Abstract
Tulip (Tulipa gesneriana, cultivar Oxford) bulbs were planted in October and grown in culture solution in a glasshouse under natural conditions. One group was cultivated in a solution containing nitrogen (N) (+N treatment: nitrate-N (N) 12.0, ammonium-N (
−N) 3.0 mg L−1). Another group was cultivated in a nitrogen-free solution (−N treatment). In the first experiment, the transverse accumulation of free amino acids in the cortex and stele of the tulip roots was measured. In the cortex, the total concentration of free amino acids amounted to 39.4 mg N g−1 dry weight (DW) in the +N treatment, but only 5.3 mg N g−1 DW in the −N treatment. In the stele, the concentration was 14.4 and 5.8 mg N g−1 DW, respectively. Glutamine was the predominant amino acid in the cortex in the +N treatment, reaching 18.1 mg N g−1 DW. The concentrations of asparagine and arginine were also higher after the +N than −N treatment. On the other hand, the concentration of 4-methyleneglutamine was not so different between the treatments in either the cortex or stele. In the second experiment, the accumulation of free amino acids at five vertical positions from the base to root tip was analyzed. The total concentration of free amino acids was not so different among the positions. According to statistics, the concentrations of glutamine and asparagine were relatively constant between the +/−N treatments. In the −N treatment, the concentration of 4-methyleneglutamine gradually decreased from the base toward the root tip, and it tended to be higher in the −N treatment than that in the +N treatment.
Introduction
In higher plants, roots have two essential roles (Fitter Citation2002): 1) to absorb water and mineral nutrients from soil, and 2) to sustain the above-ground parts as an anchorage to support canopy structure and radiation interception. Root systems have many important additional functions: the storage of nutrients such as carbohydrate, nitrogen and minerals, the synthesis of phytohormones and growth regulators, as well as propagation and dispersal. Furthermore, the roots interact with soil microorganisms, such as rhizobia and mycorrhizae. The growth and development of root systems involve complex interactions between both the soil environment and the shoots (Marschner Citation1995). The species-specific architecture of roots may vary depending on environmental factors where the plants grow (Zhang et al. Citation2007).
Tulip (Tulipa gesneriana) bulbs are planted in October or November in Japan. The fibrous adventitious roots grow rapidly from the basalplate, which is a stunted stem at the bottom of the bulb connecting the roots, shoot and scales. A tulip bulb has about 100 roots with the same diameter from base to root tip, about 0.5–1 mm (). The roots do not branch into lateral roots, and no root hairs are usually observed. The shoot sprouts in March and flowers in April or May. Then a few new bulblets grow and accumulate starch and storage proteins, while the shoots senesce. The bulbs are harvested in June, and stored in dry conditions during summer.
Figure 1. A photograph of tulip (Tulipa gesneriana) roots cultivated in culture solution during the flowering stage in early May.
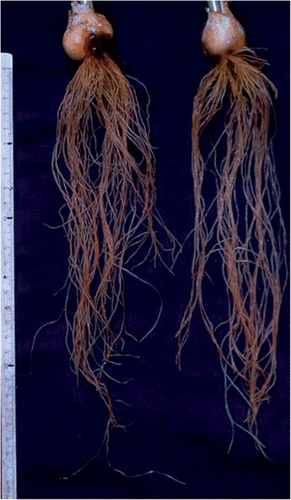
Tulip plants require definite “warm–cold–warm” temperature changes during their life cycle (Rees Citation1966; De Hertogh et al. Citation1983). Flower initiation and development occur in the harvested bulb under high temperature conditions in summer. Subsequent cold temperatures in winter are necessary to allow normal shoot development and flowering after sprouting in spring. With the forcing technique, tulip bulbs are exposed to artificial warm–cold–warm environmental conditions. The forced flowers can then be marketed from December to March after artificial cold storage for about two months in summer. Without a cold period, tulip plants exhibit an unusual dwarfed shape with a very short flower stalk and leaves, and this sometimes causes the flowers to abort (Ohyama et al. Citation1996). Tulip bulb scales contain carbohydrates as well as nitrogen (N), phosphorus (P), potassium (K) and other minerals. Cold temperature promotes the conversion of starch to sugars such as sucrose and fructans, and of storage proteins to free amino acids, like glutamine (Gln, C5H10N2O3) (Ohyama et al. Citation1988b, c; Komiyama et al. Citation1997). A deficiency of N in bulbs results in inferior plant growth and flower quality, and Baba et al. (Citation1979) recommended that the N concentration based on dry weight (DW) in tulip bulbs be higher than 1.2% for the forced cultivation of cut flowers.
Baba and Ikarashi (Citation1967, Citation1968) discovered that absorbed N was highly accumulated in tulip roots in winter, at the level of up to 8–9% based on DW. The N accumulated in tulip roots in winter can be used to support the rapid growth of the shoot and flower stalk after sprouting, supplementing the N in bulb scales, and plays an important role in improving flower quality and yield and the quality of daughter bulbs (Ohyama et al. Citation1988e).
A horizontal split-root experiment was conducted to investigate the movement of the accumulated N among individual roots through the basalplate (Kitajima et al. Citation1991). The root system was separated into two half-bunches of roots in two trays in which one bunch of roots was in an 15N-labeled solution containing nitrate and ammonium, and the other half was soaked in either a non-labeled N solution or −N solution. When 15N-labeled nitrate and ammonium were supplied from one side of the roots, 15N mainly accumulated in the roots in direct contact with 15N, and no 15N was detected in the other roots in either the non-labeled N or −N solution. This indicated that the N absorbed in one root is accumulated independently and is not easily transported to other roots in winter.
A vertical split-root experiment was also performed, to examine whether the transport of N between the upper and lower parts of one root occurs or not. Most of the absorbed 15N remained in the root which was in direct contact with the 15N solution, and was not easily moved to other parts (Komiyama et al. Citation2003). The result showed that N accumulated in the part of the root where N was absorbed. The result also showed that nitrate and ammonium absorption activity were similar between the upper and lower halves of tulip roots.
In this study, the site of N accumulation in tulip roots in autumn and winter was investigated in detail. First, the transverse accumulation of amino acids was analyzed by separating the cortex and stele. Second, the whole root was separated into five segments of equivalent length along vertical positions. We focused on the accumulation of amino acids, especially Gln and 4-methyleneglutamine (4-MeGln, C6H10N2O3). 4-MeGln is a rare amino acid, found only in groundnut (Arachis hypogaea) (Done and Fowden Citation1952) and tulip (Zacharius et al. Citation1954; Fowden and Steward Citation1957). The amino acid 4-MeGln has been detected in every part of tulip plants (i.e., bulb scales, roots, basalplate, shoots, leaves, stems and flowers) (Ohyama et al. Citation1988f). An extraordinarily high concentration of 4-MeGln was found in leaves (20 mg N g−1 DW) and roots (17 mg N g−1 DW) after sprouting (Ohyama et al. Citation1985, Citation1986, Citation1988f; Hayashi et al. Citation1987). Some 22 cultivars of tulip analyzed showed that the 4-MeGln concentrations in leaves and stems (flower stalks) were positively correlated with the concentration of soluble N, suggesting 4-MeGln to be a temporary N storage compound in vegetative organs after sprouting (Ohyama et al. Citation1988f). After sprouting, the organic acid 2-oxo-4-mehtyl-3-pentene-1,5-dioic acid (OMPD) has been observed in tulip plants and is estimated to be a degradation product of 4-MeGln releasing two N molecules. (Ohyama et al. Citation1988a).
Materials and Methods
Plant cultivation method
Tulip (Tulipa gesneriana, cultivar Oxford) bulbs were planted on October 27, 2010, and grown in tap water for the first two weeks. Then +N and −N treatments were started to elucidate the site where free amino acids accumulate in tulip roots during winter.
The composition of the culture solution was as described previously (Ohyama et al.
Citation1988a). One group of plants was cultivated in a solution containing nitrogen with the following composition in mg L−1: ammonium nitrate (NH4NO3), 17.1; calcium nitrate (Ca(NO3)2 · 4H2O), 76.0; calcium chloride (CaCl2 · 2H2O), 26.1; sodium biphosphate (Na2HPO4 · 12H2O), 30.2; potassium sulfate (K2SO4), 33.5; magnesium sulfate (MgSO4 · 7H2O), 76.1; iron sulfate (FeSO4 · 7H2O), 9.96; ethylenediaminetetraacetic acid · disodium (EDTA · 2Na), 13.32; perboric acid (H3BO4), 1.14; copper sulfate (CuSO4 · 5H2O), 0.012; zinc sulfate (ZnSO4 · 7H2O), 0.022; manganese sulfate (MnSO4 · 5H2O), 0.219; ammonium molybdate (VI) ((NH4)6Mo7O24 · 4H2O), 0.018 (+N treatment; nitrate-N ( − N) 12, ammonium-N (
−N) 3 mg N L−1). Another group (−N treatment) was cultivated in a solution of the same composition but without NH4NO3 and Ca(NO3)2 · 4H2O. Twenty bulbs were planted in a plastic box (41.0 cm length × 31.0 cm width × 20.5 cm depth) containing 26 L of culture solution. The culture solution was changed every week, and not aerated. Tulip plants were harvested on November 25 for Experiment 1 and December 21 for Experiment 2. Root primordia had already formed in the basalplate before planting. In addition, without the occurrence of lateral roots, the root number is already decided at the planting and was not different between +N/−N treatments. In the period of this experiment, the thickness, length and weight of roots were also the same between the plants grown in +/−N treatments (data not shown).
Experiment 1: Transverse accumulation of amino acids in cortex and stele
The roots of the tulip plants cultivated with +N or −N treatment were cut into segments of about 3 cm, and the cortex, including epidermis, was separated from the stele by pulling both sides of the root section after the central part of the cortex was nicked around the stele by a scalpel. After tulip roots were separated into the stele and cortex, they were freeze-dried and ground into a fine powder. They were extracted in 1 mL of 80% ethanol. The extract was separated by centrifugation at 10,000 g for 15 min. The supernatant (80% ethanol soluble fraction) was used for the amino acid analysis.
The total free amino acid concentration was measured by the ninhydrin method. The 80% ethanol extract (2.5 µL) was placed into a 1.5-mL tube, and 75 µL of citrate buffer [0.29 M citric acid and 0.53 M sodium hydroxide (NaOH)] was added. Then, 60 µL of ninhydrin reaction solution (ninhydrin, 958 mg; ascorbic acid, 33.4 mg; deionized water, 3.2 mL; methoxy ethanol, 100 mL) was added and vortexed. After 20 min of heating at 100°C, 150 µL of 60% ethanol was added and vortexed. Samples were cooled to room temperature. The absorbance at 570 nm was measured by a plate reader (Thermo Scientific Multiskan Spectrum), and the total concentration of free amino acids was calculated using a standard solution containing 5 mM Gln and 5 mM asparagine.
The concentration of each amino acid was analyzed by ultra performance liquid chromatgraphy (UPLC) (Waters, ACQUITY UPLC). First, 0.5 mL of ethanol extract was placed in a 1.5-mL microtube and evaporated dry using the rotary vacuum evaporation system. Then, 0.5 mL of pure water was added to the test tube and sonicated to dissolve free amino acids. The solution was separated by centrifugation at 10,000 g and at 4°C. The supernatant was put into another microtube. The sample solution was kept at −20°C until the analysis. A standard solution was prepared for the calibration of amino acids based on the retention time and area of the chromatogram. First, 10 µL of the standard solution and 70 µL of borate buffer were mixed well. Then, 20 µL of reagent 2 A (Waters) was added, and immediately mixed well. The solution was heated at 55°C in a heating aluminum block for 10 min. The standard solution for UPLC was transferred into a sample vial of the UPLC autosampler. The sample solution was prepared similarly to the standard solution: 10 µL of sample solution, 5 µL of 5 mM α-aminobutyric acid (AABA) solution and 55 µL of borate buffer were mixed well, 30 µL of reagent 2 A was added, and the mixture was immediately mixed well. Afterwards, it was prepared the same way as the amino acid standard solution.
Experiment 2: Vertical accumulation of amino acids in tulip roots
The tulip root system was cut into five pieces of equivalent length from root base to tip. About 10 fibrous roots with the same length were bundled and cut into five parts, “a”, “b”, “c”, “d” and “e,” from the basal part to root tip (). The root segments were freeze-dried and extracted with 80% ethanol, and the total concentration of free amino acids and each amino acid was analyzed by the ninhydrin method and by UPLC, respectively, the same way as in Experiment 1.
All results of experiments confirmed a significant difference by Tukey-test with significance at the level of P < 0.05. Different letters above bars in the figures indicate the data are significantly different at the level of P < 0.05.
Results
Experiment 1: Transverse accumulation in the cortex and stele
In Experiment 1, the amino acids in whole roots, the cortex and the stele of tulips cultivated with +N and −N treatments were measured to investigate the site of transverse accumulation. The tulip roots were separated into cortex and stele by hand.
First, the total concentration of free amino acids in the whole root, cortex and stele was measured by the ninhydrin method (). The concentration in the whole root amounted to 27.7 mg N g−1 DW in the +N treatment and 5.1 mg N g−1 DW in the −N treatment. In the cortex, the concentration was significantly high, 39.4 mg N g−1 DW, in the +N treatment, but only 5.3 mg N g−1 DW in the −N treatment. In the stele, the concentration was 14.4 and 5.8 mg N g−1 DW in the +N and −N treatments, respectively. When N was supplied to the culture solution, the concentration of free amino acids increased about eight-fold in the cortex. On the other hand, in the stele, it increased only about two-fold.
Figure 3. Comparison of the total concentration of free amino acids in whole roots, cortex and stele of tulips (Tulipa gesneriana) cultivated with −N and +N treatments. Error bars show standard error (n = 4). Different letters above bars in each part indicate a significant difference at the level of P < 0.05. N, nitrogen; DW, dry weight.
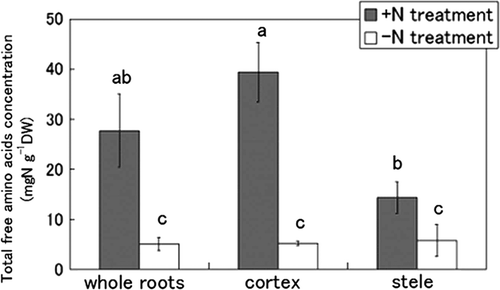
Gln was the predominant amino acid in the +N treatment, its concentration was much higher in both the cortex and stele (). The concentrations of Asn and arginine (Arg, C6H14N4O2) were also higher in the cortex and stele following +N treatment. The Gln concentration in the cortex in the +N treatment reached 18.1 mg N g−1 DW but was much lower in the stele, at 5.5 mg N g−1 DW. The Gln concentration in the −N treatment was low at 0.3 mg N g−1 DW in both the cortex and stele. The concentration of Asn and Arg in the cortex amounted to 2.2 and 2.1 mg N g−1 DW respectively, in the +N treatment. The accumulations of Asn and Arg in the stele were less than half those in the cortex. On the other hand, in the −N treatment, Asn and Arg concentrations in both the cortex and stele were much lower, at just about 0.1–0.2 mg N g−1 DW.
Figure 4. Comparison of predominant amino acids in whole roots, cortex and stele of tulips (Tulipa gesneriana) cultivated with −N and +N treatments. (a) glutamine (Gln), (b) asparagine (Asn), (c) arginine (Arg), (d) 4-methyleneglutamine (4-MeGln). Error bars show standard error (n = 4). Different letters above bars in each part indicate a significant difference at the level of P < 0.05. N, nitrogen; DW, dry weight.
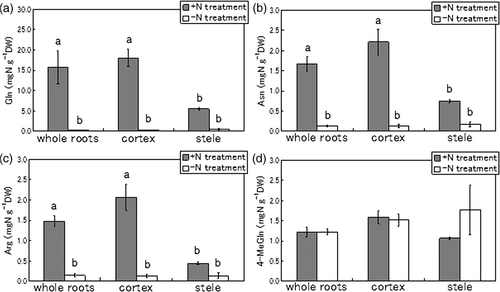
The concentration of 4-MeGln in the cortex was slightly less than that of Asn or Arg, at about 1.6 mg N g−1 DW, in the +N treatment. However, in the −N treatment, the concentration of 4-MeGln was much higher than those of Gln, Asn and Arg, about 1.5 mg N g−1 DW, almost the same level as in the +N treatment. The concentration of 4-MeGln in the stele was 1.8 mg N g−1 DW in the −N treatment and 1.1 mg N g−1 DW in the +N treatment, different from Gln, Asn and Arg.
show the ratios of the concentration of each amino acid upon +N/−N treatment in the cortex (a) and stele (b). c and 5d show ratios of the concentration of each amino acid in cortex/stele on +N treatment (c) and −N treatment (d). In , high ratios (+N/−N), over 10, were observed for Gln, Asn and Arg, and similar trends, more than 1 but less than 10, were found for 4-aminobutyric acid (GABA), ammonia (NH3), glycine (Gly), proline (Pro) and glutamic acid (Glu). However, the ratios of 4-MeGln and alanine (Ala) were about 1, which means that levels were the same in the −N and +N treatments. The +N/−N ratios of Gln, Asn, Arg and GABA tended to be higher in the stele, but lower than 1 for 4-MeGln (). The cortex/stele ratios in the +N treatment () show the greater accumulation of Arg, Glu, Gln, Asn in the cortex than in the stele. The cortex/stele ratios in the −N treatment () are lower than 1 for all the amino acids analyzed.
Figure 5. Ratios of the concentration of each amino acid. (a) Ratio of +N/−N in cortex, (b) ratio of +N/−N in stele, (c) ratio of cortex/stele on +N treatment, (d) ratio of cortex/stele on −N treatment. N, nitrogen; Gln, glutamine; Asn, asparagine; Arg, arginine; GABA, Gamma-Amino Butyric acid; NH3, ammonia; Gly, glycine; Pro, proline; Glu, glutamic acid; 4-MeGln, 4-methyleneglutamine; Ala, alanine.
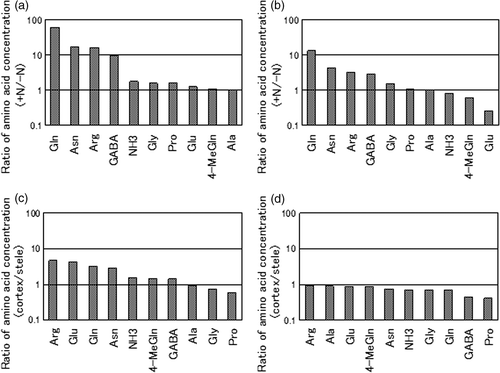
Experiment 2: Vertical accumulation of amino acids in tulip roots
In Experiment 2, we investigated where the free amino acids accumulate by examining five vertical positions in tulip roots cultivated with +N or −N treatment. Measurements of total free amino acid concentrations did not show much difference in the vertical position of roots (). In the +N treatment, the concentration of free amino acids was around 30–40 mg N g−1 DW, about 7 times higher than in the −N treatment (5 mg N g−1 DW) at all the positions. There was no significant difference among the sites in the concentration of amino acids for either the +N or −N treatment.
Figure 6. Comparison of the free amino acid concentration at five vertical positions in tulip (Tulipa gesneriana) roots cultivated with −N and +N treatments. The five parts of the roots were named “a”, “b”, “c”, “d” and “e,” from basalplate to root tip. Error bars show standard error (n = 4). Different letters above bars in each part indicate a significant difference at the level of P < 0.05. N, nitrogen; DW, dry weight.
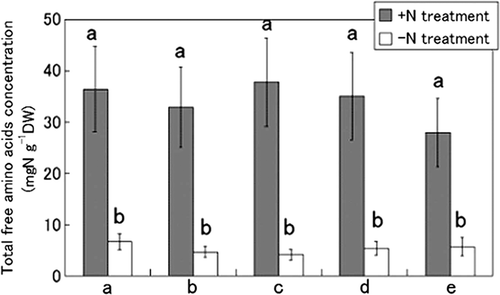
Gln was the most dominant amino acid in tulip roots in the +N treatment (). The concentration was over 20–30 mg N g−1 DW. On the other hand, in the −N treatment, the Gln concentration was significantly lower, at around 1.0 mg N g−1 DW. The concentration of Asn in the +N treatment was less than 3 mg N g−1 DW except for “a”, the basal position, where it was about 3.1 mg N g−1 DW. In contrast, the concentration of Asn in the −N treatment was around 0.5 mg N g−1 DW, except at “e” where it was 1.3 mg N g−1 DW. The concentration of Arg was relatively low for both +N and −N treatments as compared with that of Gln or Asn. Unlike Gln, Asn, and Arg concentrations, the concentration of 4-MeGln was higher in the −N treatment than +N treatment, and decreased from the basal part (“a”) toward the root tip (“e”).
Figure 7. Comparison of predominant amino acids at five vertical positions in tulip roots cultivated with −N and +N treatments. The five parts of roots were named “a”, “b”, “c”, “d” and “e” from basalplate to root tip. (a) glutamine (Gln), (b) asparagine (Asn), (c) arginine (Arg), (d) 4-methyleneglutamine (4-MeGln) Errors bar show standard error (n = 4). Different letters above bars in each part indicate a significant difference at the level of P < 0.05. N, nitrogen; DW, dry weight.
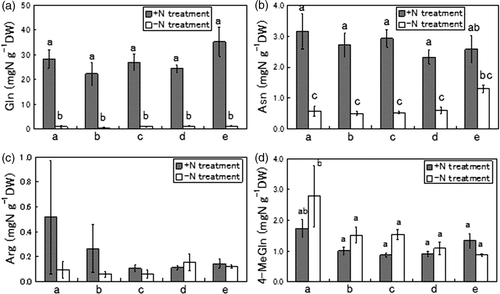
Discussion
In Experiment 1, analysis of the total concentration of amino acids in the cortex and stele indicated that supplying N to the culture solution triggered an increase in the free amino acid concentration in the cortex [from 5.1 (−N) to 27.7 (+N) mg N g−1 DW] rather than the stele [from 5.8 (−N) to 14.4 (+N) mg N g−1 DW]. The cortex/stele ratio of the area of transverse sections of tulip roots was about 10:1, and the cortex makes up most of the volume of tulip roots. Therefore, the major site of N accumulation from culture solution in tulip roots is considered to be the cortex rather than stele during autumn and winter seasons. Generally, plant roots absorb N in the form of nitrate or ammonium from the epidermis or cortex and some part of N is assimilated into amides–glutamine or asparagine. These amino acids are transported from cortex to stele thorough the casparian strip by symplastic transport, released to the apoplast in the stele, and loaded into xylem vessels. Then they are transported from roots to shoots by transpiration and root pressure. In tulip plants in autumn and winter, the plants have not sprouted yet and no transpiration occurs. Therefore, it is reasonable that N is accumulated in the cortex and not transported to the stele and other organs. After sprouting the stored N is actively transported to the shoot and used for leaf growth (Ohyama et al. Citation1988e).
UPLC confirmed that Gln was the predominant amino acid in tulip roots cultivated with +N treatment. This is the first report that Gln mainly accumulates in the cortex in tulip roots rather than the stele. Asn and Arg, which are relatively major amino acids, followed by Gln in tulip roots supplied with N, were also highly accumulated in the cortex. The tulip roots should convert the absorbed ammonium to Gln in the roots. Gln synthesis may depend on the enzyme activity of glutamine synthetase, although no direct evidence of this has been published yet.
The concentration of 4-MeGln in the cortex was not significantly different from that in the stele. Rather, it was higher in the −N treatment than in the +N treatment. +N and −N treatments did not affect the concentration at either site.
The similar concentrations of 4-MeGln in the cortex and stele might suggest that the N in 4-MeGln is mainly derived not from the culture solution but derived from the bulb. Ohyama et al. (Citation1988f) reported that 15N abundance (% of 15N labeled N in total N) was higher in Gln (92%), Arg (80%) and Asn (79%), when a 15N-labeled culture solution was supplied to the tulip plants for one month in winter. However, the 15N abundance in 4-MeGln was only 31%, which means about 70% of N was derived from the bulb.
In Experiment 2, the total concentration of free amino acids in five sections of roots from the base to the tip was analyzed. The +N treatment promoted free amino acid accumulation in all the segments at the same level. This result suggested that accumulated N is stored at the same sites as the sites of absorption from culture solution, and the activities of N absorption and accumulation are the same from base to root tip. Gln was the predominant amino acid at all vertical positions in tulip roots supplied with N (+N treatment). This suggests that the activity of N absorption and assimilation into Gln is similar among root positions. Asn and Arg also accumulated near the absorption sites. This result confirmed those obtained for the upper and lower roots (Komiyama et al. Citation2003). The +N treatment had no effect on 4-MeGln accumulation in tulip roots at any positions. But the 4-MeGln concentration in the −N treatment in the position from the basal part (“a”) to the root tip (“d”) was higher than that in the +N treatment. The substance 4-MeGln is a temporary storage form of N in tulip roots, so 4-MeGln concentration may have been little affected by N treatment. But much of the accumulated 4-MeGln was derived from N transported from the bulb; it was not derived from the N of the culture solution.
In the vertical profile of the tulip roots, 4-MeGln was mainly accumulated near the basalplate. This may be because 4-MeGln is a major N compound from bulb scales. In addition, in the −N treatment, the concentration of all amino acids was lower in the cortex than in the stele (), suggesting that amino acids such as 4-MeGln are supplied from the bulb through the stele to the cortex and the growing tip. The presence of 4-MeGln is highly specific to tulip plants and groundnut (Harry et al. Citation1981; Harry and Eugene Citation1984). It was speculated that 4-MeGln is a temporary storage form of N in leaves, flower stalks, and roots of tulip plants after sprouting in spring (Ohyama et al. Citation1988f). However, 4-MeGln may be a form of N transported from the bulb to roots during autumn and winter.
In conclusion, tulip roots accumulate absorbed N mainly in the cortex in the form of Gln in autumn and winter before sprouting, and the uptake of inorganic N (nitrate and ammonium) and Gln accumulation in the vertical position was almost the same from root base to root tip. From the absorbed N, Gln, Asn, Arg are mainly accumulated in the cortex of the roots rather than in the stele. At this stage before sprouting, the transport of N from root to shoot has not yet started. The +N treatment did not affect the concentration of 4-MeGln in the cortex or stele, unlike the concentration of Gln, Asn and Arg. The concentration of the specific amino acid 4-MeGln was similar between the cortex and stele, and higher in the root base than in the root tip. These results may be due to the fact that the main origin of 4-MeGln in the roots is the bulb, and 4-MeGln is transported from the basalplate to the root tip through the stele to support root growth.
References
- Baba , A and Ikarashi , T . 1967 . Mineral nutrition of tulip flowering phase (I) . Shokubutsu Seiri , 6 : 47 – 55 . (in Japanese)
- Baba , A and Ikarashi , T . 1968 . Mineral nutrition of tulip flowering phase (II) . Shokubutu Seiri , 7 : 13 – 20 . (in Japanese)
- Baba , A , Ikarashi , T and Okumura , N . 1979 . Concentration of mineral nutrients in bulb of tulip cropped in dune fields and secondary cropped paddy field in Niigata Prefecture . Bull. Facul. Agric. Niigata Univ. , 31 : 81 – 89 . (in Japanese with English summary)
- De Hertogh , AA , Aung , LH and Benshop , M . 1983 . The tulip: Botany, usage, growth and development . Hort. Rev. , 5 : 45 – 125 .
- Done , J and Fowden , L . 1952 . A new amino acid amide in the groundnut plant (Arachis hypogaea): Evidence of the occurrence of γ-methyleneglutamine and γ-methyleneglutamic acid . Biochem. J. , 51 : 451 – 458 .
- Fitter , A . 2002 . “ Characteristics and functions of root systems ” . In Plant Roots, the Hidden Half, , 3rd , Edited by: Yoav , W , Eshel , A and Kafkafi , U . 21 – 50 . New York, Basel : Marcel Dekker, Ink .
- Fowden , L and Steward , FC . 1957 . Nitrogenous compounds and nitrogen metabolism in the Liliaceae. I. The occurrence of soluble nitrogenous compounds . Ann. Bot. , 21 : 53 – 67 .
- Harry , CW and Eugene , ED . 1984 . Inhibition by auxins of 4-Methyleneglutamic acid synthesis in tissue cultures of peanut seeds . Plant Physiol. , 76 : 161 – 164 .
- Harry , CW , Gary , KP and Eugene , ED . 1981 . 4-Methylaneglutamine in peanut plants: Dynamics of formation, levels, and turnover in relation to other free amino acids . Plant Physiol. , 68 : 588 – 593 .
- Hayashi , H , Ohyama , T , Kera , T , Ikarashi , T and Chino , M . 1987 . Rapid analysis of 4-methyleneglutamine by ion chromatography . Jpn J. Soil Sci. Plant Nutr. , 58 : 606 – 608 . (in Japanese with English summary)
- Kitajima , N , Ohyama , T and Ikarashi , T . 1991 . Analysis of nitrogen accumulation in tulip roots during winter season by split root experiment . Jpn. J. Soil Sci. Plant Nutr. , 62 : 64 – 70 . (in Japanese with English summary)
- Komiyama , S , Tanabe , S , Murayama , A , Soraya , R , Ikarashi , T , Ohtake , N , Sueyoshi , K and Ohyama , T . 2003 . Site of nitrogen accumulation in tulip (Tulipa gesneriana L.) roots during winter . Soil Sci. Plant Nutr. , 49 : 611 – 618 .
- Komiyama , S , Yamazaki , T , Hori , E , Shida , Y , Murayama , A , Ikarashi , T and Ohyama , T . 1997 . Degradation of storage starch in tulip bulb scales induced by cold temperature . Jpn. J. Soil Sci. Plant Nutr. , 68 : 23 – 29 . (in Japanese with English summary)
- Marschner , H . 1995 . “ Effect of internal and external factors on root growth and development ” . In In Mineral Nutrition of Higher Plants, , 2nd , 508 – 536 . London : Academic Press .
- Ohyama , T , Hoshino , T and Ikarashi , T . 1988a . Isolation and structure of a new organic acid accumulated in tulip plant . Soil Sci. Plant Nutr. , 34 : 75 – 86 .
- Ohyama , T , Ikarashi , T and Baba , A . 1985 . Nitrogen accumulation in the roots of tulip plants (Tulipa gesneriana) . Soil Sci. Plant Nutr. , 31 : 581 – 588 .
- Ohyama , T , Ikarashi , T and Baba , A . 1986 . Isolation of 4-methyleneglutamine from fresh leaves of tulip plants . Jpn. J. Soil Sci. Plant Nutr. , 57 : 300 – 303 . (in Japanese with English summary)
- Ohyama , T , Ikarashi , T and Baba , A . 1988b . Effect of cold storage treatment for forcing bulbs on the C and N metabolism of tulip plants . Soil Sci. Plant Nutr. , 34 : 513 – 533 .
- Ohyama , T , Ikarashi , T , Matsubara , T and Baba , A . 1988c . Behavior of carbohydrates in mother and daughter bulbs of tulips (Tulipa gesneriana) . Soil Sci. Plant Nutr. , 34 : 405 – 415 .
- Ohyama , T , Ikarashi , T and Obata , A . 1988d . Origin of nitrogen accumulated in tulip roots during winter season . Soil Sci. Plant Nutr. , 34 : 621 – 626 .
- Ohyama , T , Ikarashi , T , Obata , A and Baba , A . 1988e . Role of nitrogen accumulated in tulip roots during winter season . Soil Sci. Plant Nutr. , 34 : 341 – 350 .
- Ohyama , T , Kera , T and Ikarashi , T . 1988f . Occurrence of 4-methyleneglutamine and 2-oxo-4-methyl-3-pentene-1,5-dopoc acid in leaves and stem of tulip plants . Soil Sci. Plant Nutr. , 34 : 613 – 620 .
- Ohyama , T , Yamazaki , T , Hori , E , Shida , Y , Komiyama , S and Ikarashi , T . 1996 . Induction of a-amylase activity and starch degradation by cold temperature in tulip bulb scales . Bull. Facul. Agric. Niigata Univ. , 48 : 81 – 92 . (in Japanese with English summary)
- Rees , AR . 1966 . The physiology of ornamental bulbous plants . Bot. Rev. , 32 : 1 – 23 .
- Zacharius , RM , Polland , JK and Steward , FC . 1954 . γ-methyleneglutamine and γ-methyleneglutamic acid in the tulip (Tulipa gesneriana) . J. Amer. Chem. Soc. , 76 : 1961 – 1962 .
- Zhang , H , Rong , H and Pilbeam , D . 2007 . Signaling mechanisms underlying the morphological responses of the root system to nitrogen in Arabidopsis thaliana . J. Exp. Bot. , 58 : 2329 – 2338 .