Abstract
Low molecular weight (LMW) organic compounds in soil solution are easily biodegradable and could fuel respiration by soil microorganisms. Our main aim was to study the mineralization kinetics of monosaccharides using 14C-radiolabelled glucose. Based on these data and the soil solution concentrations of monosaccharides, we evaluated the contribution of monosaccharides to basal respiration for a variety of tropical forest soils. Further, the factors controlling the mineralization kinetics of monosaccharides were examined by comparing tropical and temperate forest soils. Monosaccharides comprised on average 5.2 to 47.7% of dissolved organic carbon in soil solution. Their kinetic parameters (V max and KM ), which were described by a single Michaelis-Menten equation, varied widely from 11 to 152 nmol g−1 h−1 and 198 to 1294 µmol L−1 for tropical soils, and from 182 to 400 nmol g−1 h−1 and 1277 to 3150 µmol L−1 for temperate soils, respectively. The values of V max increased with increasing microbial biomass-C in tropical and temperate soils, while the KM values had no correlations with soil biological or physicochemical properties. The positive correlation between V max values and microbial biomass-C indicates that microbial biomass-C is an essential factor to regulate the V max values in tropical and temperate forest soils. The biodegradation kinetics of monosaccharides indicate that the microbial capacity of monosaccharide mineralization far exceeds its rate at soil solution concentration. Monosaccharides in soil solution are rapidly mineralized, and their mean residence times in this study were very short (0.4–1.9 h) in tropical forests. The rates of monosaccharide mineralization at actual soil solution concentrations made up 22–118% of basal respiration. Probably because of the rapid and continuous production and consumption of monosaccharides, monosaccharide mineralization is shown to be a dominant fraction of basal respiration in tropical forest soils, as well as in temperate and boreal forest soils.
Introduction
Soils contain a large pool of organic carbon (C), corresponding to approximately twice as much as the carbon pool in the atmosphere, and three times as much as in the terrestrial biomass (Raich and Schlesinger 1991). Carbon sequestration in soils contributes to maintenance of the constant carbon dioxide concentration in the atmosphere. The ability of soils to act as a sink or source of carbon is regulated by the balance between input and the decomposition of organic matter (e.g., plant tissues) in soils. Consequently, knowledge of the mechanisms and factors controlling soil carbon dynamics is crucial to predict the responses of ecosystems to global warming or climate change (Schimel et al. Citation2001; Boddy et al. Citation2007; Hill et al. Citation2008).
Most of the organic soil carbon is supplied as plant tissues and root exudates (Paul and Clark Citation1996), and it is mineralized by the soil microbial community. Since microbes can only uptake compounds in a liquid state, the dissolved organic carbon (DOC) pool in soil solution could be the main C substrate for soil microorganisms (Marschner and Kalbitz Citation2003). DOC consists of both high molecular weight (HMW) compounds (e.g., fulvic and humic acids) and low molecular weight (LMW) compounds. LMW organic compounds include sugars (mono- and disaccharides), organic acids, amino acids, amino sugars, and phenolics (van Hees et al. Citation2005a). The size of the LMW organic carbon pool is very small in comparison to that of the solid soil organic C pool (van Hees et al. Citation2005a), and concentrations in soil solution are typically low (<100 µmol L−1), comprising <10% of total DOC in most cases (Jones Citation1998; Strobel Citation2001; Jones et al. Citation2003; van Hees et al. Citation2005a).
Carbohydrates (e.g., cellulose, hemicellulose and monomeric sugars) are the main components of plant tissues, root exudates and microorganisms (Paul and Clark Citation1996). Thus, monosaccharides are the most important substrates among LMW organic compounds. Monosaccharides in soil solution are supplied from several sources: exudation and turnover of roots and microorganisms; initial leaching from fresh leaves; throughfall, and extracellular solubilization of litter and soil organic matter (SOM) (Grayston et al. Citation1996; Hongve et al. Citation2000; Jones et al. Citation2003; Hill et al. Citation2008). However, microbial degradation rapidly removes monosaccharides from soil solution (van Hees et al. Citation2005b; Boddy et al. Citation2007); they are easily biodegradable with short half-lives ranging from <0.1 to approximately 12 h for microbial uptake in topsoils (Coody et al. Citation1986; Kuzyakov and Demin Citation1998; Nguyen and Guckert Citation2001; van Hees et al. Citation2005a; Boddy et al. Citation2007, Citation2008; Hill et al. Citation2008). Due to their rapid assimilation and mineralization by soil microorganisms, CO2 efflux from monosaccharides is likely to account for a considerable proportion (2–72%) of basal respiration (van Hees et al. Citation2005a, Citation2008; Fujii et al. Citation2010).
Although some studies have quantified the fluxes and dynamics of monosaccharides in temperate, boreal, and arctic ecosystems (van Hees et al. Citation2005a, Citation2008; Boddy et al. Citation2007, Citation2008; Fujii et al. Citation2010), no information is available for tropical forest soils. Since tropical forests are one of the main sinks of global soil organic carbon (Jobbágy and Jackson Citation2000), a mechanistic understanding of soil respiration through monosaccharides is of great importance to elucidate C dynamics under tropical forests.
Our main objective was to examine the mineralization kinetics of monosaccharides. Then, based on these data and the soil solution concentrations of monosaccharides, we aimed to evaluate the contribution of monosaccharide mineralization to basal respiration in tropical forest soils. Further, the factors controlling the mineralization kinetics of monosaccharides were examined by comparing the tropical and temperate forest soils.
Materials and Methods
Site description
Soils were collected from six tropical forest sites: Ban Rakpendin (RP) in Chiang Rai Province, Thailand; and Bukit Soeharto (BS), Bukit Bankirai (BB), and Kuaro (KR1, KR2 and KR3) located in East Kalimantan Province, Indonesia (). All sites have a tropical climate, and the mean annual air temperature and annual precipitation range from 25.0 to 27.0°C, and 1977 to 2427 mm, respectively (). The dominant overstory vegetation at all sites is broad-leaved trees (). The soils developed on sedimentary rocks and are classified as Ultisols at all sites, except for KR1 (Soil Survey Staff Citation2006). At KR1 the soil developed on serpentine and is classified as Oxisols (Soil Survey Staff Citation2006). To compare the mineralization kinetics of monosaccharides, three temperate forest soils were collected in Japan. The mean annual temperature air temperature and annual precipitation there range from 6.9 to 15.9°C, and 1422 to 1782 mm, respectively (). The soils are classified as Andisols (NG), Spodosols (TG) and Inceptisols (KT) (Soil Survey Staff Citation2006). The features of these sites and their soil properties are described in detail in Fujii et al. (Citation2010) and Fujii et al. (Citation2011).
Table 1. Site description
Soil sampling
All soil samples were collected from the A horizons during the wet season and were stored at 4°C prior to analysis. These “fresh” field moist, unsieved soils were used without eliminating fine roots for the soil solution extraction and mineralization kinetic studies. Sieved (<2 mm) field moist soil samples were used to determine the microbial biomass, and air-dried and sieved (<2 mm) soil samples were used for the physicochemical analyses. After sampling, the soil solution was extracted immediately and the mineralization kinetic experiment was performed within 3−4 months.
Physicochemical analyses and microbial biomass of soils
The volumetric water contents and bulk densities of the soils were determined by oven-drying core samples (100 cc) of field moist soils at 105°C for 24 h. Particle size distribution was determined by the pipette method (Gee and Bouder Citation1986). Soil pH was measured after shaking for 1 h, using a soil-to-solution (H2O) ratio of 1:5 (w/v). Total soil carbon and nitrogen (N) contents were determined using a CN analyzer (Vario Max CN, Elementar Analysensystem GmbH). The soil microbial biomass was determined on triplicate samples by the chloroform fumigation-extraction method (Vance et al. Citation1987). In brief, after sieved (<2 mm) field moist soils (equivalent to 10 g dry weight (DW) soil) were fumigated with ethanol-free CHCl3 for 24 h at 25°C, the CHCl3 was removed. The soluble C was then extracted with 0.5 mol L−1 K2SO4 (soil to solution ratio of 1:5) for 30 min on an orbital shaker from the fumigated soils and from comparable non-fumigated soil samples. The total organic carbon in the filtered extract was determined using a total organic carbon analyzer (TOC-V CSH, Shimadzu, Japan). A kec -factor of 0.45 (Wu et al. Citation1990) was used to calculate microbial biomass-C from the difference between fumigated and non-fumigated soils.
Soil solution extraction and chemical analyses
The centrifugation-drainage technique described by Giesler and colleagues (Citation1996) and Gallet and Keller (Citation1999) was used to extract soil solution in tropical forests. Intact (field moist) soil core samples (100 cc) were sampled in triplicate and centrifuged for 30 min at a speed of 8800 rpm (10560 g; ∼1.5 MPa; KOKUSAN centrifuge). The collected soil solutions were filtered through a 0.45-µm filter (Minisart RC15, Sartorius Inc.) and frozen prior to analysis. Soil solution pH was determined with a glass electrocode meter (pH/ion meter 225; Iwaki Glass). The concentrations of DOC in soil solution were determined using a total organic carbon analyzer (TOC-VCSH, Shimadzu, Japan). The concentrations of monosaccharides in soil solution were determined using periodate oxidation (Burney and Sieburth Citation1977; Johnson and Sieburth Citation1977) and glucose standards, since it is reported that composition of monosaccharides is similar across a variety of climates and soils (Derrien et al. Citation2007) and glucose represents the dominant proportion of monosaccharides in tropical soils (Trouve et al. Citation1996; Derrien et al. Citation2007).
Glucose mineralization kinetics
We chose glucose as a model substrate of monosaccharides since it probably represented the dominant C compound entering soil (Paul and Clark Citation1996; Derrien et al. Citation2007; Hill et al. Citation2008) and the mineralization kinetics of the monosaccharides other than glucose are reported to be similar to those of glucose (van Hees et al. Citation2008). Glucose mineralization kinetics in soils were determined for tropical and temperate forest soils according to van Hees et al. (Citation2002). A 14C-radiolabelled glucose solution (100 µL; 10–2000 µmol L−1 glucose, specific activity 1.7 kBq mL−1) (U-14C; American Radiolabelled Chemicals, Inc., 0.4 GBq mmol−1) was added to 1.00 ± 0.02 g of field moist soil (equivalent to 0.62–0.78 g DW soil) in a 50-mL polypropylene tube. Both tropical and temperate soils were incubated for 24 h at 25°C before measurement. Following glucose addition, the soil was gently shaken to ensure mixing and incubated for 0.5 h (RP) or 1 h (the other soils) at 25°C in tubes hermetically sealed with parafilm. The incubation time for the glucose mineralization was determined through a pilot experiment (data not presented). The 14CO2 produced during the mineralization of glucose was collected by placing a plastic scintillation vial containing 1.0 mL of 1 mol L−1 NaOH inside the tube, on top of the soil but separated from direct contact with the soil by a spacer. 14CO2 trapped as NaH14CO3 in the NaOH was determined by liquid scintillation counting (Aloka liquid scintillation system, LSC 3050, or Wallac 1000 scintillation counter, PerkinElmer Life Sciences) using alkali compatible scintillation fluid (Hionic Fluor, PerkinElmer Life Sciences). The experiments were performed in duplicate (below average of 5% for the relative standard deviation).
The data of mineralization kinetics were fitted to a single Michaelis-Menten equation:
Basal soil respiration
Basal soil respiration rates in tropical forest soils were determined in the laboratory by measuring CO2 evolution from field moist soils (equivalent to 10 g DW soil) which were incubated in the dark at 25°C for 1 h in 100-mL Erlenmeyer flasks sealed with silicon rubber septa (Sawada et al. Citation2008). Prior to incubation, the flasks were aerated for 15 min with CO2-free air passed through 2 mol L−1 NaOH in order to achieve a low background CO2 concentration in the headspace of the flasks (ca. 100 µL L−1). The measurement of basal soil respiration was performed under the same incubation temperature and moisture condition as the experiment of the mineralization kinetics for estimating the contribution of monosaccharides to basal respiration. The soils were analyzed in triplicate. Evolved CO2 was collected in glass vials using a syringe, measured with an infrared CO2 controller (ZFP9, Fuji Electric Instruments Co., Ltd.) and expressed as nmol CO2 g−1 h−1.
Calculations and statistics
All results were expressed on an oven–dry (105°C, 24 h) soil weight basis as the mean ± standard error (SE) of three determinations. The Michaelis-Menten equation was fitted to the data of mineralization kinetics using a least squares optimization procedure with SigmaPlot 11.0 (SPSS Inc. 2002). The statistical significance of the kinetic data was tested automatically by F test at P < 0.05 with SigmaPlot 11.0 (SYSTAT Software, Point Richmond, CA, USA). A Pearson's correlation coefficient test was conducted to examine the relationship between the kinetic parameters and the soil biological and physicochemical properties by SYSTAT 11 (SYSTAT software, Point Richmond, CA, USA). Relationships between the soil microbial biomass and physicochemical properties were tested by the same method. The combined standard errors for the mineralization rates of monosaccharides in soil solution were calculated by considering the variation for both kinetic parameters and monosaccharide concentrations in soil solution, based on maximum likelihood estimation (Gallant Citation1987) and propagation of error (Taylor Citation1997).
Results
Physicochemical properties of soils
The volumetric water contents of the soils in tropical and temperate forests varied from 19.9 to 47.9% and 27.5 to 59.8%, respectively (, depending on the water retention capacities of the soils. The soil pH varied from 4.0 to 6.3 for tropical soils and 3.8 to 4.6 for temperate soils, depending on parent materials and climates (Fujii et al. Citation2008, Citation2011). The microbial biomass-C varied from 29 ± 3 mg C kg−1 (mean ± SE) at BB to 259 ± 12 mg C kg−1 at RP. In temperate forest soils, microbial biomass-C was in the range of 196 ± 2 to 699 ± 41 mg C kg−1 and typically greater than that of tropical forest soils due to the higher amounts of soil organic matter in temperate soils (). Indeed, including data of temperate soils, microbial biomass-C correlated significantly with total carbon (r = 0.91, P < 0.05) and nitrogen content in soils (r = 0.87, P < 0.05). However, microbial biomass-C had no correlation with soil water content, clay content, soil pH and C/N ratio (P > 0.05).
Table 2. Physicochemical properties of soils in tropical and temperate forests
Soil solution composition
The soil solution was moderately acidic (pH of 5.2 to 6.4) at all tropical sites (). The concentrations of DOC and monosaccharides in soil solution varied widely from 2.8 ± 0.2 to 6.8 ± 0.2 mmol L−1 (mean ± SE) and from 41.9 ± 1.3 to 278.9 ± 11.4 µmol L−1, respectively (). The monosaccharides comprised a small portion of DOC in soil solution at all sites (5.2 to 17.4%), except for the highest value at KR2 ().
Table 3. Solution pH and concentrations of dissolved organic carbon (DOC) and monosaccharides in soil solution in tropical forests
Glucose mineralization kinetics
The glucose mineralization rate data conformed well to a single Michaelis-Menten kinetic equation ( and ; R 2 > 0.98) and their parameters were all significant (P < 0.05). According to the Michaelis-Menten parameters (V max and KM ) (), the maximum rates of glucose mineralization (V max) were 11 ± 1 to 152 ± 18 nmol g−1 h−1 (mean ± SE) in tropical forest soils. The concentrations at which the half-maximal mineralization rates occur (KM ) were 198 ± 19 to 1294 ± 188 µmol L−1 (mean ± SE) in tropical soils (). Both V max and KM were higher at RP than at the other sites (P < 0.05). In temperate forest soils, V max and KM ranged from 182 ± 39 to 400 ± 122 nmol g−1 h−1 (mean ± SE) and 1277 ± 327 to 3150 ± 1268 µmol L−1, respectively (). Both kinetic parameters of temperate soils were similar to RP, or an order of magnitude higher than the other tropical soils (). The V max values in tropical and temperate soils were correlated with the microbial biomass-C (r = 0.94, P < 0.01; ), while the values of KM had no significant correlation with microbial biomass-C (r = 0.58, P > 0.05; ). No relationships were found between both kinetic parameters and soil water content, clay content, soil pH and C/N ratio (P > 0.05).
Figure 1. Concentration-dependent mineralization of glucose in tropical (a) and temperate (b) forest topsoils at 25°C and field moist condition. Soil solution concentrations represent glucose concentrations in soil solution diluted by the intrinsic soil water after substrate addition (2.1–627.2 µmol L−1 for (a) and 1.4–452.4 µmol L−1 for (b)). Symbols denote experimental data points, while the curves represent fits to a single Michaelis-Menten equation. RP, Rakpendin; BS, Bukit Soeharto; BB, Bukit Bankirai; KR, Kuaro; NG, Nagano; TG, Tango; KT, Kyoto.
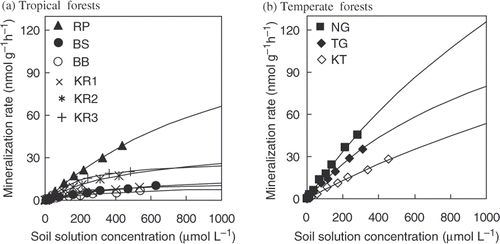
Figure 2. The relationship between the kinetic parameters and soil microbial biomass in tropical and temperate forest soils at the same incubation temperature (25°C). Figures (a) and (b) show the relationship between microbial biomass carbon (C) and the Vmax and KM values, respectively. Symbols denote the following: • are the data of tropical soils, and ▪ are those of temperate soils. The Pearson's correlation coefficients and probability are presented.
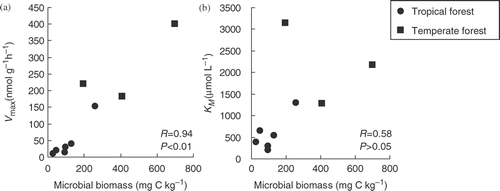
Table 4. Michaelis-Menten kinetic parameter values (Vmax and KM ) at 25°C in tropical and temperate forest soils
Rates of monosaccharide mineralization and basal soil respiration
The rates of monosaccharide mineralization at their concentrations in the field soil solution were estimated using Eq. Equation1, monosaccharide concentrations in soil solution () and the kinetic parameters ( and ), assuming that monosaccharides in soil solution are utilized by soil microorganisms as described by mineralization kinetics (), and that monosaccharides are mineralized at a similar rate as glucose. The mineralization rates of monosaccharides ranged from 9.0 ± 2.3 to 81.9 ± 3.5 nmol CO2 g−1 h−1 (mean ± SE), and the highest rate was observed at KR2 ().
Figure 3. The estimated mineralization rates of monosaccharides at soil solution concentrations and basal respiration rates in tropical forest topsoils. Bars indicate standard errors (n = 2 and 3 for combined standard errors of mineralization rates of monosaccharides, and n = 3 for basal respiration rates).
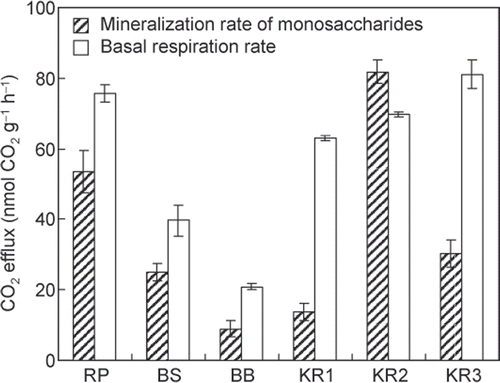
As shown in , basal soil respiration rates ranged from 20.8 ± 0.9 to 81.2 ± 4.1 nmol CO2 g−1 h−1, and were comparable to the mineralization rates of monosaccharides, which were determined under the same temperature and moisture conditions. The mineralization rates of monosaccharides corresponded to 22–118% of basal soil respiration in tropical forests ().
Discussion
Concentrations of DOC and monosaccharides in soil solution
Monosaccharides could be supplied into soil solution from living or dead plants and microorganisms via solubilization of plant litter, microbes, or root exudation. The concentrations of DOC and monosaccharides in soil solution could vary depending on vegetation and soil types, soil depth, and seasonality (Strobel Citation2001; Kawahigashi et al. Citation2003; van Hees et al. Citation2008; Fujii et al. Citation2010). In tropical forest topsoils, monosaccharide concentrations in soil solution were low and varied with sites, although DOC concentrations in soil solution were similar (). Their concentrations in soil solution were in agreement with the ranges observed in the previous studies in the temperate, boreal and arctic natural ecosystems (4–2027 µmol L−1 for monosaccharides and 0.4–18 mmol L−1 for total DOC, <5−51% of DOC; Kawahigashi et al. Citation2003; Boddy et al. Citation2007, Citation2008; Nambu et al. Citation2008; van Hees et al. Citation2008; Fujii et al. Citation2010). Low concentrations of monosaccharides in soil solutions seem to be common in temperate, boreal and tropical forests. Due to their lack of charge, monosaccharides are not adsorbed to the soil solid phase, unlike LMW organic anions (Jones and Edwards Citation1998; van Hees et al. 2003; Kuzyakov and Jones Citation2006; Hill et al. Citation2008; Fujii et al. Citation2010), so the concentration of monosaccharides in soil solution is dependent on the balance between their production and consumption, e.g., rapid mineralization and assimilation by microorganisms (Kawahigashi et al. Citation2003; van Hees et al. Citation2008; Fujii et al. Citation2010). Judging from low adsorption of monosaccharides, the low concentrations of monosaccharides in soil solution in tropical forest topsoils are thought to be caused by rapid mineralization and assimilation by microorganisms, as discussed later in detail.
Factors affecting the mineralization kinetic parameters of glucose
The mineralization rates of glucose in soil solution are concentration-dependent, which can be well described by a single Michaelis-Menten equation (), although some parameters have large variation due to the high linearity in topsoils and the lower concentration ranges studied ( and ). The kinetic parameters (V max and KM ) in tropical and temperate forest soils (11 ± 1 − 400 ± 122 nmol g−1 h−1 for V max and 198 ± 19 −3150 ± 1268 µmol L−1 for KM ; ) were similar or higher compared with the previously reported values for temperate, boreal and arctic soils (<1–134 nmol g−1 h−1 for V max and 94 − 5110 µmol L−1 for KM ; ). It is pointed out that the kinetic parameters (V max and KM ) can vary depending on incubation conditions (i.e., temperature, moisture and substrate concentration) (Coody et al. Citation1986; Nguyen and Guckert Citation2001; van Hees et al. Citation2005a; Boddy et al. Citation2008; Hill et al. Citation2008; Schneckenberger et al. Citation2008), ecosystem and soil type (Boddy et al. Citation2008; van Hees et al. Citation2008; Fujii et al. Citation2010), soil depth (van Hees et al. Citation2008; Fujii et al. Citation2010), and microbial community, biomass, and activity (van Hees et al. Citation2005a, Citation2008). In the present study, V max values were strongly correlated with microbial biomass-C (), while KM values have no significant relationship with soil physicochemical and biological properties. Anderson and Gray (Citation1990) showed similar results to our study for the relationships between glucose mineralization kinetic parameters (V max and KM ) and microbial biomass in 16 different cropland soils. The lack of correlation between KM values and microbial biomass-C may be due to microbial community rather than biomass, as the affinity systems for substrate uptake varied with microbial species (Anderson and Gray Citation1990; Vinolas et al. Citation2001). The correlation between V max values and microbial biomass-C indicates that microbial biomass-C is an essential factor to determine the V max value in tropical and temperate forest soils under similar incubation conditions (temperature and moisture). Thus, the lower V max is considered to be related to the lower microbial biomass-C. Compared with the published data, V max values obtained in tropical forests (11 ± 1 − 152 ±18 nmol g−1 h−1; ) were similar to those of temperate and boreal forest soils (15 − 134 nmol g−1 h−1 determined at 5–10°C; ), despite the higher incubation temperature in tropical forests. Although the higher soil temperature is supposed to increase V max in tropical forests, the lower microbial biomass in tropical as opposed to temperate forests is considered to result in the similarity of V max values between tropical and temperate forests in our study.
Table 5. Soil solution concentration, kinetics and turnover of monosaccharides
Magnitude of monosaccharide mineralization relative to basal respiration
Although there are a number of C substrates available for mineralization by microorganisms in soils, monosaccharides are easily biodegradable and are probably the dominant C substrates for microbial communities (Paul and Clark Citation1996; Hill et al. Citation2008). This conclusion is supported by our data in which the mineralization rates of monosaccharides at soil solution concentrations are comparable to the CO2 rates of basal respiration (22–118%; ). These percentages are as high as those observed in the topsoils of temperate and boreal forests (19−72%; ). However, when the published data are also taken into account, the proportions of basal respiration attributable to monosaccharides ranged widely from <1 to 118% in surface soils in the tropical and other forests ( and ). According to Boddy et al. (Citation2008), the minor contribution of monosaccharides to basal respiration indicates that substrates other than monosaccharides are also the main C sources utilized in arctic tundra soils (at most 25%; Jones DL 2010, personal communication). These findings suggest that the relative importance of monosaccharides as substrates for soil microorganisms varies among ecosystem and soil types.
Turnover of monosaccharides in soil solution
Monosaccharides in soil solution are quickly mineralized due to rapid uptake and assimilation by the soil microbial community (van Hees et al. Citation2005a). The mean residence times (MRTs) for monosaccharides in the soil solution in tropical forests were calculated to be 0.4–1.9 h using the concentrations and mineralization rates of monosaccharides in the soil solution ( and ). These values indicate that monosaccharides turn over rapidly (12–60 times per day) in the surface soils. The MRTs obtained in tropical forest topsoils are very short, as reported for temperate forests by Fujii et al. (Citation2010) (0.5 − 1.0 h; ) and for temperate and arctic grasslands calculated using the double exponential kinetic model (k1 ) by Boddy et al. (Citation2007, Citation2008) (0.5–1.0 h for forests and 0.6–1.6 h for grasslands). The shorter MRTs of monosaccharides in our study support the theory that microbial biodegradation drives rapid removal of monosaccharides from the soil solution (van Hees et al. Citation2005a, Citation2008; Boddy et al. Citation2007; Hill et al. Citation2008; Fujii et al. Citation2010). Consequently, this rapid removal by the microbial community results in low concentrations in soil solution in tropical forests. In our study, the rapid turnover of monosaccharides in topsoil solution is shown to be commonly observed in tropical soils as well as temperate, boreal and arctic soils.
The kinetic parameters determined by the Michaelis-Menten equation imply the importance of monosaccharide turnover in the soil solution. According to our study ( and ), the KM values are considerably higher than the concentrations of monosaccharides in the soil solution in tropical soils. This means that the mineralization capacity of microorganisms far exceeds the rate obtained at soil solution concentrations. This is consistent with the finding that soil microorganisms are exposed to severe substrate limitation (Boddy et al. Citation2007, Citation2008; Hill et al. Citation2008; van Hees et al. Citation2008). Bengtson and Bengtsson (2007) also found that DOC production from soil organic C (SOC) seemed to be a rate-limiting step for soil respiration rates in the temperate forests. Provided that soil solution pools are maintained at a steady state, the rapid rates of monosaccharide removal from the soil solution should be matched by new inputs from exudation and turnover from roots and microorganisms, initial leaching from fresh leaves, throughfall, and extracellular solubilization of litter and SOM (Grayston et al. Citation1996; Hongve et al. Citation2000; Jones et al. Citation2003; Hill et al. Citation2008). Thus, production rates of monosaccharides (e.g., solubilization of cellulose) are considered to control the CO2 effluxes of respiration by heterotrophic microorganisms in tropical forest soils.
Conclusions
The concentrations and mineralization kinetics of monosaccharides in soil solution were studied in six tropical forest topsoils. Based on these results, the mineralization rates of monosaccharides at soil solution concentrations and their magnitude relative to basal respiration were quantified. Further, the factors controlling the mineralization kinetics of monosaccharides were examined by comparing the tropical and temperate forest soils. The kinetic parameters V max and KM varied widely with soil types. The values of V max increased with increasing microbial biomass-C in tropical and temperate soils, while the KM values had no significant correlation with soil biological or physicochemical properties. The correlation between V max values and microbial biomass-C indicates that microbial biomass-C is an essential factor regulating the V max values in tropical and temperate forest soils. The biodegradation kinetics of monosaccharides indicate that the microbial capacity of monosaccharide mineralization far exceeds its rate at soil solution concentrations in tropical forests. Monosaccharides in soil solution were rapidly mineralized, and the rates of monosaccharide mineralization at soil solution concentrations accounted for the substantial proportions of basal respiration in tropical forest soils. Probably due to rapid and continuous production and consumption, monosaccharide mineralization is shown to be a dominant fraction of basal respiration in tropical forest soils, as well as in temperate and boreal forest soils.
Acknowledgments
We thank the Radioisotope Research Center, Kyoto University, for instrumental support in the radioisotope experiments. We sincerely thank Dr. Patrick A. W. Van Hees, for teaching us the methods of measurement and analysis using 14C to determine the kinetics of monosaccharides. This work was partly supported by a short term JSPS invitation fellowship for Van Hees.
References
- Anderson , T-H and Gray , TRG . 1990 . Soil microbial carbon uptake characteristics in relation to soil management . FEMS Microbiol.Ecol. , 74 : 11 – 20 .
- Bengtson , P and Bengtsson , G . 2007 . Rapid turnover of DOC in temperate forests accounts for increased CO2 production at elevated temperatures . Ecol Lett. , 10 : 783 – 790 .
- Boddy , E , Hill , PW , Farrarb , J and Jones , DL . 2007 . Fast turnover of low molecular weight components of the dissolved organic carbon pool of temperate grassland field soils . Soil Biol. Biochem. , 39 : 827 – 835 .
- Boddy , E , Roberts , P , Hill , PW , Farrar , J and Jones , DL . 2008 . Turnover of low molecular weight dissolved organic C (DOC) and microbial C exhibit different temperature sensitivities in Arctic tundra soils . Soil Biol. Biochem. , 40 : 1557 – 1566 .
- Burney , CM and Sieburth , JMcN . 1977 . Dissolved carbohydrates in seawater. II, A spectrophotometric procedure for total carbohydrate analysis and polysaccharide estimation . Mar. Chem. , 5 : 15 – 28 .
- Coody , PN , Sommers , LE and Nelson , DW . 1986 . Kinetics of glucose uptake by soil microorganisms . Soil Biol. Biochem. , 18 : 283 – 289 .
- Derrien , D , Marol , C and Balesdent , J . 2007 . Microbial biosyntheses of individual neutral sugars among sets of substrates and soils . Geoderma , 139 : 190 – 198 .
- Fujii , K , Funakawa , S , Hayakawa , C and Kosaki , T . 2008 . Contribution of different proton sources of pedogenetic soil acidification in forested ecosystems in Japan . Geoderma , 144 : 478 – 490 .
- Fujii , K , Hayakawa , C , van Hees , PAW , Funakawa , S and Kosaki , T . 2010 . Biodegradation of low molecular weight organic compounds and their contribution to heterotrophic soil respiration in three Japanese forest soils . Plant Soil , 334 : 475 – 489 .
- Fujii , K , Hartono , A , Funakawa , S , Uemura , M and Kosaki , T . 2011 . Acidification of tropical forest soils derived from serpentine and sedimentary rocks in East Kalimantan, Indonesia . Geoderma , 160 : 311 – 323 .
- Gallant , AR . 1987 . “ Univariate nonlinear regression ” . In Nonlinear regression models , Edited by: Gallant , AR . 1 – 122 . New York : John Wiley & Sons Inc. .
- Gallet , C and Keller , C . 1999 . Phenolic composition of soil solutions: comparative study of lysimeter and centrifuge waters . Soil Biol. Biochem. , 31 : 1151 – 1160 .
- Gee , GW and Bouder , JW . 1986 . “ Particle-size analysis ” . In Methods of soil analysis Part1 Physical and Mineralogical Methods, , 2nd , Edited by: Klute , A . 383 – 411 . Madison : American Society of Agronomy Inc., Soil Science Society of America Inc. .
- Giesler , R , Lundström , US and Grip , H . 1996 . Comparison of soil solution chemistry assessment using zero-tension lysimeter or centrifugation . Eur. J. Soil Sci. , 47 : 395 – 405 .
- Grayston , SJ , Vaughan , D and Jones , D . 1996 . Rhizosphere carbon flow in trees, in comparison with annual plants: the importance of root exudation and its impact on microbial activity and nutrient availability . Appl. Soil Ecol. , 5 : 29 – 56 .
- Hill , PA , Farrar , JF and Jones , DL . 2008 . Decoupling of microbial glucose uptake and mineralization in soil . Soil Biol. Biochem. , 40 : 616 – 624 .
- Hongve , D , van Hees , PAW and Lundström , US . 2000 . Dissolved components in precipitation water percolated through forest litter . Eur. J. Soil Sci. , 51 : 667 – 677 .
- Jobbágy , EG and Jackson , RB . 2000 . The vertical distribution of soil organic carbon and its relation to climate and vegetation . Ecol. Appl. , 10 : 423 – 436 .
- Johnson , KM and Sieburth , JMcN . 1977 . Dissolved carbohydrates in seawater.I, A precise spectrophotometric analysis for monosaccharides . Mar. Chem. , 5 : 1 – 13 .
- Jones , DL . 1998 . Organic acids in the rhizosphere—a critical review . Plant Soil , 205 : 25 – 44 .
- Jones , DL , Dennis , PG , Owen , AG and van Hees , PAW . 2003 . Organic acid behavior in soils—misconceptions and knowledge gaps . Plant Soil , 248 : 31 – 41 .
- Jones , DL and Edwards , AC . 1998 . Influence of sorption on the biological utilization of two simple carbon substrates . Soil Biol. Biochem. , 30 : 1895 – 1902 .
- Kawahigashi , M , Sumida , H and Yamamoto , K . 2003 . Seasonal changes in organic compounds in soil solutions obtained from volcanic ash soils under different land uses . Geoderma , 113 : 381 – 396 .
- Kuzyakov , Y and Demin , V . 1998 . CO2 efflux by rapid decomposition of low molecular organic substances in soils . Sci. Soils , 3 : 11 – 22 .
- Kuzyakov , Y and Jones , DL . 2006 . Glucose uptake by maize roots and its transformation in the rhizosphere . Soil Biol. Biochem. , 38 : 851 – 860 .
- Marschner , B and Kalbitz , K . 2003 . Controls of bioavailability and biodegradability of dissolved organic matter in soils . Geoderma , 113 : 211 – 235 .
- Nambu , K , van Hees , PAW , Jones , DL , Vinogradoff , S and Lundström , US . 2008 . Composition of organic solutes and respiration in soils derived from alkaline and non-alkaline parent materials . Geoderma , 144 : 468 – 477 .
- Nguyen , C and Guckert , A . 2001 . Short-term utilisation of 14 C-[U] glucose by soil microorganisms in relation to carbon availability . Soil Biol. Biochem. , 33 : 53 – 60 .
- Paul , EA and Clark , FE . 1996 . “ Carbon cycling and soil organic matter ” . In Soil Microbiology And Biochemistry , Edited by: Paul , EA and Clark , FE . 129 – 155 . San Diego : Academic Press .
- Raich , JW and Schlesinger , WH . 1992 . The global carbon dioxide flux in soil respiration and its relationship to vegetation and climate . Tellus , 44B : 81 – 99 .
- Sawada , K , Funakawa , S and Kosaki , T . 2008 . Soil microorganisms have a threshold concentration of glucose to increase the ratio of respiration to assimilation . Soil Sci. Plant Nutr. , 54 : 216 – 223 .
- Schimel , DS , House , JI Hibbard , KA . 2001 . Recent patterns and mechanisms of carbon exchange by terrestrial ecosystems . Nature , 414 : 169 – 172 .
- Schneckenberger , K , Dermin , D , Stahr , K and Kuzyakov , Y . 2008 . Microbial utilization and mineralization of [14C] glucose added in six orders of concentration to soil . Soil Biol. Biochem. , 40 : 1981 – 1988 .
- Soil Survey Staff . 2006 . Keys to Soil Taxonomy, , 10th , Washington : United States Department of Agriculture Natural Resources Conservation Service .
- Strobel , BW . 2001 . Influence of vegetation on low-molecular-weight carboxylic acids in soil solution—a review . Geoderma , 99 : 169 – 198 .
- Taylor , JR . 1997 . An Introduction to Error Analysis – The Study of Uncertainties in Physical Measurements, , 2nd , California : University Science Books .
- Trouve , C , Disnar , JR , Mariotti , A and Guillet , B . 1996 . Changes in the amount and distribution of neutral monosaccharides of savanna soils after plantation of Pinus and Eucalyptus in the Congo . Eur. J. Soil Sci. , 47 : 51 – 59 .
- van Hees , PAW , Johansson , E and Jones , DL . 2008 . Dynamics of simple carbon compounds in two forest soils as revealed by soil solution concentrations and biodegradation kinetics . Plant Soil , 310 : 11 – 23 .
- van Hees , PAW , Jones , DL , Finlay , R , Godbold , DL and Lundström , US . 2005a . The carbon we do not see—the impact of low molecular weight compounds on carbon dynamics and respiration in forest soils: a review . Soil Biol. Biochem. , 37 : 1 – 13 .
- van Hees , PAW , Jones , DL and Godbold , DL . 2002 . Biodegradation of low molecular weight organic acids in coniferous forest podzolic soils . Soil Biol. Biochem. , 34 : 1261 – 1272 .
- van Hees , PAW , Jones , DL , Jentschke , G and Godbold , DL . 2005b . Organic acid concentrations in soil solution: effects of young coniferous trees and ectomycorrhizal fungi . Soil Biol. Biochem. , 37 : 771 – 776 .
- van Hees , PAW , Jones , DL , Nyberg , L , Holmström , SJM , Godbold , DL and Lundström , US . 2005c . Modelling low molecular weight organic acid dynamics in forest soils . Soil Biol. Biochem. , 37 : 517 – 531 .
- Van Hees , PAW , Vinogradoff , SI , Edwards , AC , Godbold , DL and Jones , DL . 2003 . Low molecular weight organic acid adsorption in forest soils: effects on soil solution concentrations and biodegradation rates . Soil Biol. Biochem. , 35 : 1015 – 1026 .
- Vance , ED , Brookes , PC and Jenkinson , DS . 1987 . An extraction method for measuring soil microbial biomass C . Soil Biol. Biochem. , 19 : 703 – 707 .
- Vinolas , LC , Healey , JR and Jones , DL . 2001 . Kinetics of soil microbial uptake of free amino acids . Biol. Fertil. Soils , 33 : 67 – 74 .
- Wu , J , Joergensen , RG , Pommerening , B , Chaussod , R and Brookes , PC . 1990 . Measurement of soil microbial biomass C by fumigation-extraction - an automated procedure . Soil Biol. Biochem. , 22 : 1167 – 1169 .