crops Res. , 74 : 37 – 66 .
- Duan , YH , Yin , XM , Zhang , YL and Shen , QR . 2007a . Mechanism of enhanced rice growth and nitrogen uptake by nitrate . Pedosphere , 17 : 697 – 705 .
- Duan , YH , Zhang , YL , Shen , QR and Wang , SW . 2006 . Nitrate effect on rice growth and nitrogen absorption and assimilation at different growth stages . Pedosphere , 16 : 707 – 717 .
- Duan , YH , Zhang , YL , Ye , LT , Fan , XR , Xu , GH and Shen , QR . 2007b . Responses of rice cultivars with different nitrogen use efficiency to partial nitrate nutrition . Ann. Bot. , 99 : 1153 – 1160 .
- Fan , XR , Jia , LJ , Li , YL , Smith , SJ , Miller , AJ and Shen , QR . 2007 . Comparing nitrate storage and remobilization in two rice cultivars that differ in their nitrogen use efficiency . J. Exp. Bot. , 58 : 1729 – 1740 .
- Fernandes ECM, Uphoff N (2002): Summary from conference reports. In Assessment of the System of Rice Intensification (SRI), Proceedings of an International Conference, Sanya, China, April 1–4, pp. 33–39
- Hasebe , A , Koike , I , Ohmori , M and Hattori , A . 1987 . Variations in the process of nitrification and nitrate reduction in submerged paddy soils as measured by 15 N isotope dilution technique . Soil Sci. Plant Nutr. , 33 : 201 – 211 .
- Hayashi , H , Okada , Y , Mano , H , Kume , T , Matsuhashi , S , S-Ishioka , N , Uchida , H and Chino , M . 1997 . Detection and characterization of nitrogen circulation through the sieve tubes and xylem vessels of rice plants . Plant Soil , 196 : 233 – 237 .
- Honya , K . 1989 . Elucidation of high yield rice cultivation-for the crossroad of rice farming , 1 – 148 . Tokyo : Hakuyusha . (in Japanese)
- Horie , T , Shiraiwa , T , Honmma , K , Katsura , K , Mada , S and Yoshida , H . 2005 . Can yields of lowland rice resume the increase that they showed in the 1980s? . Plant Prod. Sci. , 8 : 257 – 272 .
- Inubushi , K and Wada , H . 1987 . Easily decomposable organic matter in paddy soils,VII: Effect of various pretreatments on N mineralization in submerged soils . Soil Sci. Plant Nutr. , 33 : 567 – 576 .
- Jackson , MB and Armstrong , W . 1999 . Formation of aerenchyma and the processes of plant ventilation in relation to soil flooding and submergence . Plant Biol. , 1 : 274 – 287 .
- Kaneta , Y . 1993 . Effect of paddy-upland rotation management on the productivity of rice and establishment of stable production technique for high yield of rice in –ill-drained heavy soils of Hachirogata reclaimed fields . Bull. Akita Agric. Exp. Stn. , 33 : 1 – 45 .
- Kato , Y , Okami , M and Katsura , K . 2009 . Yield potential and water use efficiency of aerobic rice (Oryza sativa L.) in Japan . Field Crops Res. , 113 : 328 – 334 .
- Katsura , K , Okami , M , Mizunuma , H and Kato , Y . 2010 . Radiation use efficiency, Naccumulation and biomass production of high-yielding rice in aerobic culture . Field Crops Res. , 117 : 81 – 89 .
- Kirk , GJD . 2001 . Plant-mediated processes to acquire nutrients: nitrogen uptake by rice plants . Plant Soil , 232 : 129 – 134 .
- Kirk , GJD . 2003 . Rice root properties for internal aeration and efficient nutrient acquisition in submerged soil . New Phytol. , 159 : 185 – 194 .
- Kirk , GJD and Kronzucker , HJ . 2005 . The potential for nitrification and nitrate uptake in the rhizosphere of wetland plants: A modeling study . Ann. Bot. , 96 : 639 – 646 .
- Kobayashi , A , Suzuki , Y , Saiten , H , Morooka , M and Ishida , H . 2000 . Effects of early release of ponding water on growth and yield of wetland rice under heavy application of rice straw in clayey gley paddy soil . Bull. Hokuriku Agric. Exp. Stn. , 43 : 25 – 45 . (in Japanese with English summary)
- Kondo , M and Yasuda , M . 2003 . Seasonal changes in N2 fixation activity and N enrichment in paddy soils as affected by soil management in the northern area of Japan . Jpn. Agr. Res. Quaterly , 37 : 105 – 111 .
- Kronzucker , HJ , Glass , ADM , Siddiqi , MY and Kirk , GJD . 2000 . Comparative kinetic analysis of ammonium and nitrate acquisition by tropical lowland rice: implications for rice cultivation and yield potential . New Phytol. , 145 : 471 – 476 .
- Kronzucker , HJ , Siddiqi , MY , Glass , ADM and Kirk , GJD . 1999 . Nitrate-ammonium synergism in rice: a subcellular flux analysis . Plant Physiol. , 119 : 1041 – 1046 .
- Li , YL , Fan , XR and Shen , QR . 2008 . The relationship between rhizosphere nitrification and nitrogen-use efficiency in rice plants . Plant Cell Environ. , 31 : 73 – 85 .
- Lin , X , Zhou , W , Zhu , D and Zhang , Y . 2005 . Effect of SWD irrigation on photosynthesis and grain yield of rice (Oryza sativa L.) . Field Crops Res. , 94 : 67 – 75 .
- Lin , XQ , Zhu , DF , Chen , HZ , Cheng , SH and Uphoff , N . 2009 . Effect of plant density and nitrogen fertilizer rates on grain yield and nitrogen uptake of hybrid rice (Oryza sativa L.) . J. Agric. Biochem. Sust. Develop. , 1 : 44 – 53 .
- Luo , AC , Xu , JM and Yang , XE . 1993 . Effect of nitrogen supply on absorption of ammonium and nitrate by conventional and hybrid rice during reproductive growth . Plant Soil , 155/156 : 395 – 398 .
- Matsuoka , M , Furbank , RT , Fukayama , H and Miyao , M . 2001 . Molecular engineering of C-4 photosynthesis . Ann. Rev. Plant Physiol. Plant Mol. Biol. , 52 : 297 – 314 .
- McDonald , AJ , Hobbs , PR and Riha , SJ . 2006 . Does the system of rice intensification outperform conventional best management? A synopsis of the empirical record . Field Crops Res. , 96 : 31 – 36 .
- McDonald , AJ , Hobbs , PR and Riha , SJ . 2008 . Stubborn facts: Still no evidence that the system of rice intensification of rice out-yields best management practices (BMPs) beyond Madagascar . Field Crops Res. , 108 : 188 – 191 .
- Mengel , K and Viro , M . 1978 . The significance of plant energy status for the uptake and incorporation of NH4-nitrogen by young rice plants . Soil Sci. Plant Nutr. , 24 : 407 – 416 .
- Mishra , A and Salokhe , VM . 2008 . Seedling characteristics and early growth of transplanted rice under different water regimes . Exp. Agric. , 44 : 365 – 383 .
- Mishra , A and Salokhe , VM . 2010 . The effects of planting pattern and water regime on root morphology, physiology and grain yield of rice . J. Agron. Crop Sci. , 196 : 368 – 378 .
- Miyazato , T , Robert , A and Lazaro , RC . 2010 . Irrigation management transfer (IMT) and system of rice intensification (SRI) practice in the Philippines . Paddy Water Environ. , 8 : 91 – 97 .
- Nemoto , K , Morita , S and Baba , T . 1995 . Shoot and root development in rice related to the phyllochron . Crop Sci. , 35 : 24 – 29 .
- Nemoto , K , Yamagishi , J and Yamagishi , T . 2010 . Eco-physiological significance of nitrogen usage efficiency in rice as revealed by QTL analysis . Jpn. J. Crop Sci. , 79 ( suppl. 1 ) : 418 – 419 . (in Japanese)
- Nguu , NV and De Datta , SK . 1979 . Increasing of plant density and plant geometry . Field Crops Res. , 2 : 19 – 34 .
- Patrick , WH Jr and Reddy , KR . 1976 . Nitrification-denitrification reactions in flooded soils and water bottoms: dependence on oxygen supply and ammonium diffusion . J. Environ. Qual. , 5 : 469 – 472 .
- Rafaralahy S (2002): An NGO perspective on SRI and its origins in Madagascar. In Assessment of the System for Rice Intensification (SRI), Proceedings of an International Conference, Sanya, China, April, 1–4, 2002
- Randriamiharisoa , R , Barison , J and Uphoff , N . 2006 . “ Soil biological contributions to the System of Rice Intensification ” . In Biological Approaches to Sustainable Soil Systems , Edited by: Uphoff , N. Boca Raton, , FL : CRC Press .
- Roger , PA and Kulasooriya , SA . 1980 . Blue-Green Algae , Los Banos, Philippines : IRRI .
- Sasaki , R . 2000 . Submergence tolerance of transplanted rice seedlings with several plant ages in leaf number-relationship between the growth during submergence and survival . Jpn. J. Crop Sci. , 69 : 372 – 379 .
- Sasaki , R . 2004 . Characteristics and seedling establishment of rice nursling seedlings . Japan Agric. Res. Quarterly , 38 : 7 – 13 .
- Sasaki , Y , Hosen , Y , Peng , S , Nie , L , Rodriguez , R , Agbisit , R , Fernandez , L and Bouman , BAM . 2010 . Do abiotic factors cause a gradual yield decline under continuous aerobic rice cultivation? A pot experiments with affected field soils . Soil Sci. Plant Nutr. , 56 : 476 – 482 .
- Sasaki , R and Hoshikawa , K . 1997a . Changes in energy dependence and morphological characteristics with the development of rice nursling seedlings raised under different light and temperature conditions . Jpn. J. Crop Sci. , 66 : 252 – 258 .
- Sasaki , R and Hoshikawa , K . 1997b . The role of crown roots from coleoptilar node in the rooting and development of transplanted rice nursling seedlings . Jpn. J. Crop Sci. , 66 : 259 – 267 .
- Sato S, Uphoff N (2007): A review of on-farm evaluation of system of rice intensification (SRI) methods in eastern Indonesia. CAB Reviews: Perspectives in Agriculture, Veterinary Science, Nutrition and Natural Resources, 2. Commonwealth Agricultural Bureau International, Wallingford, UK
- Sato S, Yamaji E, Kuroda T 2011: Strategies and engineering adaptations to disseminate SRI methods in large-scale irrigation systems in eastern Indonesia, Paddy Water and Envir., 9, 79–88
- Satyanarayana , A , Thiyagarajan , TM and Uphoff , N . 2007 . Opportunities for water saving with higher yield from the system of rice intensification . Irrig. Sci. , 25 : 99 – 115 .
- Schmidt-Rohr , K , Mao , JD and Olk , DC . 2004 . Nitrogen-bonded aromatics in soil organic matter and their implications for a yield decline in intensive rice cropping . PNAS. , 101 : 6351 – 6354 .
- Seino , K . 1969 . Method of utilizing nitrate nitrogen in the rice cultivation with high yield . Nogyo oyobi Engei. , 44 : 1383 – 1388 . (in Japanese)
- Senevirantne , R and Wild , A . 1985 . Effect of mild drying on the mineralization of soil nitrogen . Plant Soil , 84 : 175 – 179 .
- Senoo , S , Toriyama , K and Fukuta , Y . 2007 . Varietal difference of the response to the different nitrogen source in rice (Oryza sativa, L.) at vegetative growth stage . Jpn. J. Crop. Sci. , 76 : 58 – 59 . (in Japanese)
- Sheehy , JE . 2000 . “ Limits to yield for C3 and C4 rice: an agronomist's view ” . In Redesigning Rice Photosynthesis to Increase Yield , Edited by: Sheey , JE , Mitchell , PL and Hardy , B . 39 – 52 . Makati City, Philippines, and Elsevier Science, Amsterdam : IRRI .
- Sheehy , JE , Peng , S , Dobermann , A , Mitchell , PL , Ferrer , A , Yang , J , Zou , Y , Zhong , X and Huang , J . 2004 . Fantastic yields in the system of rice intensification: fact or fallacy? . Field Crops Res. , 88 : 1 – 8 .
- Sinclair , TR and Cassman , KG . 2004 . Agronomic UFOs . Field Crops Res. , 88 : 9 – 10 .
- Stoop WA (2005): The system of rice intensification (SRI): Results from exploratory field research in Ivory Coast—Research needs and prospects for adaptation to diverse production systems of resource-poor farmers, http://ciifad.cornell.edu/sri/Stoopwarda05.pdf (November 2010)
- Stoop , WA and Kassam , AH . 2005 . The SRI controversy: A response . Field Crops Res. , 91 : 357 – 360 .
- Stoop , WA , Uphoff , N and Kassam , AH . 2002 . Research issues raised for the agricultural sciences by the System of Rice Intensification (SRI) from Madagascar: Opportunities for improving farming systems for resource-limited farmers . Agr. Sys. , 71 : 249 – 274 .
- Surridge , C . 2002 . The rice squad . Nature , 416 : 576 – 578 .
- Surridge , C . 2004 . Feast or famine? . Nature , 428 : 360 – 361 .
- Ta , TC and Ohira , K . 1981 . Effect of various environmental and medium conditions on the response of indica and japonica rice plants to ammonium and nitrate nitrogen . Soil Sci. Plant Nutr. , 27 : 347 – 355 .
- Takahashi , J , Shoji , S , Nogi , T and Wada , G . 1973 . The fate of fertilizer nitrogen applied to the paddy field and its absorption by rice plant 5. The fate of basal nitrogen in the paddy field and its absorption by rice plant under the various cultural conditions . Proc. Crop Sci. Soc. Japan , 42 : 91 – 96 . (in Japanese with English summary)
- Takai , T , Matsuura , S , Nishio , T , Ohsumi , A , Shiraiwa , T and Horie , T . 2006 . Rice yield potential is closely related to crop growth rate during late reproductive period . Field Crops Res. , 96 : 328 – 335 .
- Tanaka , I . 1970 . Ecological study on irrigation method of rice plant . J. Cent. Agric. Expt. Stn. , 14 : 117 – 166 . (in Japanese with English summary)
- Thakur , AK . 2010 . Critiquing SRI criticism: Beyond scepticism with empiricism . Current Science , 98 : 1294 – 1299 .
- Thakur , AK , Rath , S , Roychowdhury , S and Uphoff , N . 2010a . Comparative performance of rice with system of rice intensification (SRI) and conventional management using different plant spacing . J. Agron. Crop Sci. , 196 : 146 – 159 .
- Thakur , AK , Uphoff , N and Antony , E . 2010b . An assessment of physiological effects of system of rice intensification (SRI) practices compared with recommended rice cultivation practices in India . Expl Agric. , 46 : 77 – 98 .
- Toriyama , K , Oda , M and Yokoyama , S . 2010 . Survey results of intensive rice cultivation (SRI) in West Java, Indonesia . Res. Tropic. Agr. , 3 : extra 1, 27 – 28 .
- Toriyama , K and Sekiya , S . 1991 . A method of forecasting the nitrogen-release pattern of paddy soils for the fertilizer management of rice plants. In Soil Management for Sustainable Rice Production in the Tropics . IBSRAM Monograph , 2 : 287 – 302 .
- Tsujimoto , Y , Horie , T , Randriamihary , H , Shiraiwa , T and Homma , K . 2009 . Soil management: the key factors for higher productivity in the fields utilizing the system of rice intensification (SRI) in the central highland of Madagascar . Agricultural System , 100 : 61 – 71 .
- Tsuno Y (1991): The theory of high yielding rice. In: The Outline of Agricultural Techniques in Crops. Fundamental Techniques: Rice. Vol. 2. Nosangyosonbunka-kyokai, Tokyo. 57–114 (in Japanese)
- Uphoff , N and Kassam , A . 2008 . A critical assessment of a desk study comparing crop production systems: The example of the “system of rice intensification” versus “best management practice” . Field Crops Res. , 108 : 109 – 114 .
- Uphoff N, Kassam A (2009): Case Study: System of Rice Intensification, Final report Agricultural Technologies for Developing Countries STOA Project “Agricultural Technologies for developing countries”, pp 1–65. FAO, Rome
- Uphoff N, Randriamiharisoa R, (2002): Reducing water use in irrigated rice production with the Madagascar System of Rice Intensification (SRI). In Water-wise Rice Production. Eds. BAM Bouman et al., pp 71–87, Proceedings of the International Workshop on Water-wise Rice Production, 8–11 April 2002: IRRI, Los Banos, Philippines
- Uphoff N, Fernandes ECM, Longping Y, Jiming P, Rafalahy S, Rabenandrasana J, eds. (2002): Assessments of the System of Rice Intensification: Proceedings of an International Conference, Sanya, China, April 1–4, 2002. 200pp
- Wang SH, Cao W, Jiang D, Dai T, Zhu Y (2002): Physiological characteristics and high-yield techniques with SRI, In: Assessment of the System for Rice Intensification (SRI), Proceedings of an International Conference, Sanya, China, April 1–4, pp. 116–124
- Yamamuro , S . 1975 . Effects of nitrate and ammonium nitrogen on the growth of rice plant . Bull. Hokuriku Natl. Agric. Exp. Stn. , 17 : 1 – 109 . (in Japanese with English summary)
- Yamasaki , T and Seino , K . 1965 . Use of nitrate fertilizer for the cultivation of paddy rice. Part 1. About the physiological character of rice seedlings supplied with nitrate as source nitrogen . J. Sci. Soil Manure Jpn. , 36 : 153 – 158 . (in Japanese)
- Yang , C , Yang , L , Yang , Y and Ouyang , Z . 2004 . Rice root growth and nutrient uptake as influenced by organic manure in continuously and alternate flooded paddy soils . Agric. Water Manag. , 70 : 67 – 81 .
- Yang , X , Zhang , WN , Ni , W and Dobermann , A . 1999 . Characteristics of nitrogen nutrition in hybrid rice . International Rice Research Notes , 24 : 5 – 8 .
- Yoneyama , T and Kumazawa , K . 1972 . Studies on the distribution of nitrogen from nitrate and ammonium sources in the leaves of rice seedlings . J. Sci. Soil Manure, Jpn. , 43 : 329 – 332 . (in Japanese)
- Youngdahl , LJ , Pacheco , R , Street , JJ and Vlek , PLG . 1982 . The kinetics of ammonium and nitrate uptake by young rice plants . Plant Soil , 69 : 225 – 232 .
- Zhang , H , Xue , Y , Wang , Z , Yang , J and Zhag , J . 2009 . An alternate wetting and moderate soil drying regime improves root and shoot growth in rice . Crop Science , 49 : 2246 – 2260 .
- Zhao , L , Wu , L , Li , U , Lu , X , Zhu , D and Uphoff , N . 2009 . Influence of the system of rice intensification on rice yield and nitrogen and water use efficiency with different N application rates . Expl. Agric. , 45 : 275 – 286 .
- Zhao , XQ , Zhao , SP and Shi , WM . 2008 . Enhancement of NH4 + uptake by NH3 − in relation to expression of nitrate-induced genes in rice (Oryza sativa) roots . Pedosphere , 18 : 86 – 91 .