Abstract
A field experiment was carried out in northern Vietnam to investigate the effects of adding different additives [rice (Oriza sativa L.) straw only, or rice straw with added lime, superphosphate (SSP), urea or a mixture of selected microorganism species] on nitrogen (N) losses and nutrient concentrations in manure composts. The composts and fresh manure were applied to a three-crop per year sequence (maize–rice–rice) on a degraded soil (Plinthic Acrisol/Plinthaquult) to investigate the effects of manure type on crop yield, N uptake and fertilizer value. Total N losses during composting with SSP were 20% of initial total N, while with other additives they were 30–35%. With SSP as a compost additive, 65–85% of the initial ammonium-N (NH4-N) in the manure remained in the compost compared with 25% for microorganisms and 30% for lime. Nitrogen uptake efficiency (NUE) of fresh manure was lower than that of composted manure when applied to maize (Zea mays L.), but higher when applied to rice (Oriza sativa L.). The NUE of compost with SSP was generally higher than that of compost with straw only and lime. The mineral fertilizer equivalent (MFE) of manure types for maize decreased in the order: manure composted with SSP > manure composted with straw only and fresh manure > manure composted with lime. For rice, the corresponding order was: fresh manure > manure composted with SSP/microorganisms/urea > manure composted with lime/with straw alone. The MFE was higher when 5 tons manure ha−1 were applied than when 10 tons manure ha−1 were applied throughout the crop sequence. The residual effect of composted manures (determined in a fourth crop, with no manure applied) was generally 50% higher than that of fresh manure after one year of manure and compost application. Thus, addition of SSP during composting improved the field fertilizer value of composted pig manure the most.
Introduction
In 2006, the number of pigs in Vietnam was around 27 million and these pigs excreted around 20 million tons of solid waste (Hoang and Dao Citation2008). Solving the major problems related to management and disposal of waste from pig production is highly necessary because the waste can be a source of air pollution and a threat to aquifers and surface water (Burton and Turner Citation2003). However, it is also a valuable resource for crop production if used appropriately (Bouwman and Booij Citation1998).
Composting of manure has been proposed as one of the ways to solve the growing problem of solid waste management (Hoitink Citation2000), as the process facilitates the handling of the manure by reducing its weight, killing pathogens and stabilising the constituent nutrients and organic matter (Michel et al. Citation1996). The major disadvantages of composting are the potentially large losses of carbon (C) through microbial degradation into carbon dioxide (CO2) and eventually methane (CH4) under local anaerobic conditions, and losses of nitrogen (N), mainly as ammonia (NH3). Losses of N during composting range from 20 to 70% (Martins and Dewes Citation1992; Eghball et al. Citation1997; Osada et al. Citation2001; Sommer Citation2001). Limiting N losses during composting, and determining the exact nutrient concentration of compost and fresh manure, are necessary in order to ensure adequate nutrient supply to maximise crop yields, while avoiding over-application (Munoz et al. Citation2008). Improving manure N use efficiency is crucial because N plays a key role in both crop productivity and environmental impacts (Oenema et al. Citation2001).
Vietnamese farmers are long accustomed to using pig manure for crops (SFI Citation2005). The pig manure is either used fresh or pre-composted by various methods in order to reduce the infection risks from pathogens, the odour and the volume of pig manure, in order to reduce the labour requirements for transporting the manure to fields (Hedlund et al. Citation2003; Phuong et al. Citation2006; Vu et al. Citation2007). Solid manure is often mixed with ash, straw or other crop residues, but additives such as lime, superphosphate and urea may also be applied, and the compost heap may be left uncovered or covered with mud (Tuan et al. Citation2006; Vu et al. Citation2007). The reasons for mixing with additives are to improve compost quality (nutrient concentrations increase by mixing with urea or with superphosphate) or to kill pathogens (the effect of mixing with lime) (SFI Citation2005). Some farmers may also apply various mixtures of cultured species of bacteria (commercially produced or home-grown) to the compost, which are anticipated to increase the effectiveness of bacterial decay of organic matter, speed up the composting process, and hence reduce the required processing time in the compost heap.
The effects of application of fresh or composted manure to crop production have been reported in many studies, especially in terms of crop N use efficiency and N recovery from N manure. Munoz et al. (Citation2008) found that the first-year apparent N availability in fresh poultry manure was much higher than in composted poultry manure and that the apparent N recovery in the year following application was also higher. However, Loecke et al. (Citation2004) applied fresh and composted solid swine manure to maize and reported that the N fertilizer equivalent value of composted manure was higher than for fresh manure. Under a continental monsoon climate, Wang et al. (Citation2007) reported that overall N recovery efficiency ranged from 28% to 54% depending on N source, and that N recovery efficiency in a wet year was higher than in a dry year. Under a subtropical monsoon climate, Pan et al. (Citation2009) applied chemical fertilizer only or combined chemical fertilizer with pig manure and straw and found that N use efficiency was much higher under combined fertilization compared with inorganic fertilization only. The residual effect of manure has also been examined in many studies. Under humid temperate conditions, the effect of residual manure N in the second year has been found to be less than 10% (Jensen et al. Citation2000; Thomsen Citation2001; Sorensen and Amato Citation2002).
Degraded soil, defined as soil of light texture and poor nutrient content, is one of the main agricultural soil types in Vietnam, comprising around 1.4 million ha (approx 14% of agricultural land) (SFI Citation2002). These soils are concentrated in some provinces in the Red River delta, which is the most intensive agricultural region in terms of livestock (poultry and pig) and crop production (rice, maize and vegetable). Because of the importance of this degraded soil region for agricultural production, some studies have been conducted in the past decade to investigate the effects of fertilizers, especially organic fertilizers made from livestock wastes, on crops. Bo et al. (Citation1995) reported that the efficiency of farmyard manure (FYM) for rice yield on degraded soil was 23–49 kg rice ton−1 FYM ha−1 and recommended a dose of 10 tons FYM per rice crop. Son and Hien (Citation1995) noted that the nitrogen use efficiency of FYM for spring and summer rice was 20 and 22 kg rice kg−1 N in FYM, respectively.
However, there is a lack of up-to-date research on the interaction between actual manure management, including various compost additives, and N losses and crop fertilization value. Therefore, the objectives of the current study were to investigate the nutrient losses from manure during field composting and the nitrogen use efficiency of varying application rates of manure and composts on degraded soil. More specifically, the study examined the following questions:
1. | How do farmers’ practices of composting with common additives affect N losses and nutrient concentration in composts when applied at field scale? | ||||
2. | How do different rates of fresh manure and compost types (with different additives) affect crop yield, N uptake and fertilizer value? | ||||
3. | How large is the residual effect of fresh manure and compost type after one year of application to three preceding crops? |
Materials and Methods
Field composting
Pig manure was collected at a pig farm in Luong Phong commune, Hiep Hoa district, Bac Giang province. The farm has about 10 sows and 50 fatteners and uses commercial feeds for both types of pigs. Manure of both sows and fatteners was manually collected and kept in plastic bags for up to a week before each composting occasion. Fresh pig manure for the field experiments was collected from the same farm in a similar way in the week preceding application to the field.
The pig manure was field-composted with different additives. The mixtures were:
For composting 1 and 2 (used for winter maize (Zea mays L.) 2007 and spring rice (Oriza sativa L.) 2008):
1. | Straw only: 500 kg fresh solid manure + 25 kg rice straw (5% by weight, around 50:50 by volume) | ||||
2. | Lime: 500 kg fresh solid manure + 25 kg rice straw + 10 kg lime calcium carbonate (CaCO3) (2% by weight) | ||||
3. | SSP: 500 kg fresh solid manure + 25 kg rice straw + 25 kg single superphosphate Ca(H2PO4)2 (5% by weight) |
1. | SSP: 500 kg fresh solid manure + 25 kg rice straw + 25 kg single superphosphate | ||||
2. | Urea: 500 kg fresh solid manure + 25 kg rice straw + 10 kg urea (CO(NH2)2) (2% by weight) | ||||
3. | Microorg.: 500 kg fresh solid manure + 25 kg rice straw + 1 kg microorganism powder (0.2% by weight) + 2.5 kg lime (0.5% by weight) + 1.5 kg potassium chloride (KCl) (0.3% by weight) + 1 kg urea (0.2% by weight) + 2.5 kg superphosphate (0.5% by weight). |
The microorganism powder is a product of the Soils and Fertilizers Research Institute which is recommended as an additive for pig manure composting. The main microorganisms in the powder are Streptomyces hygroscopicus, Bacillus polyfermenticus, Bacillus subtilis and Saccharomyces cerevisiae.
The manure and additives were mixed homogeneously, placed in dome-shaped heaps on a concrete floor and covered with mud. Wet mud was collected from a nearby pond and applied to the compost heaps to an overall thickness of 2–3 cm to reduce odour and N losses by volatilization (farmers’ practice). The composting time depended on crop season and air temperature. Composting 1 and 2 (Straw, Lime and SSP treatments) lasted 55 and 111 d, respectively, and for composting 3 (SSP, Urea and Microorg. treatments) 91 d (). During composting, the temperature in each heap and ambient temperature were measured manually by thermometer at 9 am every morning.
Experimental site
The field experiment was carried out at the Midland Centre of Soils and Fertilizers Research (21°20′ N, 106°01′ E) of the Soils and Fertilizers Research Institute in Luong Phong commune, Hiep Hoa district, Bac Giang province in Northern Vietnam, 75 km northeast of Hanoi.
The climate in this region is subtropical, with a mean annual temperature of 23.5°C and a mean annual rainfall of 1620 mm, of which more than 80% occurs between May and October. Meteorological data for the research area during the field experiment were collected at a weather station 5 km from the research site ().
The soil profile of the research area was classified as a Plinthic Acrisol (FAO Citation1990) or Plinthaquult (Soil Survey Staff Citation1992). The characteristics of the basic topsoil (0–25 cm) were 2% clay (<2 µm), 70% silt (2–20 µm), 20% fine sand (20–200 µm) and 8% coarse sand (>200 µm), pH (in 1 M KCl) 5.0, total C 6.6 g kg−1, total N 1.2 g kg−1, total P 0.35 g kg−1, total K 0.58 g kg−1, Olsen-P 17 mg kg−1, 1 M NH4OAc-extractable K 4.15 mg kg−1 and CEC 2.3 cmol kg−1.
Experimental design and treatment application
The crop experiment was a completely randomised block design with 14 different treatments and 4 replicates, and the plot size was 29 m2 (5 m × 5.8 m). The treatments with different types of manure (fresh or composted manure types), different rates of manure (0, 5 or 10 tons ha−1 for each crop) and different rates of mineral fertilizer N (maize: 45, 135 or 180 kg N ha−1, rice: 0, 50 or 100 kg ha−1 for each crop) were fixed for all crops in the crop sequence (maize 2007 - spring rice 2008 - summer rice 2008). The crop calendar is shown in . After the three–crop sequence, a last crop of maize (2008) was grown without manure application to determine the residual effect compared with mineral fertilizer. Details of the fertilizer treatments and organic and mineral fertilizers are given in . The inorganic fertilizers used were urea (46% N), single superphosphate (6.9% P) and KCl (50% K). All treatments received the same amount of KCl and single superphosphate, at rates of 39 kg P ha−1 and 100 kg K ha−1 for maize and 30 kg P ha−1 and 83 kg K ha−1 for rice in the sequence.
Table 1. Mineral fertilizer nitrogen (N) and manure or compost types and rates (ha−1) applied to the different crops in the sequence
Composts and fresh manure (FM) were applied before planting (basal fertilizers) and other chemical fertilizers were applied several times during crop growth: 3 times for maize; before planting (100% P, 50% K and 30% N), at the 4–6 leaf stage (40% N) and at the 10–12 leaf stage (30% N and 50% K) and 4 times for rice; before planting (70% P and 40% K) and 10 d (40% N), 30 d (30% N) and 45 d (30% N, 30% P and 60% K) after planting.
Crop management
The crop management followed common farming practices in the research area. For maize (Zea mays L.) and rice (Oriza sativa L.) locally recommended varieties, LVN 4 and Khang Dan 18, respectively, were used.
Maize was cultivated on raised beds, 90 cm wide and 20 cm high, with 30-cm spacing between beds. The beds were established about 1–2 d prior to sowing. Composts and FM were incorporated before the beds were completed. Maize was sown in two rows (50 cm in spacing) per bed with 50 cm × 70 cm × 25 cm spacing (approx 67,000 plants per ha). During the growth of maize, water was supplied by furrow irrigation in adequate amounts.
Rice plots were flooded 5–7 d before transplanting. Composts and FM were applied one day before transplanting and mixed with the soil during the final harrowing. Rice seedlings were grown in a nursery bed for 25 d (spring rice) or 17 d (summer rice) and then transplanted in 10 cm × 20 cm spacing with 3–4 seedlings per hill (approx 500,000 plants ha−1). Water levels in the rice plots were reduced after land preparation for transplanting and soil was kept saturated for about one week after transplanting. During the growth of rice the floodwater was maintained at 5–10 cm above the soil surface by irrigation (no through-flow of water) until field drainage 10–14 d before harvest.
Weeds were controlled manually, while pests and diseases were controlled as required with recommended pesticides. At harvest, plants were manually cut at ground level and all aboveground crop residues were removed from the field as common farmers’ practice at the regions.
Sampling
Fresh manure was collected before mixing with materials of each composting batch and before applying directly to the field of each crop as described above; composted manures were taken before and after composting to determine the nutrient concentration. Three samples in each compost heap were randomly collected after compost mixing for replication.
Plant samples were taken at mid-season (except winter maize 2008), at the 10–12 leaf stage for maize and before the ear stage for rice, and at harvesting of every crop to determine the N uptake. Five plant samples were taken for maize and 10 plants for rice, and these were randomly collected in the middle of the plot to avoid boundary effects. At harvest, grain yields were determined based on a 4 m2 area in the centre of each plot.
Measurements and analyses
Fresh and composted manure: Dry matter was determined by drying at 105°C for 24 h, and ash concentration by incinerating at 600°C for 5 h. The pH of the samples mixed with distilled water (1:4 v:v) was measured by pH meter. Total N was measured by the Kjeldahl method and NH4-N by distillation of samples extracted with dilute hydrochloric acid (HCl) (0.05 N). For determination of total P and K, samples were digested with sulphuric acid (H2SO4) and nitric acid (HNO3) (1:1 v:v), while for plant-available P and K samples were extracted with dilute HCl (0.05 N). The P concentration was then measured by the Vanado-molybdophosphoric acid method (Jasko 7800 spectrophotometer - Japan) (Kovar Citation2003) and the K concentration by flame photometry (Corning 410 - UK).
Total C concentration in fresh and composted manure was calculated according to the equation:
Plant samples: Plant samples and grain materials were oven-dried (55°C) to determine dry matter (DM), and then ground to pass a 2-mm screen, and total N in the samples was measured by the Kjeldahl method.
Calculations
Total loss of weight, DM and N was calculated by losses of dry matter FDM in g, determined by the use of conservation of P (Eq. 2) according to Sommer and Dahl (Citation1999):
Nitrogen uptake efficiency (NUE) and mineral fertilizer equivalent (MFE) of the organic manure were calculated for the first three crops in the sequence by the following equations:
Based on the fourth crop (maize 2008) with only chemical fertilizer N application, the residual Nitrogen uptake efficiency (NUEresidual) of organic manure was calculated as:
Statistical analysis
Statistical analyses were carried out for the results in the following manner:
Field composting: Data were statistically analysed by one-way analysis of variance (ANOVA) (proc glm) for the effect of composting treatment on nutrient concentration in compost.
Crop experiment: Statistical analyses of data were performed by one-way ANOVA for the combined effect of manure types, and amount of manure + mineral fertilizer N (proc glm) on crop yield, crop N uptake at mid-season and at harvesting time for each crop season; and multiway ANOVA (proc mixed) for the effect of factors manure type, manure amount and mineral fertilizer N amount on crop yield, crop N uptake at mid-season and at harvesting time for each crop season using SAS 9.1 (SAS Institute Citation1988).
Results
Temperature during composting
Because of the differences in the average ambient temperature during the three composting periods (28, 17 and 27°C) and the subsequent differences in the duration of the composting, the composting process differed between compost batches, as indicated by the temperature changes during composting (). In composting 1, the temperature in the compost heaps increased relatively slowly and did not reach the peak temperature (around 45°C) before 30 d in all additive treatments (Straw, Lime and SSP). In composting 2, although the same additives were used as in composting 1, the temperature change was different. The temperature in the compost heaps was similar between additives, increased and reached a peak at 50°C after 15 d and then slowly decreased to ambient temperature at the end of composting. In composting 3, the temperature was different between additives (Urea, Microorg. and SSP). The temperature in Urea was markedly higher than in Microorg. and SSP in the initial phase, and it reached a peak (around 50°C) after 20 d and stayed around 45 to 50°C during composting. The temperature in SSP slowly increased and reached a peak (around 50°C) after 40 d. The peak temperature in Microorg. was lower than in both Urea and SSP (38°C), and it was reached after approximately 20 d of composting.
Nutrient losses during composting
The nutrient concentration in manure before and after composting is shown in . The nutrient concentration and the characteristics of composts depended on the additive used. The initial pH value (before composting) was lowest in SSP, while after composting it was significantly lower than in Lime, Urea and Microorg., by around one unit. The difference in DM between additives as well as during composting was not significant, although it increased slightly in all treatments over time. The DM remaining after composting ranged from 70 to 90% of the initial amount, being highest in SSP and lowest in Lime ().
Figure 3. Proportion of initial dry weight (DM), total nitrogen (N) and ammonium-N (NH4-N) remaining after composting (mean values; T-bars represent the standard deviation of the samples; n = 3). SSP, superphosphate.
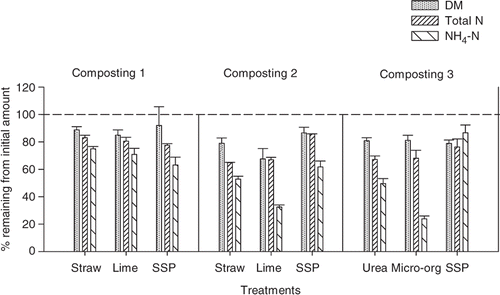
Table 2. Concentration of dry matter (DM), ash, carbon (C) and nutrients in fresh manure and composted manure in each of the three batches of composting; the values for fresh manure are averaged over all fresh manures used
The initial value of total N was naturally highest in Urea (49.9 g kg−1 DW) and it was significantly different from Microorg, Straw, Lime and SSP (around 20–30 g kg−1 DW). During composting, the decrease in total N concentration was only significant in Urea. However, after composting the total N remaining from the initial amount in Urea (60–70%) was similar to that in Microorg, Straw and Lime, but slightly lower than in SSP, which was around 80% ().
Similarly to total N, NH4-N was initially highest in Urea (21.7 g kg−1 DW) and it was also significantly different from SSP, Lime, Straw and Microorg., where NH4-N ranged from 6.1 to 11.1 g kg−1 DW. During composting, the NH4-N in Urea and Microorg. decreased significantly, while that in Lime, Straw and SSP decreased only slightly. However, after composting the amount of NH4-N compared with the initial amount was markedly different between additives. The NH4-N was highest in SSP, 65–80% of the initial value, and lowest in Microorg. (composting 3), around 25%, and Lime, around 30% (composting 2) ().
Ash, C, P and K concentration in the composts also depended on the type and amount of additive, as well as on the composting batch (). The ratio of available to total P increased after composting; available P constituted 40–70% before composting but 70–80% after composting (data not shown). The ratio of available to total K, in contrast, seemed to remain unchanged over time and was not significantly different between additives. Available K constituted around 80–95% of total K both before and after composting (data not shown). The initial C/N ratio in composts was significantly affected by additives and during composting the C/N ratio changed only slightly due to current C and N losses ().
Effects of the rates of fresh manure and compost types on crop grain yield
The application rate of manure as well as manure type (fresh manure and different composts) affected crop grain yield significantly during the crop sequence, with the effect being more marked after the first crop. However, the interaction between manure type and manure amount did not affect crop yield significantly ().
Table 3. Effects of composts and manure types, manure and mineral nitrogen (N) fertilizer amount on yield and N uptake at mid-season and harvest in the maize–rice–rice–maize crop sequence
For the first crop in the sequence (maize 2007), the effect of manure type (FM, Straw, Lime and SSP) on maize grain yield was non-significant except for the treatment with 5 tons SSP + 135 kg N ha−1, which gave the highest grain yield, 6.5 tons ha−1, compared with the same amount of FM, Straw and Lime application (). The effect on yield of increasing the amount (from 5 to 10 tons) of each manure type was small but significant in FM, Straw and Lime and non-significant in SSP. The mineral N fertilizer affected maize yield significantly (p < 0.05), with the yield of the treatment with 5 tons manure + 135 kg N ha−1 being much higher than that of the treatment with 5 tons manure + 45 kg N ha−1 and also the treatment with 10 tons manure + 45 kg N ha−1.
Figure 4. Effect of mineral fertiliser nitrogen (N), compost/manure types and compost/manure amount on crop grain yield in the crop sequence. The last graph (for maize 2008) shows the residual effect of one year of application of different compost/manure types on maize grain yield. The different letters at the top of columns represent significantly different values (P < 0.05). FM, fresh manure; SSP, superphosphate.
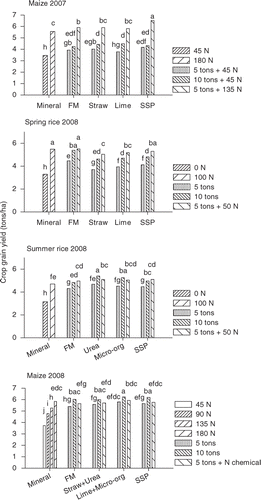
In the second crop (spring rice 2008), the effect of manure type (FM, Straw, Lime and SSP) on spring rice grain yield was highly significant (), with FM giving significantly higher yield than composted manure (Straw, Lime and SSP), but the difference in effect between compost types was not significant. With the same amount of fertilizer + manure N application, the highest spring rice yield was achieved with FM, then SSP, Lime and Straw. The mineral N fertilizer affected rice yield significantly, but the effect was reduced slightly while the effect of amount manure application increased compared with the first crop (maize 2007), because of the residual effects from previous manure application to the first maize crop ().
In the third crop (summer rice 2008), the effect of manure type (FM, Urea, Microorg. and SSP) on grain yield was significant (). With the same amount of fertilizer + manure N application, the highest rice yield was achieved in Urea, then Microorg. and SSP and FM (). The effect of amount of manure applied on rice yield increased continuously compared with the two previous crops. The yield of the treatment with 10 tons manure was significantly higher than that of the treatment with 5 tons manure and similar to that of the treatment with 5 tons manure + 50 kg N ha−1.
After one year of manure application to the three preceding crops, the last crop (maize 2008) was grown without manure application to determine the residual effect compared with mineral fertilizer. For the same amount applied, the residual effect of different composts (FM, Straw + Urea, Lime + Microorg, and SSP) had no significant effect on maize yield. Moreover, the different manure rates (total of 15 and 30 tons manure applied per plot over the three preceding crops) affected maize yield significantly (). The residual effect of mineral N was quite low because the maize yield of the previous treatments with 5 tons manure and 5 tons manure + mineral fertilizer N (more than 190 kg mineral N applied during three preceding crops) was not significantly different.
Effects of rate of fresh manure and compost type on crop N uptake
Manure type affected crop N uptake significantly in the second and third crops. Different amounts of manure affected crop N uptake significantly, except for crop N uptake at mid-season of the first and second crops. However, the interaction between manure type and manure amount had no significant effect on N uptake during the crop sequence, except N uptake at mid-season of the third crop ().
For the first crop (maize 2007), N uptake at the mid-season sampling time constituted 20–25% of total N uptake. The effect of manure type (FM, Straw, Lime and SSP) on N uptake was not significant. The effect on N uptake at harvest time when the amount of manure was increased (from 5 to 10 tons) for each manure type was only significant in Lime. Application of mineral N affected N uptake significantly. The N uptake of the treatment with 5 tons manure + 135 kg N ha−1 was much higher than that of the treatments with 5 tons manure + 45 kg N and 10 tons manure + 45 kg N ha−1 ().
Table 4. Nitrogen (N) uptake of whole aboveground plant biomass (kg ha−1) at mid-season and harvest and N use efficiency (NUE) at harvest during the maize–rice–rice–maize crop sequence for the treatments
In the second crop (spring rice 2008), N uptake at the mid-season sampling constituted around 50% of total N uptake. The effect of manure type (FM, Straw, Lime and SSP) on N uptake was highly significant, with FM being significantly higher than composted manures, but there was no significant difference between compost types (). The effect on N uptake at harvest of increasing the amount of each manure type was significant for composted manures and non-significant for FM. In contrast to the first crop (maize 2007), the N uptake of spring rice in the treatments with 5 tons manure + 50 kg N ha−1 and 10 tons manure was not significantly different.
In the third crop (summer rice 2008), N uptake at the mid-season sampling time constituted 60–70% of total N uptake at harvest. With the same amount of fertilizer + manure N application, the highest N uptake was achieved in Urea, then Microorg. and SSP and FM (). The effect of the amount (5 and 10 tons) of each manure type on N uptake was significant. Similarly to the second crop, the N uptake of the treatments with 5 tons manure + 50 kg N ha−1 and 10 tons manure was not significantly different.
In the last crop (maize 2008), there was no significant difference in residual effect of manure type on crop N uptake at the same manure application rate. The residual effect of the different manure rates (in total 15 and 30 tons manure per plot during three preceding crops) on N uptake was significant in all but the Straw only + Urea treatment (). The residual effect of mineral N did not affect N uptake of maize because the N uptake of the previous treatments with 5 tons manure and 5 tons manure + mineral fertilizer N (applying more than 190 kg mineral N during the three preceding crops) was not significantly different, except after FM application.
Nitrogen efficiency and mineral fertilizer equivalent value of manure types
In the first crop (maize 2007), the NUE was different between manures and much lower than that of the mineral fertilizer. The NUE was highest in SSP and then FM, Straw and Lime (). The NUE of the treatment with 5 tons manure seemed to be higher than that of 10 tons except for Lime (). The mineral fertilizer equivalent (MFE) was highest for 5 tons SSP, almost equivalent to mineral fertilizer N (). The MFE was lowest for 5 tons Lime compost, where the 100 kg total N present was only equivalent to 26 kg mineral fertilizer N ha−1.
Figure 5. Mineral fertiliser equivalent (MFE) of the composts and manure types applied, calculated based on nitrogen (N) uptake at harvest. FM, fresh manure; SSP, superphosphate.
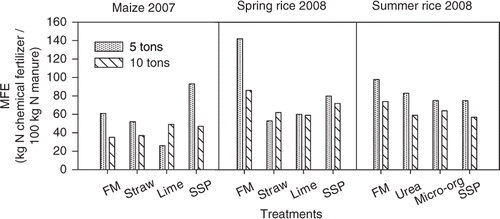
In the second crop (spring rice 2008), the NUE expressed both the efficiency of the manure applied for spring rice, and the residual effect of manure applied for the first crop (maize 2007). In contrast to the first crop, in the second crop the NUE of the FM application was highest, 0.29 kg N uptake kg−1 N applied in FM, compared with 0.19, 0.14 and 0.15 kg N uptake kg−1 N applied in SSP, Lime and Straw, respectively, when averaged over the 5 and 10 ton ha−1 application rates. The NUE of the 5 tons FM treatment was even higher than for mineral fertilizer N, 0.25 kg N uptake kg−1 N (). The MFE of FM was therefore also high; 100 kg N in FM was equivalent to 114 kg mineral fertilizer N. The MFE (kg mineral fertilizer N equivalent per 100 kg N manure) was 69 to 76 in SSP, 59 to 60 in Lime and 54 to 58 in Straw. The MFE of the 5-ton manure treatment was higher than of the 10-ton treatment in FM and SSP but lower in Straw ().
In the third crop (summer rice 2008), the plots with Straw and Lime composts were replaced by Urea and Microorg. composts but with the same respective compost application rates. The NUE therefore included not only the effect of manure applied for summer rice (FM, Urea, Microorg. and SSP), but also the cumulative effects of the two previous manure applications (for maize 2007 and spring rice 2008). In this crop, the NUE of FM application (0.33 kg N uptake kg−1 N applied) was higher than the NUE of other composts (around 0.26 kg N uptake kg−1 N applied). By N uptake calculation, the MFE of FM was highest, 86 kg mineral fertilizer N equivalent with 100 kg N in FM. The MFE values of the other composts (SSP, Urea and Microorg.) were quite similar, 65–70 kg mineral fertilizer N equivalent with 100 kg N applied in composts. Unlike the first and second crops, in the third crop the NUE and MFE of 5 tons ha−1 manure treatment were higher than those of the 10 tons ha−1 treatment ( and ).
The residual effects after one year of manure application were considerable. The highest residual MFE value was found in the SSP and Lime + Microorg. (23 to 24 kg), then in Straw only + Urea (21 kg) and lowest in FM (15 kg mineral N fertilizer equivalent per 100 kg total accumulated manure N applied), when averaged over manure application rates ().
Table 5. Residual mineral fertilizer equivalent (MFE) of different composts and manure types after application to the 3 previous crops in 1 y
Discussion
Nutrient concentration in manure and change in manure N after field composting
In this study the nutrient concentration in fresh pig manure, which was collected at a normal pig farm, was (% of wet weight): around 1% total N, 1% total P, 0.2% total K and it had a water content of 69% (). Previous data reported by Can (Citation1975), which have been used as a reference for a long time (SFI Citation2005), are lower in N and P but higher in K (0.7% total N, 0.6% total P, 1% total K and 66% water). However, recently Tuan et al. (Citation2006) found results similar to ours, with 1.6% total N, 1.0–1.5% total P, 0.4–0.5% total K and 50–55% water. The changes in the nutrient concentration of freshly excreted pig manure in Northern Vietnam from 1975 to 2006 could possibly be the result of changes in the feeding regime (Tran et al. Citation2011). Those authors showed that the main change in nutrients in fresh pig manure has been the rate of K compared with N and P. In the past the ratio of N:P:K in fresh manure was 1.0:1.0:1.5, but at present it is around 1.0:1.0:0.3. This finding is important for optimal use of pig manure for crops on degraded soil. Mussgnug et al. (Citation2006) found that K was the most limiting macronutrient in intensive rice-based cropping on these soils, and hence addition of more chemical K may be necessary to supply the crop with sufficient amounts.
The temperature curve in compost heaps with different additives was surprisingly similar, except during composting 3, where the Microorg. treatment deviated (). The maximum temperature in all compost heaps was low compared with that reported in other studies on full-scale compost heaps, which reached 60–70°C (Petersen et al. Citation1998; Sommer and Dahl Citation1999). However, in a study by Hansen et al. (Citation2006), the temperature in solid pig manure stored in a covered heap did not rise to more than 35°C, which was quite low compared with an uncovered heap in the same study. In our study, the heaps were covered with a mud plaster with rather low permeability, and this may have reduced aeration markedly, causing the rather moderate and slow temperature profile observed, similar to the Hansen et al. (Citation2006) study.
The N losses during composting were dependent on the pH value in the compost heap. The lowest total N losses were observed with SSP as the additive, where N losses constituted 20% of the initial amount, while in the other compost heaps with Microorg., Straw and Lime the total N losses were between 30 and 35% of the initial amount. The amount of NH4-N remaining in the SSP treatment was also significantly higher than for the other additives, except in composting 1 (). The lowest total N losses and highest residual NH4-N were obtained with SSP because of the lower pH value in the compost heap caused by SSP addition. Initially and after composting, the pH value in SSP was significantly (P < 0.05) lower than in other additives, by around one unit, except at the start of composting 1 (). A decrease in N losses brought about by lowering the pH value in manure has been reported in many previous studies (McCrory and Hobbs Citation2001; DeLaune et al. Citation2004; Van der Stelt et al. Citation2007; Sorensen and Eriksen Citation2009). Furthermore, the higher NH4-N concentration remaining in SSP was not only due to lower N losses, but also to higher N mineralisation compared with other additives because of the lower pH, as also observed in some recent studies (Fangueiro et al. Citation2009).
The effectiveness of compost covering in reducing N losses has been widely reported (Portejoie et al. Citation2003; Hansen et al. Citation2006; Amon et al. Citation2007). In the current study the compost heaps were densely covered with wet mud, which is a common farmer practice in Vietnam, but still, a considerable amount of N was lost. However, the N losses from initial N in manure in the study, 20% in SSP and 30–35% in Straw only and Lime, were lower than reported for small-scale batch composting with the same amount of Straw, Lime and SSP additives and aerobic conditions [25–35% with SSP, 45–55% with Straw and Lime; (Tran et al. Citation2011)]. In the present study we did not measure the amount of N lost to the atmosphere, but some of the ammonia volatilized may also have been retained as ammonium in the clay of the mud cover; this was not mixed into the compost or sampled upon termination of the composting though.
The most suitable manure and compost types for maize and rice on degraded soil in northern Vietnam
The NUE of fresh manure was higher than for composted manure for the second and third crop, but not for the first crop (). This could have been caused by the higher N mineralisation that is typical for fresh manure compared with composted manure (Chang and Janzen Citation1996; Gale et al. Citation2006; Miller et al. Citation2006). In the first season, straw was added together with the fresh manure and this may have induced N immobilisation, causing the reduced NUE for this treatment. However, using FM for crops is not recommended (SFI Citation2005), primarily because N release from fresh manure may be faster than from composted manures, and therefore field application of FM could increase the risks of N leaching and runoff from the flooded paddy soils (Miller et al. Citation2006). The residual effects to crops could then be lower than for composted manure (Munoz et al. Citation2008). In this study we did not determine N leaching or runoff from manure application, but the residual effects of composted manure after three seasons of application were somewhat higher than for FM ( and ). In the last crop (maize 2008), the NUE of the residual effect was 0.067 and 0.091 kg N uptake kg−1 N accumulated input from FM and composted manure, respectively (data calculated from ; average of 5 and 10 t ha−1 treatments), compared to the immediate NUE of fertilizer of 0.43 kg N uptake kg−1 N applied in same season (for maize 2008). The residual MFE values of composted manure were therefore on average higher than for fresh manure ().
Another consideration is that pig manure (normally slurry) in Europe and North America is applied in the field by tractor, whereas Vietnamese farmers have to collect and apply it by hand (Porphyre and Coi Citation2006). Therefore, applying FM can easily pose a potential risk to human health, because of the transmission of disease through animal manure (De et al. Citation2003).
The SSP compost resulted in slightly higher crop yield and N uptake than the other composts in the first and second seasons ( and ). This was confirmed in concurrent studies (Tran et al. Citation2011), showing higher fertilizer value of SSP compost compared with Straw only and Lime composts. The NUE of the SSP treatment was also higher than that of the Straw only or Lime treatments (). In the third crop, SSP, Urea and Microorg. composts were applied, but it was difficult to compare the effects of individual composts because of the accumulative residual effects from different manure types in previous applications (). However, the effects of different composts (SSP, Urea and Microorg) on crop yield were not significantly different ( and ). Furthermore, the residual effects of different composts after one year of application were significantly different from fresh manure, but there were no significant differences between compost types (; and ).
The most suitable manure rate for maize and rice on degraded soil in northern Vietnam
In many previous studies using farmyard manure (FYM, excreta + bedding material) for crops in Vietnam, the recommended rate for rice and maize is 10–15 tons ha−1 (Bo et al. Citation1995; Son and Hien Citation1995; SFI Citation2005). In a survey of 420 farmer households in the Red River delta, Bui (Citation1996) noted that FYM application rate varied from 9.7 to 14.9 tons ha−1.
The total N concentration in FYM on Vietnamese farms has earlier been assumed to be around 2.7 kg N ton−1 wet weight (Son and Hien Citation1995), much lower than we found in our present study, and hence application of 10 tons FYM ha−1 crop−1 year−1 will, with the earlier assumed N concentration, correspond to an estimated N application rate of 54 kg N manure N annually in a 2 crops year−1 system and 81 kg N manure in a 3 crops year−1 system. The recommended amount of manure N applied in Vietnam therefore appears to be lower than the maximum amount of animal manure (equivalent to 170 kg N ha−1) which can be applied to farmland in Europe (CEC Citation1991). However, although the actual manure application rates may not have increased over the years, the current increase in nutrient concentration, due to changes in feeding practice as discussed earlier, will result in greatly elevated manure nutrient inputs. As an example, application of 10 tons FYM ha−1 crop−1 in the current study (total manure N concentration around 10 kg N ton−1 fresh weight; ) corresponded to 300 kg N manure in the 3 crops year−1 system, which by far exceeds the maximum permissible amount for farmland in Europe. However, FYM quality varies from farm to farm and over time, and as earlier discussed, standard values may not be valid any longer, but should be reassessed for appropriate advice on manure and fertilizer application rates.
In this study two rates of fresh and composted manure (5 and 10 tons ha−1) were applied in the same plot over the three-crop sequence (maize 2007, spring rice 2008 and summer rice 2008) to determine the effect of different rates on crop production. The results ( and ) showed that the crop yield and N uptake of the treatments with 10 tons manure ha−1 were significantly higher than for the 5 tons ha−1 treatment over the crop sequence. Similar results were reported by Son and Hien (Citation1995), who examined fertilizer rates for rice in the Red River delta and found that grain yield increased with increasing FYM application. However, in the present study the MFE of 5 tons ha−1 treatment was generally higher than that of the 10 tons ha−1 treatment (). This was especially clear in the third crop (summer rice 2008). Our findings indicate that if manure is applied continuously at high rates, the NUE and agronomic efficiency of applied fertilizers and organic manures will be reduced over time, because of the cumulative residual effects on soil N mineralisation during the growing season. This confirms findings by Bo et al. (Citation1995), who reported that the NUE of FYM for rice on degraded soil decreased when N application increased.
Practical implications
This study confirmed that in field-scale composting, covering the compost heaps with mud reduces N losses, but that a significant proportion was still lost with most treatments. Adding superphosphate can minimise N losses and produce a higher concentration of plant-available NH4-N than in the initial material compared with other additives (straw only, lime, urea and Microorg. powder). However, SSP as composting additive should only be used when P demand in soil is higher than the native P concentration in the manure compost in order to avoid P overload in soil. In the current study, manure P would have been sufficient to meet crop demand.
Fresh manure is more effective than composted manure in promoting crop yield at first application, but composted manure is more sanitary to apply than fresh manure and its residual effects can be higher than those of fresh manure. When the manure is composted, less manure N will be available for application compared to applying fresh manure directly. Based on the N losses during composting (), the land area to which can be applied a certain rate of N in composted manure will be 20–35% less than if fresh manure was applied. However, in regions where intensive agricultural and livestock production is developed, farmers will often give priority to the growing problem of solid livestock waste management, and for them composting is a solution to important sanitary concerns for farm workers.
The rate of manure application to crops in Vietnam is very much based on total manure amounts in tons and advisory recommendations range from 5 to 25 tons ha−1 crop−1 year−1 depended on crops. However, the nutrient content in manures and compost may be very different (), and therefore the recommended rate of manure application to crops should ideally be based on nutrient concentration of the manure to avoid nutrient overload in soil and environmental pollution. For manure with qualities similar to those of the composts in this study, the fertilizer value for a maize-rice-rice crop sequence on degraded soil in Northern Vietnam can be improved if the manure dose does not exceed 10 tons ha−1 crop−1. With several manure applications in a year, the next crop should only be amended with manure at a lower rate or not at all, in order to exploit the residual effect of manure and limit the overload of nutrients, especially N, in soil. Lowering the manure dose will also allow the manure or compost to be used on a larger field area, hence improving the overall utilisation of its nutrient concentration, including N, P and K.
Conclusion
Total N losses during composting were lowest with an SSP additive, around 20% of initial amount) compared with 30–35% for other additives. The NH4-N remaining after composting was highest with SSP, 65–85% of the initial amount, and lowest in with Lime and Microorg. (25–30%).
The mineral fertilizer equivalent (MFE) of manure types for maize decreased in the order: manure composted with SSP > manure composted with Straw only and fresh manure > manure composted with Lime. For rice, the order was: fresh manure > manure composted with SSP/Microorg./Urea > manure composted with Lime/Straw alone. The MFE of 5 tons ha−1 manure was higher than that of 10 tons ha−1 over the crop sequence.
The residual effect after one year of application of composted manure was markedly higher than that of fresh manure.
Acknowledgments
We thank Mrs Tran Thi Thu Trang, Nguyen Thi Loc, Cao Thi Hai and Nguyen Thi Tam in the Midland Centre of Soils and Fertilizers Research (Hiep Hoa, Bac Giang) for their help and support in the field experiment. We thank Mr Nguyen Van Chien, Mrs Nguyen Thi Lan and all staff of the Central Analytical Laboratory (Soils and Fertilizers Research Institute, Hanoi, Vietnam) for technical support in analysing samples. This study was funded by the Council for Development Research (RUF) – Danish Ministry of Foreign Affairs via the research project SUSANE (Sustainable, sanitary and efficient management of animal manure for plant nutrition; Project No.J.nr.104. Dan.8.L.722).
References
- Amon , B , Kryvoruchko , V , Frohlich , M , Amon , T , Pollinger , A , Mosenbacher , I and Hausleitner , A . 2007 . Ammonia and greenhouse gas emissions from a straw flow system for fattening pigs: Housing and manure storage . Livest. Sci. , 112 : 199 – 207 .
- Bo , NV , Dinh , BD , Ba , PV , Tram , BT , Son , CK and Thuy , NT . 1995 . “ Research on nutrition for hybrid rice on degraded soils ” . In Research Results of the National Institute for Soils and Fertilizers , Edited by: NV , Bo , BD , Dinh , T , Phien , NT , Siem , TT , Son and NT , Thi . 36 – 46 . Hanoi : Agriculture Publishing House . (in Vietnamese)
- Bouwman , AF and Booij , H . 1998 . Global use and trade of feedstuffs and consequences for the nitrogen cycle . Nutr. Cycl. Agroecosyst. , 52 : 261 – 267 .
- Bui , DD . 1996 . An overview on fertilizer use in Vietnam . Vietnam Soil Sci. J. , 6 : 21 – 25 . (in Vietnamese)
- Burton , C and Turner , C . 2003 . Manure Management: Treatment Strategies for Sustainable Agriculture, , 2nd , Silsoe, Bedford, UK : Silsoe Research Institute .
- Can , LV . 1975 . Fertilizers Handbook , Hanoi, Vietnam : Liberation Publishing House . (in Vietnamese)
- CEC . 1991 . Council directive of 12 December 1991 concerning the protection of waters against pollution caused by nitrates from agricultural sources (91/ 676/ CEC) . OJEC , L375 : 1 – 8 .
- Chang , C and Janzen , HH . 1996 . Long-term fate of nitrogen from annual feedlot manure applications . J. Environ. Qual. , 25 : 785 – 790 .
- De , NV , Murrell , KD , Cong , LD , Cam , PD , Chau , LV , Toan , ND and Dalsgaard , A . 2003 . The foodborne trematode zoonoses of Vietnam . Southeast Asian J. Trop. Med. Publ. Health. , 34 : 12 – 34 .
- DeLaune , PB , Moore , PA , Daniel , TC and Lemunyon , JL . 2004 . Effect of chemical and microbial amendments on ammonia volatilization from composting poultry litter . J. Environ. Qual. , 33 : 728 – 734 .
- Eghball , B , Power , JF , Gilley , JE and Doran , JW . 1997 . Nutrient, carbon, and mass loss during composting of beef cattle feedlot manure . J. Environ. Qual. , 26 : 189 – 193 .
- Fangueiro , D , Cabral , F , Vasconcelos , E , Ribeiro , H and Coutinho , J . 2009 . “ Effect of pig slurry treatment by acidification followed by solid-liquid separation on nitrogen mineralization after application to soil ” . In Conference Proceedings of the 16th Nitrogen Workshop , Edited by: Grignani , C , Acutis , M , Zavattaro , L , Bechini , L , Bertora , C , Gallina , PM and Sacco , D . 357 – 358 . Italy : University of Torino .
- FAO 1990: FAO-UNESCO Soil Map of the World. Revised Legend. World resources report No. 60. Food and Agricultural Organization, Rome
- Gale , ES , Sullivan , DM , Cogger , CG , Bary , AI , Hemphill , DD and Myhre , EA . 2006 . Estimating plant-available nitrogen release from manures, composts, and specialty products . J. Environ. Qual. , 35 : 2321 – 2332 .
- Hansen , MN , Henriksen , K and Sommer , SG . 2006 . Observations of production and emission of greenhouse gases and ammonia during storage of solids separated from pig slurry: Effects of covering . Atmos. Environ. , 40 : 4172 – 4181 .
- Hedlund , A , Witter , E and An , BX . 2003 . Assessment of N, P and K management by nutrient balances and flows on peri-urban smallholder farms in southern Vietnam . Eur. J. Agron. , 20 : 71 – 87 .
- Hoang , KG and Dao , LH . 2008 . Livestock Development and Environment Protection , Hanoi, Vietnam : Livestock department, MARD . (in Vietnamese)
- Hoitink , HAJ . 2000 . “ Trends in treatment and utilization of solid waste through composting in the United States ” . In Proceedings of the International Composting Symposium (ISC 1999) , Edited by: Warman , PR and Taylor , BR . Vol. 1 , 1 – 13 . Nova Scotia : CBA Press Inc .
- Jensen , LS , Pedersen , IS , Hansen , TB and Nielsen , NE . 2000 . Turnover and fate of N-15-labelled cattle slurry ammonium-N applied in the autumn to winter wheat . Eur. J. Agron. , 12 : 23 – 35 .
- Kovar , JL . 2003 . “ Methods of determination of P, K, Ca, Mg and trace elements ” . In Recommended Methods of Manure Analysis , Edited by: Peter , J , Combs , S , Hoskins , B , Jarman , J , Kovar , J , Watson , M , Wolf , A and Wolf , N . 39 – 47 . Madison , WI : University of Wisconsin-Extension .
- Loecke , TD , Liebman , M , Cambardella , CA and Richard , TL . 2004 . Corn response to composting and time of application of solid swine manure . Agron. J. , 96 : 214 – 223 .
- Martins , O and Dewes , T . 1992 . Loss of nitrogenous compounds during composting of animal wastes . Bioresource Technol. , 42 : 103 – 111 .
- McCrory , DF and Hobbs , PJ . 2001 . Additives to reduce ammonia and odor emissions from livestock wastes: A review . J. Environ. Qual. , 30 : 345 – 355 .
- Michel , FC , Forney , LJ , Huang , AJF , Drew , S , Czuprenski , M , Lindeberg , JD and Reddy , CA . 1996 . Effects of turning frequency, leaves to grass mix ratio and windrow vs. pile configuration on the composting of yard trimmings . Compost Sci. Util. , 4 : 26 – 43 .
- Miller , JJ , Olson , ECS , Chanasyk , DS , Beasley , BW , Larney , FJ and Olson , BM . 2006 . Phosphorus and nitrogen in rainfall simulation runoff after fresh and composted beef cattle manure application . J. Environ. Qual. , 35 : 1279 – 1290 .
- Munoz , GR , Kelling , KA , Rylant , KE and Zhu , J . 2008 . Field evaluation of nitrogen availability from fresh and composted manure . J.Environ. Qual. , 37 : 944 – 955 .
- Mussgnug , F , Becker , M , Son , TT , Buresh , RJ and Vlek , PLG . 2006 . Yield gaps and nutrient balances in intensive, rice-based cropping systems on degraded soils in the Red River Delta of Vietnam . Field Crop Res. , 98 : 127 – 140 .
- Oenema , O , Bannink , A , Sommer , SG and Velthof , GL . 2001 . “ Gaseous nitrogen emissions from livestock farming systems ” . In Nitrogen in the Environment: Sources, Problems and Management , Edited by: Follett , RF and Hatfield , JL . 255 – 289 . Amsterdam : Elsevier Science .
- Osada , T , Sommer , SG , Dahl , P and Rom , HB . 2001 . Gaseous emission and changes in nutrient composition during deep litter composting . Acta Agr. Scand. B-S P. , 51 : 137 – 142 .
- Pan , G , Zhou , P , Li , Z , Smith , P , Li , L , Qiu , D , Zhang , X , Xu , X , Shen , S and Chen , X . 2009 . Combined inorganic/organic fertilization enhances N efficiency and increases rice productivity through organic carbon accumulation in a rice paddy from the Tai Lake region, China . Agr. Ecosyst. Environ. , 131 : 274 – 280 .
- Petersen , SO , Lind , AM and Sommer , SG . 1998 . Nitrogen and organic matter losses during storage of cattle and pig manure . J. Agr. Sci. , 130 : 69 – 79 .
- Phuong , ND , Tuan , VD and Toan , TD . 2006 . “ Farmers’ practices in organic and inorganic fertilization on crops, trees and vegetables ” . In Pig Production Development, Animal Waste Management and Environment Protection: A Case Study in Thai Binh Province, Northern Vietnam , Edited by: Porphyre , V and Coi , NQ . 145 – 162 . France : PRISE Publication .
- Porphyre , V and Coi , NQ . 2006 . Pig Production Development, Animal Waste Management and Environment Protection: A Case Study in Thai Binh Province, Northern Vietnam , France : PRISE Publication .
- Portejoie , S , Martinez , J , Guiziou , F and Coste , CM . 2003 . Effect of covering pig slurry stores on the ammonia emission processes . Bioresource Technol. , 87 : 199 – 207 .
- SAS Institute . 1988 . SAS/STAT User's Guide, Release 6.03 Edition , Cary , NC : SAS Institute Inc .
- SFI (Soils and Fertilizers Institute) . 2002 . “ The grey degraded soils ” . In The Basic Information of Main Soil Units of Vietnam , Edited by: Bo , NV , Dinh , BD , Duc , HQ , Hien , BH , Loc , DT , Phien , T and Ty , NV . 76 – 83 . Hanoi : Ministry of Agriculture and Rural Development (MARD) .
- SFI (Soils and Fertilizers Institute) . 2005 . Fertilizers Handbook , Hanoi : Agriculture Publishing House . (in Vietnamese)
- Schulte , EE and Hopkins , BG . 1996 . “ Estimation of organic matter by weight loss-on-ignition ” . In Soil Organic Matter: Analyses and Interpretation, SSSA Spec. Publ. 46 , Edited by: Magdoff , FR , Tabatabai , MA and Hanlon , EA . 21 – 31 . Madison , WI : SSSA .
- Soil Survey Staff 1992: Keys to Soil Taxonomy, 5th ed., SMSS Technical Monograph No. 19. United States Department of Agriculture. Pocahontas Press Inc, Virginia, USA
- Sommer , SG . 2001 . Effect of composting on nutrient loss and nitrogen availability of cattle deep litter . Eur. J. Agron. , 14 : 123 – 133 .
- Sommer , SG and Dahl , P . 1999 . Nutrient and carbon balance during the composting of deep litter . J. Agr. Eng. Res. , 74 : 145 – 153 .
- Son , TT and Hien , DV . 1995 . “ Determining the fertilizer rates for high yield and economic efficiency of rice on alluvial soil in the red river delta ” . In Limited Nutrient Factors of Crop Yield and Strategy for Crop Nutrient Management in Vietnam (Report of the KN-01-10 Project) , Edited by: BD , Dinh . 33 – 47 . Hanoi : Agriculture Publishing House . (in Vietnamese)
- Sorensen , P and Amato , M . 2002 . Remineralisation and residual effects of N after application of pig slurry to soil . Eur. J. Agron. , 16 : 81 – 95 .
- Sorensen , P and Eriksen , J . 2009 . Effects of slurry acidification with sulphuric acid combined with aeration on the turnover and plant availability of nitrogen . Agr. Ecosyst. Environ. , 131 : 240 – 246 .
- Thomsen , IK . 2001 . Recovery of nitrogen from composted and anaerobically stored manure labelled with N-15 . Eur. J. Agron. , 15 : 31 – 41 .
- Tran , MT , Vu , TKV , Sommer , SG and Jensen , LS . 2011 . Nitrogen turnover and loss during storage of slurry and composting of solid manure under typical Vietnamese farming condition . J. Agr. Sci. , 149 : 285 – 296 .
- Tuan , VD , Porphyre , V , Farinet , JL and Toan , TD . 2006 . “ Composition of animal manure and co-products ” . In Pig Production Development, Animal Waste Management and Environment Protection: A Case Study in Thai Binh Province, Northern Vietnam , Edited by: Porphyre , V and Coi , NQ . 127 – 143 . France : PRISE Publication .
- Van der Stelt , B , Temminghoff , EJM , Van Vliet , PCJ and Van Riemsdijk , WH . 2007 . Volatilization of ammonia from manure as affected by manure additives, temperature and mixing . Bioresource Technol. , 98 : 3449 – 3455 .
- Vu , TKV , Tran , MT and Dang , TTS . 2007 . A survey of manure management on pig farms in Northern Vietnam . Livest. Sci. , 112 : 288 – 297 .
- Wang , XB , Cai , DX , Hoogmoed , WB , Perdok , UD and Oenema , O . 2007 . Crop residue, manure and fertilizer in dryland maize under reduced tillage in northern China. I: Grain yields and nutrient use efficiencies . Nutr. Cycl. Agroecosyst. , 79 : 1 – 16 .