Abstract
Treatment of animal and food wastes using a methane fermentation technique is drawing considerable public attention as a suitable option for the utilization of biomass resources. The application of a fermentation byproduct (methane fermentation digested liquid) as an agricultural fertilizer has been investigated. Determining the appropriate timing required for applying digested liquid on a rice (Oryza sativa L.) paddy plot is important. The concentrations of soil nitrogen (N) components and rice yield should be considered because digested liquid contains both inorganic and organic N. This study compares the N transformation and the rice yield and growth at different application times over a period of 3 y. The effects of the timing of basal application on soil N were different and the timing that maximized the rice yield was different in each year. Days before ponding (DBP) affected soil N before mid-summer drainage, and rice growth rates at the panicle formation stage and the ear emergence stage. The effects of DBP disappeared before harvest. The results indicated that sufficient potentially mineralizable N existed regardless of DBP, and the effect of DBP lessened after the mid-summer drainage, which coincides with the period when N uptake is most active.
Introduction
Methane (CH4) fermentation is an effective option to increase the utilization of biomass resources such as animal and food wastes. However, the high cost of treating the fermentation byproduct (methane fermentation digested liquid, hereinafter called digested liquid) is a significant problem and complicates the sustainable management of a methane fermentation facility. A potential solution is to use digested liquid as an agricultural fertilizer because it contains high levels of available crop nutrients. Moreover, the use of digested liquid can indirectly reduce greenhouse gas emissions because of a decreased need for inorganic fertilizers (Arthurson Citation2009). In Japan, it is anticipated that digested liquid would be applied to rice paddy fields because, except for the Hokkaido region, there are only a few upland fields to which large quantities of digested liquid can be applied.
Digested liquid differs from chemical and other organic fertilizers in that it contains both organic and inorganic nitrogen (N). However, the ratio varies depending on the methane fermentation plant. Therefore, application methods that take the specific mineralization rate of organic N in digested liquid into account are required (Möller and Stinner Citation2009).
When organic matter such as animal manure and green manure is applied to the soil, meeting the crop's inorganic N requirements is an important consideration (Schröder Citation2005). In order to meet N requirements, appropriate timing, rates, and placement heights (distance from the soil surface) for the application of manure have been developed (Thomsen Citation2001; Hartl et al. Citation2003; Schils and Kok Citation2003). Regulating the application rate enables the synchronization of N requirements and supply. However, rice cultivation presents a particular problem because an overabundant supply of N makes rice plants grow considerably and lodge before harvest (Schröder et al. Citation2000). Changing the application placement can increase the efficient use of N by reducing ammonia volatilization; therefore, it is effective for the application of digested liquid to upland fields (Fujikawa and Nakaumra Citation2010; Tokuda et al. Citation2010). For rice cultivation, yields were maximized when the field was ploughed immediately after the application of digested liquid to the soil surface by a spreading method (Watanabe et al. Citation2011). Many studies which considered timing attempted to establish the effects of N fertilization using organic manure applied before cultivation (Kankanen et al. Citation1998; Dahlin et al. Citation2005; Schröder Citation2005). Numerous studies have considered both rate and timing (Adeli et al. Citation2003; Read et al. Citation2008; Gomez-Munoz et al. Citation2011). Kurdali and Al-Shamma’a (Citation2010) showed that, to obtain greater dry matter and N yield for sorghum, the appropriate timing for the incorporation of green manure appeared to be 30 d before planting rather than 15 d before planting.
Little is known about the effect of timing of application of organic matter on rice plants. In paddy fields, the soil is saturated and remains under anaerobic conditions for a long time, and N dynamics are presumed to be distinctly different from grasslands or other arable land that does not require puddling (Win et al. Citation2009). Hara et al. (Citation2009) examined the effect of the timing (0, 2, and 4 weeks before puddling) of the application of cattle manure compost on N use efficiency in forage rice, and demonstrated that cattle manure compost could be an effective fertilizer if it is applied close to the time of puddling. Witt et al. (Citation1998) compared the incorporation of rice straw 63 d before transplanting to incorporation 14 d before transplanting in a double cropping system. They concluded that, compared to a later incorporation date, plant N accumulation and grain yield were 20–24% greater when the residue was incorporated earlier and without any application of chemical N fertilizer. The mineralization rate of digested liquid would be different from that of animal or green manure. Kamioka and Kamewada (Citation2011) investigated the growth and yield of rice (Oryza sativa L. cv Koshihikari) with basal application of digested liquid at different timings. They concluded that, as compared to the later application (2 d before flooding), the earlier application of digested liquid (9 d before flooding) led to a decrease of up to 40% in N uptake by the plants, and suggested that it was due to nitrification under aerobic conditions and denitrification after flooding. In order to explain why earlier application of digested liquid lessened N efficiency, a specific investigation of soil N is needed. Furthermore, there are few studies about application timing of digested liquid into paddy soil, especially into actual paddy fields.
In this study, we examine the N transformations in paddy soil and rice yields. Over a period of 3 y, we undertook 3 applications of digested liquid at different times, i.e., 2, 1, and 0 weeks, before ponding.
Materials and methods
Field experiments
The field experiments were carried out from May 2008 to September 2010 on 4 paddy plots (plot numbers 1–4) located in the Hidokoro district, Yagi town, Nantan city, Kyoto Prefecture, Japan (135°54′E, 35°09′N, and 120 m above sea level). The variety of the cultivated rice was “Oryza sativa L. cv Kinuhikari.” The livestock and food waste were treated using a methane fermentation process for biogas production at a treatment plant (Yagi Bio-Ecology Center: YBEC) that has been in operation since 1998.
shows the application dates and crop management components. The timing of basal applications of digested liquid is denoted as DBP (days before ponding). Three variants of DBP were undertaken to compare the effect on soil N dynamics and crop yield. is the schema of experimental plots. Each plot was divided into halves (11 m × 100 m) and digested liquid was applied to each sub-plot at different DBP. Each plot was divided into sub-plots to determine the influence of soil characteristics.
Table 1. Applications of digested liquid and cultivation practices
In 2008, basal application was conducted by the injection method. A slurry injector was used to furrow the soil and inject digested liquid at the furrow depth (15–20 cm below the surface). In 2009 and 2010, digested liquid was spread on the soil surface by a slurry spreader, and tillage followed immediately. Each amount of the total N was 5.66 g N m−2 in basal and additional applications and the total amount of applied N over the crop duration was 11.32 g N m−2, which is the conventional amount of N applied to the “Kinuhikari” variety in this prefecture. Digested liquid was applied to each sub-plot at the rate of about 2.3 t. After the surface was drained, an additional application was poured onto the paddy plots using a septic truck. Digested liquid was delivered from YBEC on the day of application. The average N content of digested liquid is shown in (methods of analysis are described later). The mid-summer drainage was conducted and the soil remained unsaturated for the first half of July 2008 and 2009 and the last half of July 2010.
Table 2. Components of methane fermentation liquid for application (mg NL−1)
The daily mean value for air temperature, rainfall, and daylight was recorded for the investigation period. These measurements were taken by the Sonobe meteorological station (135°27’E, 35°06’N, and 134 m above sea level), which was at a distance of 41 km from the experimental site. The data were obtained from the Japan Meteorological Agency's database. shows the relevant meteorological changes during the crop seasons for 3 y.
Soil sampling and analyses
Soil samples were collected at 2 points in each sub-plot at a depth of 0–10 cm (). Sampling started each year just before basal application, and additional samples were taken immediately after application and after ponding and puddling and transplanting. Sampling continued at intervals of 1 or 2 weeks until harvest. Until they were analyzed, samples were stored at about −8°C.
The dissolved total N (DTN) in the soil was analyzed according to the method described by Jones and Willett (Citation2006). Ten grams of unsieved field-moist soil was shaken in 225-cm3 glass bottles with 100 mL of 100 g L−1 potassium chloride (KCl) [1:10 weight:volume (w:v) soil-to-solution ratio] for 1 h. After shaking, the soil extracts were refrigerated for more than 30 min and the supernatant was then put through a cellulose acetate membrane filter (0.45 µm; Advantec Toyo Kaisha Ltd., Tokyo, Japan). The filtrate was stored in polypropylene bottles in a refrigerator. DTN was determined after potassium persulfate (K2S2O8) oxidation of the extract (Cabrera and Beare Citation1993; Doyle et al.
Citation2004). Ammonium-N (-N) was determined by the indophenol method. Both DTN and
-N were analyzed colorimetrically with a spectrophotometer (UVmini-1240; Shimadzu, Kyoto, Japan). The concentration of nitrate-N (
-N) (including nitrogen dioxide-N,
-N) was determined by cadmium-copper column reduction with flow injection analysis (FIA; F · I · A Instruments Co. Ltd., Tokyo, Japan). The dissolved organic N (DON) was determined by the difference between the DTN and the inorganic N (sum of
-N and
-N) in the KCl extract.
A 200-mL sample of digested liquid was filtered through a 1 -µm cellulose filter paper (No.5C; Advantec Toyo Kaisha Ltd., Tokyo, Japan). The total N in the unfiltered sample and the total dissolved N in the filtrate were determined colorimetrically after K2S2O8 oxidation. The -N content in the filtrate was also determined colorimetrically.
-N and
-N in the filtrate were analyzed by ion-chromatography (CDD-6A; Shimadzu, Kyoto, Japan). The digested liquid's DON is the difference between the total dissolved N and the inorganic N in the filtrate.
Growth and yield of rice
Six stocks of rice samples were collected near the soil sampling points at the panicle formation stage (PFS) and the ear emergence stage (EES) in each year, as shown in . The dry weight and the N concentration of the whole plant above ground were measured. The N content of the rice plant was determined by multiplying the dry weight by the N concentration. Taste was measured by a rice grain taste analyzer (RCTA11A, Satake Co., Hiroshima, Japan) (Ryu et al. Citation2010). The yield was determined as the average of dry weight of unhulled rice in each 5 m × 5 m block, including the soil sampling point, by a head-feeding combine (VY50, Mitsubishi Agricultural Machinery Co. Ltd., Shimane, Japan) equipped with a GPS and a grain flow-rate sensor (Iida et al. Citation2009).
Statistical analyses
Statistical analyses were conducted using JMP 9.0.2 (SAS Institute, Cary, NC, USA). A one-way analysis of variance (ANOVA) was conducted for the DBP effect in each plot on each observation date. Tukey's procedures were then used to test all pairwise comparisons (maintaining the α level at 0.05). Correlations between soil N concentration on each investigation date and rice variables (dry weight, N content, yield, and taste) were determined by Pearson's correlation analyses.
Results
N concentrations in soil before transplanting
shows temporal changes in DON, NH4 + -N, and -N concentrations in the soil at different DBP from basal application to transplanting for 3 crop y.
Figure 3. Temporal changes in nitrogen (N) concentrations in soil before transplanting. The symbols and error bars indicate the average and standard deviation of sub-plots (2 sampling points), respectively. DAP; days after ponding; DBP, days before ponding; TP, transplanting; DON, dissolved organic nitrogen. *Denotes significant differences in each sub-plot.
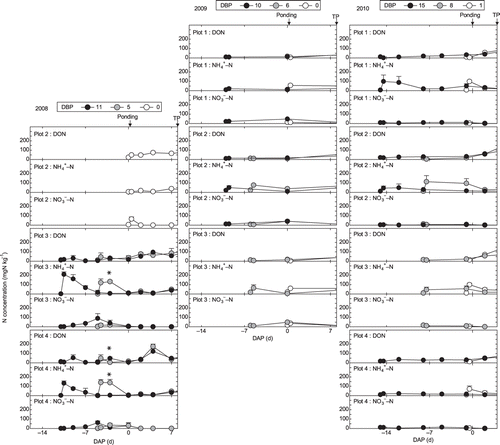
Before the applications of digested liquid, all N contents were low. Immediately after the basal applications, -N increased markedly, especially at 11 DBP, in 2008. The increase in
-N decreased gradually before ponding. DON in the soil did not increase immediately after the basal applications despite a high concentration in digested liquid (). In 2008, DON increased slightly 1 or 2 d after the application and declined before ponding. In 2009 and 2010, no increase in DON was observed during this period.
-N showed a slow peak accompanied by
-N reduction one week after the basal applications in 2008 (plots 3 and 4) and 2009 (plots 2 and 3). However, in 2010, there was no change in
-N even when
-N decreased. In 2008, there were significant differences in
-N between the sub-plots in plots 3 and 4 and in DON in plot 4. In all years,
-N and
-N fell to low level after ponding and DON increased slightly in some sub-plots between ponding and transplanting.
There was no rainfall before the first basal application (11 DBP) in 2008; rainfall of over 20 mm d−1 fell 7 DBP and 4 DBP, as shown in . In 2009, rainfall of over 20 mm d−1 fell 1 d after the first basal application. In 2010, rainfall of less than 10 mm d−1 fell several times before ponding. The daily mean air temperature ranged between 10 and 20°C from basal application to transplanting in each year.
N concentrations in soil from transplanting to additional application
illustrates DON and -N concentrations in the soil during the period from transplanting to additional applications of digested liquid for 3 crop y; the figure does not include
-N concentrations. Because of saturated soil conditions,
-N concentrations were at almost zero level during this period. DON increased gradually up to 100–250 mg N kg−1 after transplanting and maintained this level during the irrigation period. The rates at which DON reached its maximum level were different among years, plots, and DBPs. The average rate at which DON increased in 2008 was lower than those for 2009 and 2010. However, in 2008, the rate increase for DON continued until mid-summer drainage. By comparison, in 2009 and 2010, among the increasing rates of DON for different DBP in each sub-plot, the shorter DBP had higher rates. However, DBP did not affect the maximum level of DON. Significant differences in DON between sub-plots appeared only in plots 1 and 2 in 2009 and plot 2 in 2010. DON declined during mid-summer drainage in most sub-plots, except plot 2 in 2008 (DBP = 0). In 2008 and 2009, the DON values in the mid-summer drainage period were in the range of 50–100 mg N kg−1 but fell to around 10 mg N kg−1 in 2010.
Figure 4. Temporal changes in nitrogen (N) concentrations in soil from transplanting to additional application. The symbols and error bars indicate the average and standard deviation of sub-plots (2 sampling points), respectively. DAP, days after ponding; DBP, days before ponding; TP, transplanting; AA, additional application; PFS, panicle formation stage; DON, dissolved organic nitrogen. *Denotes significant differences in each sub-plot.
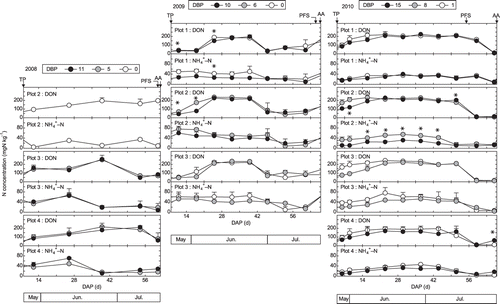
Similar to DON, -N also increased after transplanting and maintained a constant level until the additional applications. In 2009, the maximum value of
-N occurred just after transplanting and then decreased gradually. In 2010, the maximum value of
-N appeared 2 or 3 weeks after transplanting. In both years and in all sub-plots,
-N levels were higher for shorter DBP. The difference in
-N level relative to DBP was especially significant for plot 2 in 2010.
In all years, the daily mean air temperature tended to rise between transplanting and the additional application (). Similarly, rainfall of over 20 mm d−1 occurred frequently in all years. In particular, there was considerable rainfall and fewer sunlight hours in 2010.
N concentrations in soil from additional application to harvest
shows the temporal changes in DON and -N between the additional applications of digested liquid and harvest for 3 crop y. Despite dry conditions, there was a very low concentration of
-N (data not shown). After the additional application, DON demonstrated a peak in some sub-plots. However, other sub-plots did not show any peak. The significant differences in DON among sub-plots appeared in plot 4 in 2008, plots 2 and 3 in 2009, and plot 3 in 2010. After the additional applications,
-N increased in all years. However, 5 d after the additional applications,
-N declined to a low level. The
-N level ranged from 0.5 to 27.0 mg N kg−1 for 3 y. There were no significant differences in
-N between the sub-plots during this period.
Figure 5. Temporal changes in nitrogen (N) concentrations in soil from additional application to harvest. The symbols and error bars indicate the average and standard deviation of sub-plots (2 sampling points), respectively. DAP, days after ponding; DBP, days before ponding; AA, additional application; EES, ear emergence stage; DON, dissolved organic nitrogen. *Denotes significant differences in each sub-plot.
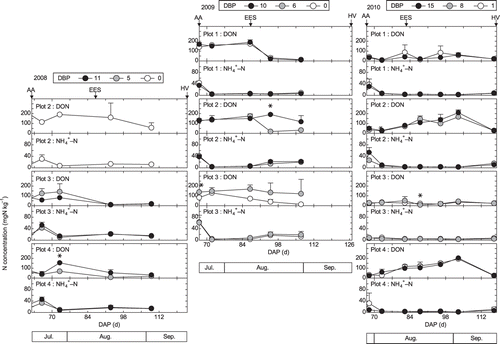
Rice yield, taste, and growth
shows the average and standard deviation in yield, taste, and growth (dry weight, N concentration, and N content) at each sub-plot for 3 crop y. These results of yield were at a level similar to that of the data with the unhulled rice yield (820 ± 17 g m−2, 2 DBP) in a plot to which chemical fertilizer was applied in 2008 (Watanabe et al. Citation2011). The maximum values of rice yield were obtained at 0 DBP in 2008, 6 DBP in 2009, and 8 DBP in 2010. Relative to DBP, significant differences occurred in rice growth at PFS in plot 3 and at EES in plot 4 in 2008, and in yield in plot 1 and in dry weight at PFS in plot 2 in 2009. In most plots, the average value of rice yield, dry weight, and N content were frequently higher for sub-plots with shorter DBP.
Figure 6. The average values (2 sampling points) of rice (Oryza sativa L.) yield, taste, dry weight, nitrogen (N) concentration, and N content at sub-plots. PFS, panicle formation stage; EES, ear emergence stage; DBP, days before ponding. The error bar indicates standard deviation. The numbers in the figure denote DBP. *Denotes the significance level P < 0.05; **denotes P < 0.01. The black bars indicate that at the same plot in the same year, the values for shorter DBP are smaller than those for longer DBP. The gray bars indicate the reverse, i.e., values for shorter DBP are greater than those for longer DBP.
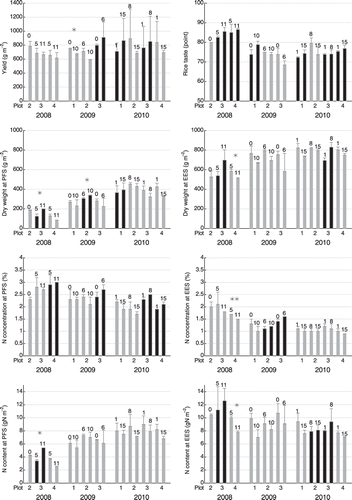
Discussion
N transformation at each stage
It was expected that the -N in digested liquid would be utilized by plant uptake immediately after transplanting. In fact, despite the increase in
-N after the basal applications,
-N declined gradually before ponding, especially for longer DBP, and therefore,
-N was not available for the rice seedlings. In 2008 and 2009,
-N rose at the same time as
-N decreased to almost zero. This suggests that nitrification occurred. This result corresponds to the suggestion by Kamioka and Kamewada (Citation2011). In upland fields to which digested liquid was applied, nitrification occurred predominantly immediately after application (Fujikawa and Nakamura Citation2010). Moreover, the fact that the decline in
-N was larger than the increase in
-N suggests that other reactions depressed both
-N and
-N. For example, it is likely that immobilization and volatilization of
-N and leaching of
-N were stimulated. Witt et al. (Citation1998) showed that early residue incorporation (63 d before transplanting) in paddy soil caused
-N accumulation in the fallow period and
-N leaching after heavy rainfall. Volatilization of N when manure was applied before transplanting or seeding was also reported by Adeli et al. (Citation2003). It is well known that
-N in digested liquid volatilizes under aerobic conditions (Möller and Stinner Citation2009; Tokuda et al.
Citation2010). In this study, digested liquid was applied by the injecting method or spreading method with ploughing in order to prevent volatilization of
-N. However, in 2010, nitrification was not active. Since there were few differences in meteorological conditions (rainfall and air temperature) before the ponding among the 3 y, we could not determine why nitrification was inert in 2010. In 2009 and 2010, DON did not increase. This suggests that immobilization, i.e., the accumulation of inorganic N in soil microbes smaller than 0.45 µm, barely occurred. It has been reported that when soil microbes have high metabolic activity, larger microbes may uptake inorganic N after the application of organic matter to dry soil (Bowden and Hann Citation1996; Dahlin et al.
Citation2005). Consequently, it is possible that DON did not increase and high molecular organic N increased through the immobilization process.
The decrease in -N after ponding in some sub-plots suggests that
-N in the surface layer (10–20 cm) diluted and diffused into deeper layers because of ponding and puddling with irrigation water.
DON increased 1 week after ponding. It is generally thought that mineralization of organic N to -N is accelerated by ponding. The increase in
-N in paddy soil after ponding or flooding has been reported in other investigations (Witt et al.
Citation2000; Unger et al.
Citation2010). Only a few studies have measured DON in paddy soil. Witt et al. (Citation1998) showed that after transplanting, DON increased and remained at a constant level. Half of DON in arable soil consists of hydrolysable amino sugars, heterocyclic-N bases, amino compounds, and free amino acids (Murphy et al.
Citation2000). It may be presumed that decomposition of larger-sized organic N elevated DON concentration. Li et al. (Citation2010) indicated that submergence periods in rice cultivation enhanced solubilization of organic matter and depolymerization of high molecular organic compounds, which also increased dissolved organic matter (DOM) content in paddy soils. Moreover, Unger et al. (Citation2010) showed that the concentration of phenolic compounds increased during the flooding period, and Olk et al. (Citation2006) concluded that the accumulation of phenolic lignin residues in the soluble organic matter (SOM) is a frequent characteristic of anaerobic agroecosystems.
DON and -N decreased during mid-summer drainage. A decline in
-N has also been reported by Witt et al. (Citation1998). This study explained that the lack of increase in extractable
-N resulted from rapid N uptake by the rice crop. The DON decrease implies that soil oxidation stimulated microbial metabolism and the immobilization rate of DON exceeded the decomposition rate.
-N increased temporally after additional applications and disappeared after 5 d. After PFS, plant growth was significant.
-N in soil is supposed to be absorbed by rice plants. The level of DON was not constant after additional applications. This suggests that each plot was irrigated intermittently at different intervals and the soil was repeatedly subjected to saturated and unsaturated conditions. Therefore, the balance of each reaction (immobilization, decomposition, mineralization, nitrification, and denitrification) fluctuated at each plot.
Immediately before harvest, both DON and -N showed low levels for 3 y (except for DON of 10 DBP in plot 2 and 6 DBP in plot 3 in 2009). This suggests that the available DON and
-N had been utilized. Moreover, low concentration of
-N indicates that nitrification did not seem to occur despite the aerobic conditions.
Effect of DBP on soil N
From ponding to mid-summer drainage, the rate of DON accumulation increased and -N showed higher levels at the sub-plots where the basal applications were later (DBP was shorter). Witt et al. (Citation1998) demonstrated that the magnitude of
-N increase after flooding was influenced by the time of residue incorporation. In their study,
-N was 33% greater 4 d after transplanting when residues were incorporated earlier rather than later in the period before transplanting. This result is not in agreement with our results. Our results indicate that, when digested liquid was applied earlier, N transformed to more recalcitrant phases because of immobilization during the aerobic period and needed more time to convert to DON (Kirchmann and Lundvall Citation1993). It is reasonable to conclude that the effect of DBP on soil N weakened gradually after ponding. This is based on our results, which show DON at consistent levels before the mid-summer drainage and that
-N decreased to the same level after mid-summer drainage in each plot. Following additional applications, significant differences were obtained in only 4 cases (DON in plot 4 in 2008, in plots 2 and 3 in 2009, and in plot 3 in 2010). The differences in sampling points were larger than those for DBP. Therefore, it is thought that the effects of DBP on soil N were not significant during this period.
In this study, the effects of longer DBP (for example, 1 or 2 months before ponding) were not considered. However, there were indications that the soil N levels were lower when DBP was longer because of increased immobilization and losses by leaching, denitrification, and volatilization in the aerobic condition. Although more studies are required to establish the most appropriate timing of basal application, this study indicated that the effect of DBP on soil N concentrations was not significant for DBP < 2 weeks.
Effect of DBP on rice yield, taste, and growth
Significant differences resulting from DBP in rice yield appeared only in plot 1 in 2009. Rice growth (dry weight, N concentration, and N content) was significantly different only at PFS and EES in 2008 and at PFS in 2009 (). There were no significant differences in most plots; however, the average growth tended to increase earlier in sub-plots with shorter DBP. This result corresponds to the change in soil N concentration immediately after ponding. Therefore, it is possible that rice growth was influenced by DBP through the change in soil N. As time passed, the differences in soil N relative to DBP decreased and the effect of DBP on rice growth also decreased.
From the fact that there were few significant differences in rice yield and taste, it is presumed that the effects of DBP decreased before harvest. This result indicates that, regardless of different DBP, rice plants were consistently able to absorb soil N when they were most active and needed N in large quantities (approximately after PFS) because high molecular organic N had decomposed prior to this period.
Correlations between soil N concentration and yield, taste, and growth of rice
Yield, N concentration, dry weight, and N content of rice (rice variables) were significantly different with respect to the timing of application in only a few cases in 2008 and 2009. In addition, averages for each rice variable were different in each year. However, rice variables such as N content in rice plants might be influenced by temporal changes in soil N. and show the correlation coefficients between rice variables and soil N concentration (DON and -N) on each sampling date (DAP). There were significant correlations between
-N at early stages (DAP 0 to 51) and rice variables at PFS.
-N at the same stage showed significant correlation with rice variables at EES in 2008 and 2009. DON correlated to rice growth variables at EES and yield in 2008 (). However there were few comparable correlations in 2009 and 2010. These data suggest that, in 2008,
-N in soil before PFS influenced rice growth at PFS and EES and DON before and after PFS affected rice growth at EES and yield. It is likely that, in 2009 and 2010,
-N levels before PFS also influenced rice growth at PFS and EES; on the other hand, DON did not correlate with rice growth at all in 2010.
Figure 7. The correlations between the dissolved organic nitrogen (DON) concentration and rice yield in 2008 at days after ponding (DAP) = 0, 61, and 66.
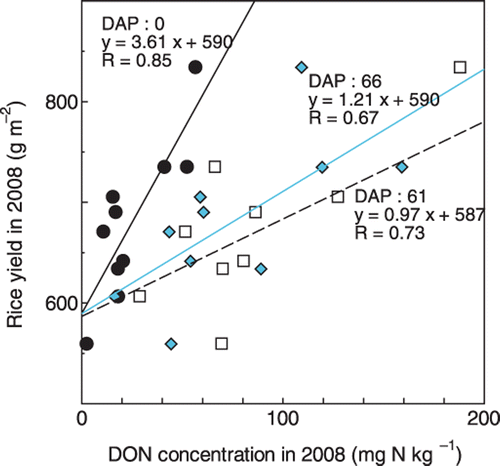
Table 3. Correlation coefficients between rice variables and soil dissolved organic nitrogen (DON) concentrations in soil on all investigation dates
Table 4. Correlation coefficients between rice variables and soil ammonium-nitrogen (
-N) concentrations in soil on all investigation dates
Conclusions
The effect of the timing of basal application of digested liquid on soil N concentration and rice yield and growth was investigated at 4 paddy plots for 3 consecutive y from 2008 to 2010. The effects of the timing of basal application on soil N were different in each year and the timing that maximized the rice yield was also different in each year.
DBP influenced soil N at early stages and soil N showed higher levels when DBP was shorter. The effect of DBP on soil N decreased gradually before mid-summer drainage. The effect of DBP on rice growth (dry weight, N concentration, and N content) showed significant differences in 2008. However, the average values for rice growth were larger for shorter DBP as well as for soil N at early stages. Despite some significant correlations between soil N (-N in 3 years and DON in 2008) and rice growth (dry weight, N concentration, and N content at PFS and EES), there were no significant correlations between soil N and rice yield. This result indicates that the influence of DBP on early stage soil N and rice growth gradually lessened and did not show significant differences. This may be because the potentially mineralizable N, which was almost the same regardless of DBP, had decomposed to DON before the period when the rice plants’ N uptake was active.
These results indicate that the basal application of digested liquid to paddy plots can be conducted from 0 to 15 DBP without decrease in rice yield. Through these results, overconcentration of application timing can be avoided. In most regions that have biogas plants and need to distribute digested liquid to paddy fields, it is a crucial issue to arrange application plans. Therefore, this conclusion must support their planning processes.
Acknowledgments
This study was supported by a grant-in-aid for scientific research from the Japan Society for the Promotion of Science, No. 22-4403.
References
- Adeli , A , Varco , JJ and Rowe , DE . 2003 . Swine effluent irrigation rate and timing effects on bermudagrass growth, nitrogen and phosphorus utilization, and residual soil nitrogen . J. Environ. Qual. , 32 : 681 – 686 .
- Arthurson , V . 2009 . Closing the global energy and nutrient cycles through application of biogas residue to agricultural land - Potential benefits and drawbacks . Energies , 2 : 226 – 242 .
- Bowden , W and Hann , M . 1996 . The availability of nitrogen following topsoil application of liquid digested sludge . Nutr. Cycl. Agroecosyst. , 47 : 167 – 172 .
- Cabrera , ML and Beare , MH . 1993 . Alkaline persulfate oxidation for determining total nitrogen in microbial biomass extracts . Soil Sci. Soc. Am. J. , 57 : 1007 – 1012 .
- Dahlin , S , Kirchmann , H , Katterer , T , Gunnarsson , S and Bergstrom , L . 2005 . Possibilities for improving nitrogen use from organic materials in agricultural cropping systems . Ambio , 34 : 288 – 295 .
- Doyle , A , Weintraub , MN and Schimel , JP . 2004 . Persulfate digestion and simultaneous colorimetric analysis of carbon and nitrogen in soil extracts . Soil Sci. Soc. Am. J. , 676 : 669 – 676 .
- Fujikawa , T and Nakamura , M . 2010 . Influence of application method and rate of digested liquid from methane fermentation on N2O gas emission and plant growth . Jpn. J. Soil Sci. Plant Nutr. , 81 : 240 – 247 . (in Japanese with English abstract)
- Gomez-Munoz , B , Hatch , DJ , Bol , R , Dixon , ER and Garcia-Ruiz , R . 2011 . Gross and net rates of nitrogen mineralisation in soil amended with composted olive mill pomace . Rapid Comm. Mass Spectrom. , 25 : 1472 – 1478 .
- Hara , Y , Tsuchiya , K and Nakano , K . 2009 . Effects of the stage of maturity and timing of application of cattle manure compost on the nitrogen fertilizer effect in forage rice cultivation . Jpn. J. Soil Sci. Plant Nutr. , 80 : 241 – 249 . (in Japanese with English abstract)
- Hartl , W , Putz , B and Erhart , E . 2003 . Influence of rates and timing of biowaste compost application on rye yield and soil nitrate levels . Eur. J. Soil Biol. , 39 : 129 – 139 .
- Iida , M , Ohdoi , K , Ryu , C and Umeda , M . 2009 . Basal application of methane fermentation digested liquid using a slurry injector . J. Jpn. Soc. Agric. Mach. , 71 : 81 – 87 . (in Japanese with English abstract)
- Jones , D and Willett , V . 2006 . Experimental evaluation of methods to quantify dissolved organic nitrogen (DON) and dissolved organic carbon (DOC) in soil . Soil Biol. Biochem. , 38 : 991 – 999 .
- Kamioka , H and Kamewada , K . 2011 . Effect of anaerobically digested cattle slurry as basal application on paddy rice Koshihikari . Jpn. J. Soil Sci. Plant Nutr. , 82 : 31 – 40 . (in Japanese with English abstract)
- Kankanen , H , Kangas , A , Mela , T , Nikunen , U , Tuuri , H and Vuorinen , M . 1998 . Timing incorporation of different green manure crops to minimize the risk of nitrogen leaching . Agric. Food Sci. Finl. , 7 : 553 – 567 .
- Kirchmann , H and Lundvall , A . 1993 . Relationship between N immobilization and volatile fatty acids in soil after application of pig and cattle slurry . Biol. Fertil. Soils , 15 : 161 – 164 .
- Kurdali , F and Al-Shamma’a , M . 2010 . Growth and nitrogen uptake in sorghum plants manured with Leucaena leucocephala leaves as affected by nitrogen rate and time of application . Comm. Soil Sci. Plant Anal. , 41 : 308 – 319 .
- Li , ZP , Han , CW and Han , FX . 2010 . Organic C and N mineralization as affected by dissolved organic matter in paddy soils of subtropical China . Geoderma , 157 : 206 – 213 .
- Möller , K and Stinner , W . 2009 . Effects of different manuring systems with and without biogas digestion on soil mineral nitrogen content and on gaseous nitrogen losses (ammonia, nitrous oxides) . Eur. J. Agron. , 30 : 1 – 16 .
- Murphy , DV , Macdonald , AJ , Stockdale , EA , Goulding , KWT , Fortune , S , Gaunt , JL , Poulton , PR , Wakefield , JA , Webster , CP and Wilmer , WS . 2000 . Soluble organic nitrogen in agricultural soils . Biol. Fertil. Soils , 30 : 374 – 387 .
- Olk , DC , Cassman , KG , Schmidt-Rohr , K , Anders , MM , Mao , J-D and Deenik , JL . 2006 . Chemical stabilization of soil organic nitrogen by phenolic lignin residues in anaerobic agroecosystems . Soil Biol. Biochem. , 38 : 3303 – 3312 .
- Read , JJ , Brink , GE , Adeli , A and McGowen , SL . 2008 . Swine effluent application timing and rate affect nitrogen use efficiency in common bermudagrass . J. Environ. Qual. , 37(SUPPL. 5) : S180 – S189 .
- Ryu , C , Suguri , M , Iida , M and Umeda , M . 2010 . Investigation into possible use of methane fermentation digested sludge as liquid fertilizer for paddy fields . Eng. Agric. Environ. Food , 3 : 32 – 37 .
- Schils , RLM and Kok , I . 2003 . Effects of cattle slurry manure management on grass yield . Neth. J. Agric. Sci. , 51 : 41 – 65 .
- Schröder , J . 2005 . Revisiting the agronomic benefits of manure: A correct assessment and exploitation of its fertilizer value spares the environment . Bioresour. Technol. , 96 : 253 – 261 .
- Schröder , J , Neetesona , JJ , Oenemaa , O and Struik , PC . 2000 . Does the crop or the soil indicate how to save nitrogen in maize production? Reviewing the state of the art . Field Crops Res. , 66 : 151 – 164 .
- Thomsen , IK . 2001 . Recovery of nitrogen from composted and anaerobically stored manure labelled with 15N . Eur. J. Agron. , 15 : 31 – 41 .
- Tokuda , S , Tanaka , Y , Higashio , H , Murakami , K , Aizawa , S , Uragami , A and Kunihisa , M . 2010 . Method for application of anaerobically digested cattle slurry to the field cultivation of cabbage . Jpn. J. Soil Sci. Plant Nutr. , 81 : 105 – 111 . (in Japanese with English abstract)
- Unger , IM , Muzika , R-M and Motavalli , PP . 2010 . The effect of flooding and residue incorporation on soil chemistry, germination and seedling growth . Environ. Exp. Bot. , 69 : 113 – 120 .
- Watanabe , S , Nakamura , K , Ryu , C , Iida , M and Kawashima , S . 2011 . Effects of different application methods of methane fermentation digested liquid into the paddy plot on soil nitrogen behavior and rice yield . IDRE Journal , 274 : 17 – 26 . (in Japanese with English abstract)
- Win , KT , Toyota , K , Motobayashi , T and Hosomi , M . 2009 . Suppression of ammonia volatilization from a paddy soil fertilized with anaerobically digested cattle slurry by wood vinegar application and floodwater management . Soil Sci. Plant Nutr. , 55 : 190 – 202 .
- Witt , C , Biker , U , Galicia , CC and Ottow , JCG . 2000 . Dynamics of soil microbial biomass and nitrogen availability in a flooded rice soil amended with different C and N sources . Biol. Fertil. Soils , 30 : 520 – 527 .
- Witt , C , Cassman , KG , Ottow , JCG and Biker , U . 1998 . Soil microbial biomass and nitrogen supply in an irrigated lowland rice soil as affected by crop rotation and residue management . Biol. Fertil. Soils , 28 : 71 – 80 .