Abstract
Methylglycinediacetic acid (MGDA) is a readily biodegradable complexing agent in compliance with Organization for Economic Cooperation and Development standards. In the present study, the use of MGDA for iron (Fe) and arsenic (As) bioavailability and uptake by rice plants (Oryza sativa L.) was investigated. The highest plant biomass was observed at pH 7, and the growth of rice seedlings decreased significantly (p < 0.05) with increasing pH of the nutrient solution. This might be due to Fe deficiency to the plant at alkaline pH. When rice seedlings were grown with different concentrations of MGDA (0.1, 0.25, 0.5, 1.0, 2.5, and 5 mM), the highest plant biomass was observed at 0.25 mM MGDA, while further increases of the ligand concentration decreased the plant growth. Fe concentrations on rice root surfaces decreased gradually with increasing MGDA concentrations in the growing medium, while Fe concentrations in rice roots and shoots increased with increasing MGDA concentrations up to 0.25 mM and then decreased gradually. This indicates that the concentration of the chelating ligand influences Fe uptake in the plant. Arsenic concentrations on rice root surfaces decreased, while As concentrations in roots and shoots increased with the addition of MGDA in the growing medium, indicating that the ligand enhanced As bioavailability and uptake in rice. The mechanism behind the MGDA effect on Fe and As uptake in plant is likely to be due to that Fe exists mostly in insoluble particulate forms [e.g., ferric oxide (Fe2O3), ferric hydroxide (Fe(OH)3) and ferric oxyhydroxide (FeOOH)] at neutral or alkaline pH, and the soluble [e.g., ferric ion (Fe3+), iron hydroxide ion (Fe(OH)2+) and iron dihydroxide ion ()] and apparently soluble (colloidal) fractions of Fe are increased at moderate concentrations of the ligand that increases Fe bioavailability. Since arsenate [As(V)] binds to the insoluble Fe-oxides/hydroxides, the binding sites for As(V) decreases with the increase of the soluble fractions of Fe by the ligand, which slightly increased As uptake in rice plants.
1. Introduction
Iron (Fe) is an essential nutrient element for plant growth and development that is involved in several physiological activities such as respiration, photosynthesis, and many other cellular and biochemical functions such as DNA synthesis, nitrogen fixation, and hormone production (Vert et al. Citation2002). Fe deficiency causes chlorosis in green plants because it is required in the production of chlorophyll-a (Chl-a) (Kumar and Soll Citation2000). Although abundant in nature, Fe forms insoluble ferric oxides and hydroxide [e.g., ferric oxide (Fe2O3), ferric hydroxide (Fe(OH)3) and ferric oxyhydroxide (FeOOH)] in the presence of oxygen (O) at neutral or alkaline pH (Guerinot and Yi Citation1994) and thus has low bioavailability to plants at standard soil pH, resulting in Fe deficiency being a common problem in neutral and alkaline soils. Fe deficiency can also occur if the soil is too waterlogged. Therefore, Fe deficiency is one of the most common nutritional problems in paddy fields, especially in calcareous soil (Xiao et al. Citation2008).
High concentrations of arsenic (As) in paddy soils from natural and anthropogenic sources increase As uptake in rice (Oryza sativa L.) plants (Wang et al. Citation2006; Rahman et al. Citation2007c), can cause toxicity (Rahman et al. Citation2006; Panaullah et al. Citation2009), and potentially reduce rice yield (Marin et al. Citation1992; Rahman et al. Citation2007a). Phytoextraction, a process of heavy metal uptake in aerial parts of plants from contaminated soils by hyperaccumulating plants, would reduce heavy metal contamination in soil, and uptake in food crops (Raskin et al. Citation1997; Tu et al. Citation2002). Arsenic solubility and bioavailability is reduced at alkaline soil pHs due to the adsorption of arsenate [As(V)], the predominant species in aerobic environments, onto variable charged minerals (e.g., ferric ion (Fe3+) and aluminum ion (Al3+)) (Xu et al. Citation2008). Therefore, increase of As solubility and bioavailability in soil may influence As uptake in plants.
Despite its abundance in soil, Fe is only slightly soluble under aerobic conditions, especially in high-pH and calcareous soils (Marschner Citation1995). Higher plants have two distinct strategies to acquire insoluble/slightly soluble Fe from the rhizosphere: (i) the reduction strategy of nongraminaceous (strategy I) plants, and (ii) the chelation strategy of graminaceous (strategy II) plants (Romheld and Marschner Citation1986; Romheld Citation1987; Kobayashi and Nishizawa Citation2012). Takagi (Citation1976) discovered the mugineic acid family phytosiderophores (MAs), which are Fe3+-solubilising molecules (also known as phytosiderophores) secreted from Fe-deficient graminaceous plants including rice, as important chelators for Fe uptake. In a recent review, Kobayashi and Nishizawa (Citation2012) discused the roles of phytosiderophores in Fe uptake, translocation, and regulation in nongraminaceous and graminaceous plants. In addition to the Fe3+-solubilising phytosiderophores, rhizospheric microbes also exude siderophores to solubilize ferric Fe in the rhizosphere, and thus render Fe soluble and bioavailable, resulting in uptake by graminaceous plants via specific membrane receptors (Romheld and Marschner Citation1986; Romheld Citation1987). Synthetic chelating ligands belonging predominantly to the two groups aminopolycarboxylates and polyphosphonates are also effective and have widely been used for increasing Fe bioavailability and uptake in plants (Gil-Ortiz and Bautista-Carrascosa Citation2004; Rahman et al. Citation2009), and for correcting Fe-chlorosis in plants (Alvarez-Fernandez et al. Citation2005). These synthetic chelating ligands can also increase the bioavailability and uptake of heavy metals in plants (Luo et al. Citation2006; Wang et al. Citation2007).
Aminopolycarboxylates such as ethylenediaminetetraacetic acid (EDTA), nitrilotriacetate (NTA), and diethylenetriaminepentaacetate (DTPA) are popular and widely used chelating agents to achieve this purpose. However, these aminopolycarboxylates are quite persistent in the environment because of their low biodegradability (Kos and Lestan Citation2003). This, in addition to their high affinity for heavy metal complexation, results in an increased risk of leaching (Bucheli-Witschel and Egli Citation2001) and release of heavy metals into underground and surface waters. Therefore, these aminopolycarboxylates are being replaced by a new generation of biodegradable complexing agents (Kos and Lestan Citation2003; Cao et al. Citation2007). Biodegradable chelating ligands, such as methylglycinediacetic acid (MGDA), SS-ethylenediamine-N,N′-disuccinic acid (EDDS) and hydroxyiminodisuccinic acid (HIDS) are less persistent and, therefore, are regarded as potential alternatives to EDTA for increasing Fe bioavailability and uptake in plants (Tandy et al. Citation2006; BASF Citation2007; Rahman et al. Citation2009; Jachula et al. Citation2012).
MGDA () is a strong complexing agent, readily biodegradable in compliance with Organization for Economic Cooperation and Development (OECD) standards (BASF Citation2007; Jachula et al. Citation2012). MGDA belongs to the group of aminopolycarboxylic compounds, and is characterized by high biodegrability, nontoxicity, high stability in the entire pH range and at high temperature (up to100°C), and high solubility in water in the large pH range 2–13 (Schmidt and Brauch Citation2004; BASF Citation2007; Jachula et al. Citation2012). Compared to EDTA, MGDA showed a far higher biodegradability. It was found that 89–100% of MGDA can be degraded in 14 d, while no EDTA was degraded in 30d. MGDA also showed higher biodegradability than EDDS (90% of EDDS can be degraded in 20 d) (Kos and Lestan Citation2003). The most important property of MGDA is its ability to form water-soluble complexes with polyvalent ions with metal ions [calcium (Ca), magnesium (Mg), copper (Cu), lead (Pb), zinc (Zn), cadmium (Cd), mercury (Hg), manganese (Mn), and Fe], over a wide pH range from 2 to 13.5. The logarithmic stability constant (log K) for MGDA-Fe3+ complexes is 16.5, the highest among all metal ions, indicating that MGDA has a high binding affinity for Fe. The high stability of MGDA-Fe3+ complexes prevents Fe3+ ions from participation that results in Fe bioavailability. It is important to mention that MGDA is a tetradentate chelating ligand, and the chelation involves three carboxylate groups and a nitrogen (N) atom (BASF Citation2007).
MGDA has an excellent ecological and toxicological profile. The active ingredient contained in MGDA is classified as being readily biodegradable according to the OECD criteria and, therefore, there are no restrictions on the use of MGDA in many applications (BASF Citation2007). Previously, MGDA was studied for heavy metal (Pb and Zn) phytoextraction by Mirabilis jalapa L. (Cao et al. Citation2007). However, there is no report on the use of this biodegradable chelating ligand for Fe uptake and for As phytoextraction. The present study investigated the effect of MGDA as Fe-fertilizer on Fe bioavailability and uptake in rice plants. The effect of this chelating ligand on As phytoextraction was also investigated since the biogeochemistry of Fe and chelating ligands in the growing medium significantly influence As uptake in plants.
2. Materials and Methods
2.1. Seed sterilization and germination
The test species was Oryza sativa L. (rice) cv. BRRI Dhan29, and the seeds were collected from the Bangladesh Rice Research Institute (BRRI), Bangladesh. The seeds were surface-sterilized by soaking approximately 100 g seeds in 200 mL of 1% methyl-1-butylcarbamoyl-2-benzimidazole carbonate solution for 10 min. After that, the seeds were washed with deionized (DI) water (using an E-pure system; Barnstead) and kept in DI water at 20°C for 24 h. The seeds were then washed and transferred to DI water at 45 then 52°C for 2 and 10 min, respectively.
2.2. Chemicals
Glassware and dishes were washed with detergent and then 5 M hydrochloric acid (HCl) solution before rinsing in DI water for eight times before use. Chemical solutions were prepared with DI water. A stock solution of 10 M MGDA was prepared by dissolving methylglycinediacetic acid (Kanto Chemical, Japan) in DI water. All other chemical reagents used in the experiment were of analytical grade.
2.3. Nutrient solution
Sterilized rice seeds were germinated on pre-sterilized blotting paper for one week with DI water. Modified Murashige and Skoog (MS) (Murashige and Skoog Citation1962) growth medium was used to grow the rice seedlings, with ferrous sulfate heptahydrate (FeSO4 · 7H2O) used as the Fe source instead of the suggested Sodium-iron(III)-EDTA (NaFe3+-EDTA) (). The Fe concentration in the pre-experimental solution (used for growing the rice seedlings prior to the application of treatments) was 27.8 mg L−1, while the Fe concentration was 50 mg L−1 in the experimental solution. The pH of the pre-experimental solution was adjusted to 6.5, while pH was adjusted to 8.5 in the experimental solution using 0.1 M HCl or potassium hydroxide (KOH) to induce Fe-oxides (Fe-plaque) on rice roots.
Table 1. Composition of modified Murashige and Skoog (MS) pre-experimental* and experimental solutions used in the experiment
2.4. Experimental setup
Rice seedlings were transferred to the experimental solution after growing in the pre-experimental solution for 5d. Approximately 100 mL of the solution was added to 250-mL polycarbonate bottles. Three uniform seedlings were planted in each bottle. Rice plants were grown in a plant growth chamber set to 14:10 h light/dark schedule, 100–125 µEm−2 s−1 light intensity, and 20 ± 2°C. To investigate the effect of pH and MGDA concentrations on growth, rice seedlings were grown in experimental solutions with different pHs (6, 7, 8, 9, 10, and 11) and MGDA concentrations (0, 0.1, 0.25, 0.5, 1.0, 2.5, and 5.0 mM). To investigate the effect of MGDA concentrations on Fe and As bioavailability and uptake, rice seedlings were grown in two stages. In the first stage, rice seedlings were grown with different concentrations of MGDA and 50 mg L−1 of Fe to observe the effect of the chelating ligand on Fe uptake. In the second stage, 6 µM disodium hydrogen arsorate heptahydrate (Na2HAsO4 · 7H2O) was added to the nutrient solution containing 0.25 mM MGDA and 50 mg L−1 Fe to see the effect of the chelating ligand on As uptake. Rice seedlings were grown for two weeks in the experimental solution for each of these stages. Each treatment was replicated three times. The solution was not changed during Stages 1 and 2; however, the pH of the solution was checked and adjusted twice a week using 1 M HCl or KOH.
2.5. CBE-extraction of Fe-plaque from root surfaces
At harvest, the shoots were cut 1 cm above the join with the roots and roots, and shoots were separated. The Fe-plaque from root surfaces was extracted using the citrate–bicarbonate–EDTA (CBE) technique, a modified version of the dithionite–citrate–bicarbonate (DCB) extraction technique developed by Taylor and Crowder (Citation1983) originally designed to measure extra- and intra-cellular Fe and As in rice roots. The CBE solution was prepared from 0.03, 0.125 and 0.05 M of sodium citrate, sodium bicarbonate, and EDTA, respectively, and the pH of the solution was adjusted to 8. Roots were treated with 10 mL of CBE solution for 60 min at room temperature. The roots were then rinsed with DI water three times, and the rinsed water was added to the CBE-extracts to make a total of volume of 30 mL.
2.6. Sample preparation and chemical analysis
After rinsing with DI water four times, the root samples were transferred to clean absorbent paper to remove the water from the root surfaces. Both the root and the shoot samples were dried at 65°C until they reached constant weights. Then the entire dried samples were weighed (10–30 and 30–90 mg of roots and shoots, respectively) and placed in 50-mL polyethylene tubes (DigiTubes, SCP Science, Canada) for digestion. Five milliliters of 65% nitric acid (HNO3) was added to each tube. After 12 h incubation at room temperature, the samples were heated on a heating block (DigiPREP, SCP Science, Canada) at 95°C for 2 h. After cooling to room temperature, 3 mL of 30% hydrogen peroxide (H2O2) was added to each sample and the samples were heated again to 105°C for 30 min. Then, the digests were diluted to 30 mL with DI and analyzed for total As and Fe concentrations by graphite-furnace atomic absorption spectrometer (GF-AAS, Perkin Elmer, AAnalyst 800). At least two reagent blanks and three certified standard reference material (CSRM) 1573a (tomato (Solanum lycopersicum L.) leaf from NIST, USA), and three replicate samples were included in each analytical batch to check the precision of As analysis by GF-AAS.
2.7. Statistical analysis
Data analysis was performed using the SPSS package (ver. 17.0 for Windows, Chicago, SPSS Inc.). Comparison of treatment means was made by Duncan's Multiple Range Test (DMRT).
3. Results
3.1. Effect of pH and MGDA concentrations on rice seedling growth
The growth of rice seedlings in terms of root and shoot biomass production decreased significantly (p < 0.05) with increasing pH of the solution (). The highest dry weight (DW) of plant biomass, 57.15 ± 10.82 mg, was observed at pH 7, followed by 53.65 ± 2.19 mg DW at pH 6, while the growth of rice seedlings was minimal (14.85 ± 5.16 mg DW) at pH 11. Both root and shoot biomasses decreased gradually (p < 0.05) with increasing pH above 7. The growth of rice seedlings was also influenced significantly (p < 0.05) by MGDA concentrations (). The highest growth of rice seedlings were observed in 0.25 mM MGDA concentration, and the growth decreased gradually with further increasing of MGDA concentration. About 24.20 ± 1.80 mg DW of shoots was found in 0.25 mM MGDA treatment, which was significantly higher than those in other treatments of the ligand.
Figure 2. Biomass of rice (Oryza sativa L.) seedlings at pH between 6 and 11 after growing in Murashige and Skoog (MS) nutrient solution for a total of 3 weeks. Values are mean ± SD (n = 3). Different letters indicate significant differences (p < 0.05) between treatments for roots (lower case) or shoots (upper case).
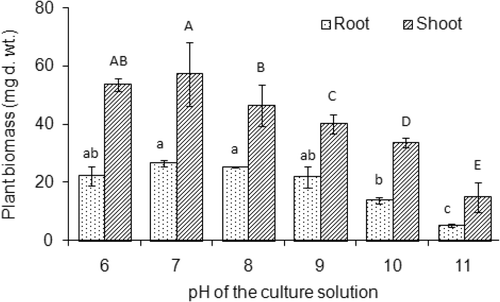
Figure 3. Shoot biomass of rice (Oryza sativa L.) seedlings grown at different concentrations of methylglycinediacetic acid (MGDA) in nutrient solution. The age of rice seedlings was 3 weeks from germination. Values are mean ± SD (n = 3). Different letters indicate significant differences (p < 0.05) between treatments.
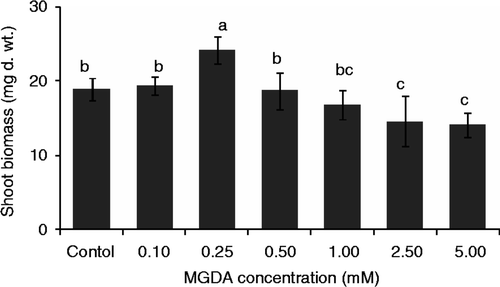
3.2. Effect of MGDA concentrations on Fe and As uptake in rice
To investigate the effect of MGDA concentrations on Fe and As bioavailability and uptake, rice seedlings were grown with different concentrations of the ligand. Fe concentration in rice root surfaces of control treatment was 41.11 ± 5.49 µmol g−1 DW, which was significantly (p < 0.01) higher than that of the MGDA treatments (). Compared to the high ligand concentrations (2.5 and 5.0 mM), Fe concentration in rice root surfaces of moderate ligand concentrations (between 0.1 and 1.0 mM) was significantly (p < 0.05) higher. Fe concentrations were higher in roots than in root surfaces. In roots, the highest Fe concentration (74.91 ± 12.07 µ mol g−1 DW) was observed at a moderate concentration of MGDA (0.25 mM), which was about 26 and 19–39% higher than its concentration at control and other MGDA concentrations, respectively (). In shoots, Fe concentration at moderate MGDA concentrations (between 0.25 and 1.0 mM) was significantly (p < 0.05) higher than that of high ligand concentrations (). About 21.28 ± 3.91 and 20.48 ± 1.09 µmol g−1 DW of Fe was observed in shoots of 0.25 and 0.5 mM MGDA treatments, which was about 40 and 20–44% higher than that of control and other MGDA concentrations, respectively.
Figure 4. The influence of methylglycinediacetic acid (MGDA) concentrations on iron (Fe) concentrations on root surfaces (a), in roots (b), and in shoots (c) of rice seedlings (Oryza sativa L.). Fe concentrations in roots were measured after removing Fe from root surfaces by citrate–bicarbonate–ehylenediaminetetraacetic acid (CBE) solution. Values are mean ± SD (n = 3). Different letters indicate significant differences (p < 0.05) between treatments.
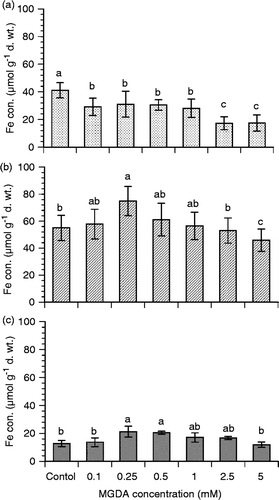
The influence of MGDA on As uptake in rice was investigated by growing rice seedlings with 0.25 mM of the ligand since Fe uptake in roots and shoots was highest at this concentration. Arsenic concentration on rice root surfaces in control treatment (without ligand) was about 0.019 ± 0.002 µmol g−1 DW, which decreased to 0.012 ± 0.002 µmol g−1 DW for the addition of 0.25 mM MGDA (). The As concentrations in roots and shoots of rice seedlings were increased slightly (p > 0.05) for the addition of the ligand in the solution. The As concentrations in roots of control and ligand-treated plants were 0.068 ± 0.009 and 0.079 ± 0.013 µmol g−1 DW, respectively. In shoots, As concentration was 0.012 ± 0.002 µmol g−1 DW, which increased to 0.017 ± 0.003 µmol g−1 DW for MGDA treatment (). The results showed that As uptake was not increased significantly (p > 0.05) by MGDA. Total As concentration in roots was about 6-fold higher than that in shoots of rice seedlings. The results indicate that a major part of the total As in rice plants is stored in the root (about 68%) compared to that in the shoot (12%) and root surface (20%).
Figure 5. Arsenic (As) uptake in rice (Oryza sativa L.) seedlings as influenced by methylglycinediacetic acid (MGDA; 0 and 1.0 mM). Arsenic concentrations in roots were measured after removing As from root surfaces by citrate–bicarbonate–ethylenediaminetetraacetic acid (CBE) solution. Values are mean ± SD (n = 3). Different letters indicate significant differences (p < 0.05) between treatments.
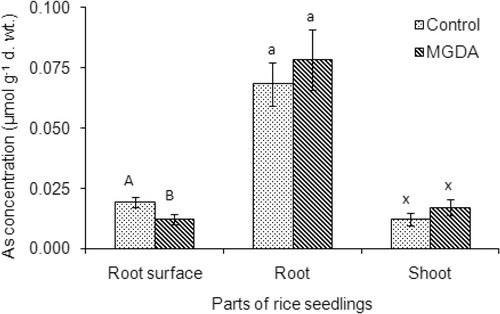
4. Discussion
4.1. pH and MGDA concentration influence Fe uptake and rice growth
The growth of rice seedlings decreased significantly (p < 0.05) with increasing pH of the growth medium (). The results of the present study agree with the results of other studies (Rahman et al. Citation2009; Chun et al. Citation1997). Alam (Citation1981) also reported substantial reduction in rice plant biomass at pH 8.5. It is likely that the decrease in growth is the result of reduced Fe solubility, bioavailability and uptake due to the formation of Fe-oxide/hydroxide precipitates in the growing medium at high pHs under aerobic conditions (Alam Citation1981; Rahman et al. Citation2009). In most soils, Fe3+-oxides are a common source of Fe for plants, which after transformation in the rhizosphere is absorbed by plant in ionic and chelated forms via soil solution (Stein et al. Citation2009). In the common pH range of aerobic soils, the bioavailability of Fe3+ ions is extremely low and insufficient to meet the Fe requirement for plants (Lindsay Citation1988; Schwertmann Citation1991). This is because Fe3+ forms insoluble ferric hydroxide under aerobic conditions and alkaline pHs that decreases Fe bioavailability in the rhizosphere and results in Fe deficiency in plants (Robinson et al. Citation2006). Therefore, the solubility and dissolution rate of the Fe(III)-oxides are important determinants for Fe bioavailability and uptake (Schwertmann Citation1991).
In the present study, the highest growth of rice seedlings was observed in moderate ligand concentration (0.25 mM MGDA), and the growth decreased gradually with further increasing of the ligand concentration (). Synthetic chelating ligands are widely used in agriculture as additives in fertilizer for the increase of Fe bioavailability (Alvarez-Fernandez et al. Citation2005). The present study showed that the concentration of chelating ligands is an important factor that influences Fe bioavailability and uptake in plants. Moderate concentration of chelating ligands would be favourable to plants for chelant-enhanced Fe bioavailability and uptake, and eventually plant growth. However, there might be three possible reasons for the decrease of plant growth at high ligand concentrations; (i) Fe toxicity that might occur as a result of excess Fe uptake at high ligand concentrations due to high Fe solubility; (ii) direct toxicity of the chelating ligand, and (iii) Fe deficiency caused by decreased bioavailability of Fe as result of chelation with the ligand.
Growth of plants depends on the acquisition of sufficient Fe, and excessive uptake of some trace elements such as Fe, Zn, Mn and Cu produces toxicity to organisms (Morel and Hering Citation1993). Fe toxicity is a common nutrient disorder in lowland rice (Stein et al. Citation2009; Audebert and Fofana Citation2009), which occurs in irrigated or rain-fed paddy fields when the soils contain excessive amounts of bioavailable Fe. Associated with leaf discoloration symptoms (bronzing), excessive Fe uptake causes poor growth and tillering (Becker and Asch Citation2005; Audebert and Fofana Citation2009). Ferric ions and their complexes, which have low solubility in aqueous solution, are also extensively buffered by chelation (Morel and Hering Citation1993) that increases its dissolved concentration and rate of uptake by the organisms. Since the concentrations of Fe in roots of rice seedlings is decreased, and since Fe concentrations in shoots were not significantly positively correlated with Fe concentrations in roots (), growth reduction of rice seedlings at high ligand concentrations is unlikely to be due to Fe deficiency in this case.
Fe concentration in rice roots was affected significantly by the concentration of MGDA in the growing medium. The highest concentrations of Fe in rice roots and shoots were observed in the 0.25 mM MGDA treatment compared to those in other treatments (). The results indicate that Fe uptake was high at moderate ligand concentrations (0.25 mM), which might be due to the increase of Fe bioavailability.
Since MGDA concentrations of higher than 0.25 mM decreased Fe concentrations in roots and shoots significantly (p < 0.05), it was assumed that the reduction of rice seedling growth was due to Fe deficiency. Chelating ligands form Fe-ligand complex (Fe-L) with Fe3+ and increase the dissolved fractions of the Fe [e.g., Fe2+, Fe(OH)2+] by dissolving particulate fractions of the element [e.g., Fe2O3, Fe(OH)3, Fe(OOH)]. Dissolution of precipitated/particulate Fe and the increase of dissolved bioavailable fractions of the element are high at moderate equilibrium reaction of Fe and chelating ligand. However, strong ‘Fe-L’ complex formation at high ligand concentrations increases Fe solubility but decreases its bioavailability to plants.
4.2. MGDA increases As uptake in rice
The influence of MGDA on As uptake in rice was investigated. Arsenic concentrations slightly decreased in rice root surfaces while increasing in roots and shoots with the addition of MGDA in the growing medium (). This might be due to the increase of As bioavailability in the growing medium, which is related to the chemistry of Fe, As(V), and the chelating ligand. It has been reported that the formation of Fe-oxides/hydroxides (Fe-plaque) on rice root surfaces at alkaline pHs decreases As uptake into rice roots due to the adsorption of As(V) on the Fe-plaque (Liu et al. Citation2004; Liu et al. Citation2005), and uptake of As depends on the amount of Fe-plaque on root surfaces (Liu et al. Citation2005). Thus, Fe-plaque acts as a ‘buffer’ to prevent As uptake inside the roots as was found in this study. However, the addition of MGDA in the growing medium reduced the amount of Fe-oxides/hydroxides on root surfaces and in the growing medium by dissolving particulate fractions of the Fe [e.g., Fe2O3, Fe(OH)3, Fe(OOH)] and increasing its soluble fractions [e.g., Fe2+, Fe(OH)2+]. Since As(V) has high binding affinity to Fe-oxides/hydroxides, dissolution of the Fe-oxides/hydroxides by the ligand reduces the binding sites for the As(V). The dissolution of the Fe-oxides/hydroxides by MGDA may also have desorbed some of the As(V) that was adsorbed on Fe- oxides/hydroxides on the root surfaces. Thus, MGDA increased As bioavailability in the growing medium that subsequently increased its uptake in roots.
Arsenic concentration was about 6 times higher in roots than in shoots indicating that As translocation from roots to shoots was limited (). Other studies also showed that As translocation from rice roots to shoots is limited, and As concentration is several orders of magnitude higher in roots than in shoots of the plant (Wang et al. Citation2006; Rahman et al. Citation2007b). This is because As(V) comprises the major species in the rhizosphere soil of paddy field, which is taken up by rice plants through phosphate transporters (Meharg and Hartley-Whitaker Citation2002), and is reduced to arsenite (AsIII) inside the root cells (Zhao et al. Citation2009). Arsenite has a high affinity to the sulfhydryl (–SH) groups of peptides such as glutathione (GSH) and phytochelatins (PCs) (Zhao et al. Citation2009). In vitro studies showed that GSH and As(III) form a (GS)3-As(III) complex with cysteinyl sulphhydryl (Delnomdedieu et al. Citation1994). Complexation of As(III) with thiols in roots limits As transport from roots to shoots. In addition, As(III) is sequestered into vacuoles of root cells (Zhao et al. Citation2009), which may also limit As translocation from roots to shoots. Thus, (i) reduction of As(V) to As(III) and subsequent As(III)-thiol complexation, and (ii) vacuolar sequestration of As(III) in root cells, decrease As translocation from roots to shoots.
Although several plant physiological activities have been found to decrease As translocation from roots to shoots (as discussed above), the present study showed that MGDA slightly increased As translocation from roots to shoots (p > 0.05; ). However, chelant-enhanced translocation of As and heavy metals from rice roots to shoots has been reported by some researchers (Luo et al. Citation2006; Rahman et al. Citation2009). Since MGDA improves Fe bioavailability and uptake significantly without a significant increase of As uptake in rice plant, this biodegradable chelating ligand would be an useful Fe-fertilizer in As-contaminated and Fe-deficient paddy fields. However, more intensive studies are necessary to see how this chelating ligand works in the field.
5. Conclusion
Chelating ligands favour Fe uptake in plants; however, their concentration is one of the important determinants that influence Fe solubility, bioavailability, and uptake. Generally, Fe bioavailability and uptake in plants should be increased with increasing chelating ligand concentration. But the present study showed that Fe concentrations in roots and shoots of rice seedlings decreased at high MGDA concentrations. Therefore, why Fe uptake in rice plant decreased at high ligand concentrations is an important concern that needs to be further investigated. The possible reasons for the decrease of Fe uptake at high ligand concentrations would be that (i) the chelating ligand binds most of the Fe in the growing medium to form strong complex of ‘Fe-ligand (Fe-L)’ that may increase Fe solubility, but the large molecule of ‘Fe-L’ complex may not be taken up by membrane transporters, and (ii) the concentration of soluble Fe is decreased due to a lopsided equilibrium reaction of the Fe ions and chelating ligand. Therefore, it is hypothesized that Fe bioavailability depends on the stability constant and concentration of chelating ligands, which control the complex formation rate constant (KFe–L) and equilibrium reactions between Fe and chelant in the growing medium.
Acknowledgments
This research was supported partly by Grants-in-Aid for Scientific Research (20·08343) from the Japan Society for the Promotion of Science (JSPS). The authors also wish to acknowledge the University of Technology, Sydney (UTS), Australia, for supporting the work. We also would like to thank Dr Suzie Reichman, School of Civil, Environmental and Chemical Engineering, RMIT University, Australia, for reviewing the manuscript prior to submission.
References
- Alam , SM . 1981 . Effects of solution pH on the growth and chemical composition of rice plants . J. Plant Nutr. , 4 : 247 – 260 .
- Alvarez-Fernandez , A , Garcia-Marco , S and Lucena , JJ . 2005 . Evaluation of synthetic iron(III)-chelates (EDDHA/Fe3 +, EDDHMA/Fe3+ and the novel EDDHSA/Fe3+) to correct iron chlorosis . Eur. J. Agron. , 22 : 119 – 130 .
- Audebert , A and Fofana , M . 2009 . Rice yield gap due to iron toxicity in west Africa . J. Agron. Crop Sci. , 195 : 66 – 76 .
- BASF 2007: Technical information, Trilon® M, TI/EVD 1418 e, BASF Corporation, http://chemagent.ru/index.php?id=243&cid=357&fid=19&task=download&option=com_flexicontent (January, 2012)
- Becker , M and Asch , F . 2005 . Iron toxicity in rice–conditions and management concepts . J. Plant Nutr. Soil Sci. , 168 : 558 – 573 .
- Bucheli-Witschel , M and Egli , T . 2001 . Environmental fate and microbial degradation of aminopolycarboxylic acids . FEMS Microbiol. Rev. , 25 : 69 – 106 .
- Cao , A , Carucci , A , Lai , T , La Colla , P and Tamburini , E . 2007 . Effect of biodegradable chelating agents on heavy metals phytoextraction with Mirabilis jalapa and on its associated bacteria . Eur. J. Soil Biol. , 43 : 200 – 206 .
- Chun , SC , Schneider , RW and Cohn , MA . 1997 . Sodium hypochlorite: Effect of solution pH on rice seed disinfestation and its direct effect on seedling growth . Plant Dis. , 81 : 821 – 824 .
- Delnomdedieu , M , Basti , MM , Otvos , JD and Thomas , DJ . 1994 . Reduction and binding of arsenate and dimethylarsinate by glutathione: A magnetic resonance study . Chem-Biol. Interact. , 90 : 139 – 155 .
- Gil-Ortiz , R and Bautista-Carrascosa , I . 2004 . Effects of Fe-EDDHA chelate application on evolution of soil extractable iron, copper, manganese, and zinc . Commun. Soil Sci. Plant Anal. , 35 : 559 – 570 .
- Guerinot , ML and Yi , Y . 1994 . Iron: nutritious, noxious, and not readily available . Plant Physiol. , 104 : 815 – 820 .
- Jachula , J , Kołodynska , D and Hubicki , Z . 2012 . Methylglycinediacetic acid as a new complexing agent for removal of heavy metal ions from industrial wastewater . Solvent Extr. Ion Exch. , 30 : 181 – 196 .
- Kobayashi , T and Nishizawa , NK . 2012 . Iron uptake, translocation, and regulation in higher plants . Ann. Rev. Plant Biol. , 63 : 131 – 152 .
- Kos , B and Lestan , D . 2003 . Influence of a biodegradable ([S,S]-EDDS) and nondegradable (EDTA) chelate and hydrogel modified soil water sorption capacity on Pb phytoextraction and leaching . Plant Soil , 253 : 403 – 411 .
- Kumar , AM and Soll , D . 2000 . Antisense HEMA1 RNA expression inhibits heme and chlorophyll biosynthesis in arabidopsis . Plant Physiol. , 122 : 49 – 56 .
- Lindsay , WL . 1988: Solubility and redox equilibria of iron compounds in soils. In Iron in Soils and Clay Minerals, Eds. Stucki JW, Goodman BA, Schwertmann U, pp. 37–60. NATO ASI Ser, Dordrecht
- Liu , WJ , Zhu , YG and Smith , FA . 2005 . Effects of iron and manganese on arsenic uptake by rice seedlings (Oryza sativa L.) grown in solution culture supplied with arsenate and arsenite . Plant Soil , 277 : 127 – 138 .
- Liu , WJ , Zhu , YG , Smith , FA and Smith , SE . 2004 . Do iron plaque and genotypes affect arsenate uptake and translocation by rice seedlings (Oryza sativa L.) grown in solution culture? . J. Exp. Bot. , 55 : 1707 – 1713 .
- Luo , C , Shen , Z , Lou , L and Li , X . 2006 . EDDS and EDTA-enhanced phytoextraction of metals from artificially contaminated soil and residual effects of chelant compounds . Environ. Pollut. , 144 : 862 – 871 .
- Marin , A , Masscheleyn , P and Patrick , W . 1992 . The influence of chemical form and concentration of arsenic on rice growth and tissue arsenic concentration . Plant Soil , 139 : 175 – 183 .
- Marschner , H . 1995 . Mineral Nutrition of Higher Plants , London : Elsevier .
- Meharg , AA and Hartley-Whitaker , J . 2002 . Arsenic uptake and metabolism in arsenic resistant and nonresistant plant species . New Phytol. , 154 : 29 – 43 .
- Morel , FMM and Hering , JG . 1993 . Principles and Applications of Aquatic Chemistry: Complexation , New York : Wiley .
- Murashige , T and Skoog , F . 1962 . A revised medium for rapid growth and bio assays with tobacco tissue cultures . Physiol. Plant. , 15 : 473 – 497 .
- Panaullah , GM , Alam , T Hossain , MB . 2009 . Arsenic toxicity to rice (Oryza sativa L.) in Bangladesh . Plant Soil , 317 : 31 – 39 .
- Rahman , MA , Hasegawa , H , Kadohashi , K , Maki , T and Ueda , K . 2009 . Hydroxyiminodisuccinic acid (HIDS): A novel biodegradable chelating ligand for the increase of iron bioavailability and arsenic phytoextraction . Chemosphere , 77 : 207 – 213 .
- Rahman , MA , Hasegawa , H , Mahfuzur Rahman , M , Nazrul Islam , M , Majid Miah , MA and Tasmen , A . 2007a . Effect of arsenic on photosynthesis, growth and yield of five widely cultivated rice (Oryza sativa L.) varieties in Bangladesh . Chemosphere , 67 : 1072 – 1079 .
- Rahman , MA , Hasegawa , H , Rahman , MM , Rahman , MA and Miah , MAM . 2007b . Accumulation of arsenic in tissues of rice plant (Oryza sativa L.) and its distribution in fractions of rice grain . Chemosphere , 69 : 942 – 948 .
- Rahman , MS , Kitajima , N , Sugawara , R , Kondo , T , Huq , SMI and Kawai , S . 2006 . Physiological and mineralogical properties of arsenic-induced chlorosis in rice seedlings grown hydroponically . Soil Sci. Plant Nutr. , 52 : 691 – 700 .
- Rahman , MA , Rahman , MM , Hasegawa , H , Islam , MN , Miah , MAM and Tasmin , A . 2007c . Arsenic accumulation in rice (Oryza sativa L.) varieties of Bangladesh: A glass house study . Water, Air, Soil Pollut. , 185 : 5361
- Raskin , I , Smith , RD and Salt , DE . 1997 . Phytoremediation of metals: using plants to remove pollutants from the environment . Curr. Opin. Biotechnol. , 8 : 221 – 226 .
- Robinson , B , Kim , N , Marchetti , M , Moni , C , Schroeter , L , van den Dijssel , C , Milne , G and Clothier , B . 2006 . Arsenic hyperaccumulation by aquatic macrophytes in the Taupo Volcanic Zone, New Zealand . Environ. Exp. Bot. , 58 : 206 – 215 .
- Romheld , V . 1987 . Different strategies for iron acquisition in higher plants . Physiol. Plant. , 70 : 231 – 234 .
- Romheld , V and Marschner , H . 1986 . Evidence for a specific uptake system for iron phytosiderophores in roots of grasses . Plant Physiol. , 80 : 175 – 180 .
- Schmidt , CK and Brauch , H-J . 2004 . Impact of aminopolycarboxylates on aquatic organisms and eutrophication: Overview of available data . Environ. Toxicol. , 19 : 620 – 637 .
- Schwertmann , U . 1991 . Solubility and dissolution of iron oxides . Plant Soil , 130 : 1 – 25 .
- Stein , RJ , Duarte , GL , Spohr , MG , Lopes , SIG and Fett , JP . 2009 . Distinct physiological responses of two rice cultivars subjected to iron toxicity under field conditions . Ann. Appl. Biol. , 154 : 269 – 277 .
- Takagi , S-I . 1976 . Naturally occurring iron-chelating compounds in oat- and rice-root washings . Soil Sci. Plant Nutr. , 22 : 423 – 433 .
- Tandy , S , Ammann , A , Schulin , R and Nowack , B . 2006 . Biodegradation and speciation of residual SS-ethylenediaminedisuccinic acid (EDDS) in soil solution left after soil washing . Environ. Pollut. , 142 : 191 – 199 .
- Taylor , GJ and Crowder , AA . 1983 . Use of DCB technique for extraction of hydrous iron oxides from roots of wetland plants . Am. J. Bot. , 70 : 1254 – 1257 .
- Tu , C , Ma , LQ and Bondada , B . 2002 . Arsenic accumulation in the hyperaccumulator Chinese brake and its utilization potential for phytoremediation . J. Environ. Qual. , 31 : 1671 – 1675 .
- Vert , G , Grotz , N , Dédaldéchamp , F , Gaymard , F , Guerinot , ML , Briat , JF and Curie , C . 2002 . IRT1, an arabidopsis transporter essential for iron uptake from the soil and for plant growth . Plant Cell , 14 : 1223 – 1233 .
- Wang , F-M , Chen , Z-L , Zhang , L , Gao , Y-L and Sun , Y-X . 2006 . Arsenic uptake and accumulation in rice (Oryza sativa L.) at different growth stages following soil incorporation of roxarsone and arsanilic acid . Plant Soil , 285 : 359 – 367 .
- Wang , H-Q , Lu , S-J , Li , H and Yao , Z-H . 2007 . EDTA-enhanced phytoremediation of lead contaminated soil by Bidens maximowicziana . J. Environ. Sci. , 19 : 1496 – 1499 .
- Xiao , H , Yin , L , Xu , X , Li , T and Han , Z . 2008 . The Iron-regulated transporter, MbNRAMP1, isolated from malus baccata is involved in Fe, Mn and Cd trafficking . Ann. Bot. , 102 : 881 – 889 .
- Xu , XY , McGrath , SP , Meharg , AA and Zhao , FJ . 2008 . Growing rice aerobically markedly decreases arsenic accumulation . Environ. Sci. Technol. , 42 : 5574 – 5579 .
- Zhao , FJ , Ma , JF , Meharg , AA and McGrath , SP . 2009 . Arsenic uptake and metabolism in plants . New Phytol. , 181 : 777 – 794 .