Abstract
Paddy field is a major emission source of methane. Methane is the terminal product of anaerobic decomposition of organic matter and generated by methanogenic archaea under flooded conditions in paddy fields. This study aimed to reveal the effect of winter flooding on methanogenic archaeal community structure in paddy fields of Andosols under organic farming. Soil samples were collected from experimental paddy fields in the Field Science Center, Tohoku University, for two years. They were under flooding conditions during winter with organic farming, under non-flooding conditions during winter with organic farming and under non-flooding conditions during winter with conventional farming (non-organic farming). Polymerase chain reaction-denaturing gradient gel electrophoresis (PCR-DGGE) analysis of methanogenic archaeal 16S rRNA gene revealed that the DGGE patterns were nearly the same irrespective of the treatment and sampling times. Twenty-three bands were observed from each treatment and 4, 13 and 6 sequences were closely related to Methanomicrobiales, Methanosarcinales and Methanocellales, respectively. Real-time quantitative PCR analysis indicated that the abundance of methanogenic archaeal 16S rRNA gene and mcrA gene, encoding α subunit of methyl-coenzyme M reductase, was not significantly different among the paddy fields. This study first revealed a methanogenic archaeal community in an Andosol paddy field and showed that the community was not affected by winter flooding under organic farming.
Introduction
Methane (CH4) is a greenhouse gas and more than 70% of atmospheric methane originates from the activity of methanogenic archaea (Chen and Prinn Citation2005). On a molecular basis, the global warming potential of CH4 is about 25 times greater than that of carbon dioxide (CO2) (Forster et al. Citation2007). Paddy fields are reported to be a major emission source of CH4 (Minami Citation1994). Rice agriculture feeds about a third of the world population. As one of the major biogenic greenhouse gas emission sources the paddy fields emitted almost 5–19% of the total methane budget on Earth (Denman et al. Citation2007). Thus, it is important to reveal the mechanism of methanogenesis in paddy fields. In paddy fields, flooding leads to anaerobic conditions in soil because the diffusion rate of oxygen in the water is extremely low and microbial consumption of oxygen exceeds the diffusion rate. After flooding of paddy fields, oxygen is consumed by aerobic bacteria and chemical oxidation reactions and is depleted fast in most regions of the soil. Methane is the terminal product in the decomposition process of organic materials and is produced by methanogenic archaea, which are strict anaerobes, under reduced conditions. Many studies have focused on the structure of methanogenic archaeal communities in paddy fields. As for phylogenetic diversity of methanogenic archaea, members belonging to Methanomicrobiales, Methanosarcinales, Methanobacteriaceae, and Methanocellales inhabit paddy field soil (Watanabe et al. Citation2010a). A remarkably stable community of methanogenic archaea was demonstrated in paddy field soil, i.e. the structure of methanogenic archaeal communities showed no significant shift in different seasons (Krüger et al. Citation2005), under double cropping (rice [Oryza sativa L.]-wheat [Triticum aestivum L.]) conditions (Watanabe et al. Citation2006), by intermittent drainage (Ma et al. Citation2012), and even by oxygen exposure of soil (Yuan et al. Citation2009).
Organic farming systems use organic fertilizers such as compost and manure with natural control of pests instead of chemical substances such as mineral fertilizers and synthetic pesticides, and arouse a broad interest as a so-called “environment-friendly farming system” (Mäder et al. Citation2002). In Japan, paddy fields under organic farming are often concomitant with flooding during winter season, which aims at enhancing the richness of biological diversity such as waterfowls, increasing the fertility of soil and suppressing weeds (Mineta et al. Citation2009, Akita et al. Citation2012). These paddy fields under organic farming with winter flooding are kept almost flooded in one year, and organic fertilizers are applied to the paddy fields before flooding in winter. These practices could promote anoxic conditions of soil and production of methanogenic substrates in soil (Akita et al. Citation2010b). Methane emission was increased by winter flooding of paddy fields in the rice growing periods or for a year (Fitzgerald et al. Citation2000; Matsumoto et al. Citation2002; Yoshida et al. Citation2010a, Citationb) and significant flux of methane was also reported during the winter season under flooded conditions (Cai et al. Citation2000, Citation2003; Fitzgerald et al. Citation2000). In addition, greater decomposition of rice straw was reported in continuously flooded rice fields than in drained rice fields during winter (Manley et al. Citation2005). Thus, methanogenic archaea possibly increase their activity and may alter their community structure associatively. Lopes et al. (Citation2011) recently reported that the bacterial community was different between rice fields under organic and conventional farming in Portugal, but the fields were drained during winter. The structure of methanogenic archaeal communities in winter-flooded paddy fields with organic farming has not yet been investigated. In this study, therefore, analysis of methanogenic archaeal community structure under rice cultivation was performed in an experimental paddy field with winter flooding under organic farming using molecular approaches of polymerase chain reaction-denaturing gradient gel electrophoresis (PCR-DGGE) and quantitative PCR methods. The objective of the present study was to clarify the effect of winter flooding on methanogenic archaeal community structure in a paddy field under organic farming.
Materials and Methods
Sampling sites and soil samples
The soil samples were collected from an experimental paddy field located in the Field Science Center, Tohoku University, Osaki, Miyagi, Japan (latitude 38°44′ N, longitude 140°45′ E). The experiment of organic farming with winter flooding was begun in 2008. The soil was Anthraquic non-allophanic Kuroboku soil [Aluandic Andosols; total carbon (C) content, 30–34 g kg–1; total nitrogen (N) content, 2.3–2.6 g kg–1; pH [H2O], 5.6–5.7]. The paddy field consisted of three kinds of treatments with two replicate plots: under flooding condition during winter with organic farming (OwF), under non-flooding (drained) condition during winter with organic farming (OwD), and under non-flooding (drained) condition during winter with conventional farming (non-organic farming) (CwD) (). The area of each plot was 300–400 m2.
Figure 1. Water management and fertilizer application in the study fields and the sampling dates. OwF, flooding in winter with organic farming; OwD, non-flooding in winter with organic farming; CwD, non-flooding in winter with conventional farming. CMC, cattle manure compost; CF, chemical fertilizer.
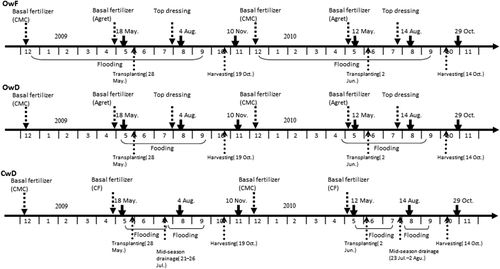
The field was cropped with rice (Oryza sativa L. cv. Hitomebore). Fertilizer application rates were as follows: cattle manure compost (10 t ha–1) was applied to all the plots in early to mid December. A commercial organic fertilizer [“Agret”, Asahi Industries Co., Tokyo, Japan; 40 kg ha–1 N, 40 kg ha–1 phosphorus pentoxide (P2O5) and 40 kg ha–1 potassium oxide (K2O)] was applied to the OwF and OwD plots, and a chemical fertilizer (“Toruzokun”, Coop Chemical Co., Tokyo, Japan; 70 kg ha–1 N, 100 kg ha–1 P2O5 and 70 kg ha–1 K2O) was applied to the CwD plots as a basal fertilizer in late April to early May. For top dressing to the OwF and OwD plots, the organic fertilizer “Agret” (15 kg ha–1 N, 15 kg ha–1 P2O5 and 15 kg ha–1 K2O) was used. In additon, 80 kg ha–1 of rice bran pellets (15 kg ha–1 N, 15 kg ha–1 P2O5 and 15 kg ha–1 K2O) was applied to the OwF and OwD plots for weed control and fertilization. The OwF and OwD plots were flooded in mid December and early May, respectively, and drained in early to mid September. The CwD plots were flooded in late May, and drained for 5–10 days in late July to early August (mid-season drainage) and in early to mid September. The flooding period in a year was about 270, 120–130 and 90–110 d for the OwF, OwD and CwD plots, respectively. Puddling was conducted in late May and rice seedlings were transplanted in late May to early June. Rice plants were harvested in early to mid October, except for the CwD plots in 2010 (late September).
Soil samples were taken from the plow layer with a depth of 5–10 cm at four points in each plot on May 18 (before transplanting), August 4 (during rice cultivation) and November 10 (after harvest of rice) in 2009, and on May 12 (before transplanting), August 14 (during rice cultivation) and October 29 (after harvest of rice) in 2010. The fields were under flooding and drained conditions in August and November or October, respectively. In May, the OwF and OwD fields were flooded and the CwD field was non-flooded. The soil samples were brought to the laboratory in a polyethylene bag and stored at 4°C then passed through a 2-mm mesh sieve and stored at –20°C until use.
DNA extraction and PCR amplification
DNA was extracted from the soil samples using the FastDNA SPIN Kit for soil (MP Biomedicals; Solon, OH, USA) by the bead-beating method with three replicates, according to the manufacture's instructions. The concentration of double-stranded DNA (dsDNA) in soil was determined with PicoGreen dsDNA reagent as described by Lee et al. (Citation2011). PCR amplification was carried out using the primer set 1106F-GC (5′-TTW AGT CAG GCA ACG AGC-3′) and 1378R (5′-TGT GCA AGG AGC AGG GAC-3′), which targeted the methanogenic archaeal 16S rRNA gene, as described by Watanabe et al. (Citation2006). The PCR program had an initial denaturation at 95°C for 90 s, followed by 35 cycles of denaturation at 95°C for 30 s, annealing at 55°C for 30 s and extension at 72°C for 90 s, and a final extension at 72°C for 6 min. The PCR products were observed on agarose gel (2.0%) with 1 × TAE buffer [(40 mM Tris-acetate, 1 mM ethylenediaminetetraacetic acid (EDTA)] and ethidium bromide under UV light.
DGGE, cluster analysis and sequencing
DGGE was performed with a Dcode Universal Mutation Detection System (Bio-Rad Laboratories, Hercules, CA, USA) and the denaturant gradient of the gel was ranged from 32 to 62%, in which 100% denaturant contained 7 M urea and 40% formamide as described by Watanabe et al. (Citation2006). Electrophoresis was run for 14 h at 60°C at 100 V. The gels were stained for 20 min with SYBR Green I nucleic acid gel stain (Lonza, Rockland, ME, USA). The stained gel was immediately photographed under UV light. The cluster analysis was performed for the data obtained from the DGGE band patterns based on the intensity of DGGE bands (0, no band; 1, weak; 2, moderate; 3, strong) using the Black Box Program (Aoki Citation1996).
Representative DGGE bands were carefully excised from the DGGE gel with a 1-mL pipet tip into a 1.5-mL tube and the DNA was eluted by incubating each gel stripe in 50 µL of 1 × TE buffer (10 mM Tris, 1 mM EDTA, pH 8.0) overnight at 4°C. The 1 µL of eluted DNA was used as a template for PCR amplification using the same primer set as described above. The resulting amplicons were electrophoresed again on a DGGE gel to verify their position to match the original bands. This operation was repeated three times until the band appeared to be a single band. The primer without GC clamp (1106F) was used in the template amplification by PCR and the subsequent cycle sequencing using the BigDye Terminator v3.1 Cycle Sequencing Kit (Applied Biosystems Foster City, CA, USA). The products were purified by ethanol precipitation with EDTA buffer. Then the products were run in ABI 3130 automated sequencers (Applied Biosystems). Close relatives and phylogenetic affiliation of the obtained sequences were determined using the Basic Local Alignment Search Tool (BLAST) search program at the DNA Data Bank of Japan (DDBJ) web site. A phylogenetic tree was constructed by 1000-fold bootstrap analysis using the neighbor-joining method with the Molecular Evolutionary Genetic Analysis software (MEGA 4.1; Tamura et al. Citation2007, Kumar et al. Citation2008).
Real-time quantitative polymerase chain reaction
Real-time PCR was carried out to quantify the methanogenic archaeal 16S rRNA gene and mcrA gene, which encodes a key enzyme for methanogens (methyl-coenzyme M reductase α subunit) in soil samples. For 16S rRNA gene, the real-time PCR was conducted with a LightCycler ST300 (Roche Diagnostics, Basel, Switzerland) and the primers 1106F and 1378R were used for the quantification (Watanabe et al. Citation2007). The reaction mixture (20 µL) contained 12.5 µL SYBR Premix ExTaq, primer set 1106F/1378R (50 µM) 0.2 µL each, template DNA 1 µL, and sterilized ultrapure water 8.6 µL. The PCR program consisted of initial denaturation at 95°C for 10 s, followed by 45 cycles of amplification reactions of denaturation at 95°C for 10 s, annealing at 57°C for 10 s and extension at 72°C for 6 s. For mcrA gene, a Thermal Cycler Dice Real Time System (TaKaRa, Otsu, Japan) and the primers (mcrA-f: GGTGGTGTMGGATTCACACARTAYGCWACAGC and mcrA-r: TTCATTGCRTAGTTWGGRTAGTT) (Luton et al. Citation2002) were used for quantification. The real-time PCR was performed in a total volume of 25 µL reaction mixture containing 12.5 µL of SYBR Premix ExTaq, 0.1 µL each of primer set mcrA-f/mcrA-r (50 µM), 1 µL template DNA, and 11.3 µL sterilized ultrapure water. The PCR program was: initial denaturation at 95°C for 30 s, followed by 45 cycles of amplification reactions of denaturation at 95°C for 40 s, annealing at 55°C for 30 s and extension at 72°C for 1 min (Watanabe et al. Citation2010b). The mixture of known numbers (101 to 107 copies) of 16S rRNA gene fragments (Watanabe et al. Citation2007) or mcrA gene fragments (Watanabe et al. Citation2010b) from three methanogenic archaeal strains were used as the standard references. Blanks were always run with water as the template instead of soil DNA extract.
Accession numbers
The GenBank/EMBL/DDBJ accession numbers for the 16S rRNA gene sequences of methanogenic archaea in the present study are AB673134–AB673156.
Results
DGGE banding patterns of methanogenic archaeal communities
The same DGGE band pattern was observed in the three replications of DNA extraction from each plot and there was no difference between the patterns from two replicate plots for each treatment (data not shown). For comparison of the DGGE patterns in the different seasons, all the samples from one of the two replicate plots were subjected to DGGE analysis (). The patterns were almost identical among the OwF, OwD and CwD fields in every sampling period, and 23 bands were observed in the OwF, OwD and CwD fields. There was also no difference in the band patterns of the methanogenic archaeal community in May when the CwD field was drained and the OwF and OwD fields were flooded. Cluster analysis of the patterns showed no specific grouping by plots or sampling periods () and confirmed similarity of the patterns through the two years of the experiment.
Figure 2. Denaturing gradient gel electrophoresis (DGGE) band patterns of methanogenic archaeal 16S rRNA genes obtained from paddy field soils in the Field Science Center of Tohoku University on May 18, August 4, and November 12 in 2009, and May 12, August 14, and October 29 in 2010. OwF, flooding in winter with organic farming; OwD, non-flooding in winter with organic farming; CwD, non-flooding in winter with conventional farming. The numbers indicated by arrows show the bands whose sequences were determined and correspond to the ones on the phylogenetic tree in .
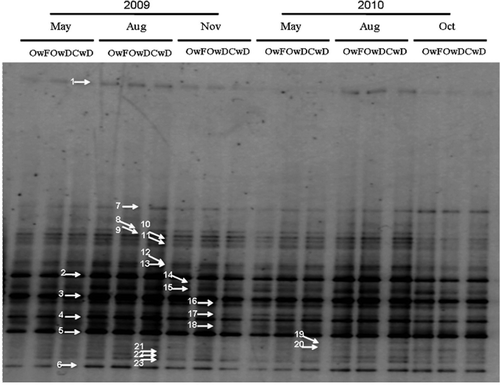
Sequencing and phylogenetic analysis of DGGE bands
The 23 bands were excised from the gel and analyzed (). The sequences from the paddy fields in Chikugo and Anjo (Watanabe et al. Citation2006) were included in the phylogenetic tree. In the present study, four, 13 and six sequences were affiliated to Methanomicrobiales, Methanosarcinales and Methanocellales (Rice cluster-I), respectively (). In the order Methanosarcinales, one band belonged to Methanosarcinaceae, six bands to Methanosaetaceae and six bands to the uncultured group Zoige cluster I (ZC-I) (Zhang et al. Citation2008).
Figure 4. Phylogenetic relationships of the methanogenic archaeal 16S rRNA gene sequences retrieved from denaturing gradient gel electrophoresis (DGGE) bands amplified with 1106F-GC and 1378R from the paddy field soils. The sequences from the paddy field soils in the Field Science Center of Touhoku University are shown in numbers. The sequences from the Chikugo and Anjo paddy fields are shown in bold letters. Aquifex pyrophilus was used as an outgroup. The scale bar represents a 5% sequence difference. The accession numbers of the references are indicated in parentheses.
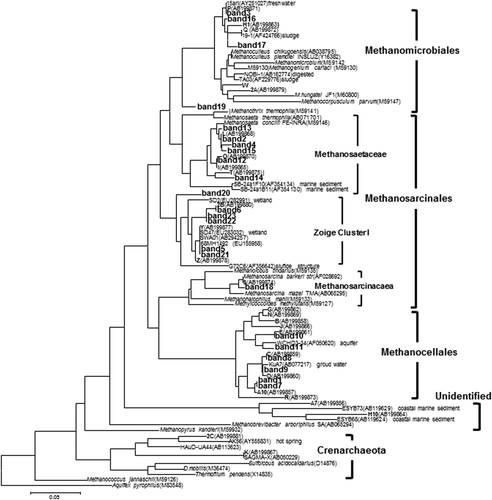
Quantification of methanogenic archaeal numbers
Numbers of 16S rRNA genes (copies g–1 dry soil) ranged from 8.0 × 107 to 1.47 × 108, 9.0 × 107 to 6.4 × 108, and 4.9 × 107 to 3.0 × 108 in OwF, OwD and CwD fields, respectively (a). Numbers of mcrA genes (copies g–1 dry soil) were slightly fewer than those of 16S rRNA gene and ranged from 4.5 × 107 to 4.3 × 108, 6.6 × 107 to 5.2 × 108, and 4.7 × 107 to 4.6 × 108 in OwF, OwD and CwD fields, respectively (b). Apparently, the numbers of 16S rRNA and mcrA genes showed no significant differences among the OwF, OwD and CwD fields and the three sampling periods. The numbers of 16S rRNA genes were positively correlated with those of mcrA genes (r = 0.939; P < 0.01).
Figure 5. The total number of copies of (a) 16S rRNA and (b) mcrA genes of methanogenic archaea in paddy soils. The number shows log number of the copies in 1 g dry soil. OwF, flooding in winter with organic farming; OwD, non-flooding in winter with organic farming; CwD, non-flooding in winter with conventional farming.
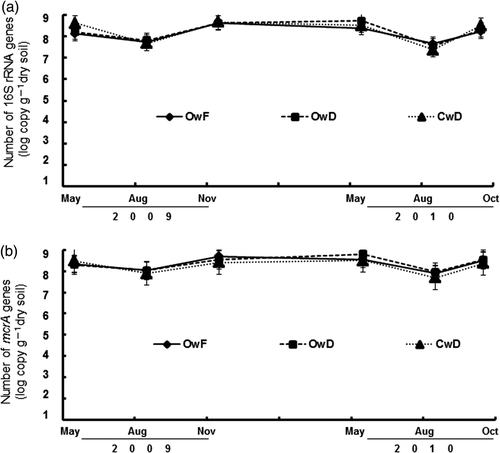
Discussion
In the present study, the paddy rice fields with three different treatments (OwF, OwD and CwD) were investigated. Cluster analysis demonstrated that the DGGE band patterns of 16S rRNA gene were almost identical among the OwF, OwD and CwD fields, irrespective of sampling season, in 2009 and 2010. In addition, the real-time PCR indicated no significant differences in numbers of 16S rRNA and mcrA genes among the OwF, OwD and CwD fields. Therefore, the methanogenic archaeal community was not affected by winter flooding with organic farming in the present study. Because of the low temperature in the present study area in the winter season [monthly average air temperature from December to March was –0.1 to 2.6°C in 2008–2009 and –0.7 to 1.8°C in 2009–2010 at Kawatabi station (38°44′ N, 140°45′ E)] according to the Japan Meteorological Agency (2011), the organic materials in OwF field during winter may have been scarcely decomposed. This may be a reason why the methanogenic archaeal community showed no difference among the OwF, OwD and CwD fields. In addition, good survivability of methanogenic archaea and stability of their community in soil (Asakawa and Hayano Citation1995; Asakawa et al. Citation1998; Watanabe et al. Citation2006, Citation2007) should be taken into consideration. Watanabe et al. (Citation2006) reported that the methanogenic archaeal community was extremely stable through one year irrespective of flooded or drained conditions in the fields with double cropping, and also showed that the effect of long-term application of organic matter (rice straw, wheat straw or rice straw compost) on the community was small. The present study also revealed the methanogenic archaeal community was not influenced by application of organic fertilizers in the organic farming system.
Before transplanting, a significant amount of CH4 was emitted from the OwF field during the late stages of winter flooding from April to May in 2010, while no emission was observed from the OwD and CwD fields (Akita 2011, unpublished results). This may be due to the development of soil reduction in the OwF field. Concentrations of ferrous iron (Fe2+) and ammonium ions () in soil were significantly higher in the OwF field than in the OwD and CwD fields (Akita et al. 2010a, b) and vice versa for the Eh (oxidation-reduction potential) values of soil (Akita 2011, unpublished results). Increase in air temperature during the above period (April and May, monthly average, 1.4 to 9.7°C) may promote decomposition of organic materials and reduction of soil in the OwF. Furthermore, seasonal emission of CH4 from the OwF field was also significantly higher than those from the OwD and CwD fields in the rice cultivation period in 2010 (Akita 2011, unpublished results). In fact, Watanabe et al. (Citation2009) found that different members in a stable methanogenic archaeal community transcribed mcrA genes during the annual cycle of rice-wheat rotation depending on the soil conditions. Consequently, winter flooding promoted methane production without any effects on community structure of methanogenic archaea, but their active members may have varied depending on the conditions of soil under different treatments in the studied fields.
In the present study, Methanomicrobiales, Methanosarcinales and Methanocellales (Rice cluster-I) were the dominant groups, which corresponded with the findings from other regions in Japan (Anjo and Chikugo; Watanabe et al. Citation2006) and China (Wang et al. Citation2010). Methanosarcinales was the main order in the present study and approximately a half of the sequences were comprised in this order. At the family level, one, six, and six out of 13 sequences of Methanosarcinales members were affiliated to Methanosarcinaceae, Methanosaetaceae and uncultured group Zoige cluster I (ZC-I), respectively. Methanogenic archaea utilize three major substrates: hydrogen (H2)/CO2, methyl compounds, and acetate (Liu and Whitman Citation2008). Only members of Methanosarcinaceae and Methanosaetaceae can utilize acetate. Members of the Methanosarcinaceae favor higher concentrations of acetate, while those of the Methanosaetaceae are prevalent at lower concentrations (Fey and Conrad Citation2000; Krüger et al. Citation2005). Methanosarcinaceae are dominant when acetate concentration is extremely high in the early stage of decomposition of organic materials. In contrast, when acetate is almost consumed, Methanosaetaceae instead of Methanosarcinaceae are dominant at the last stage of decomposition (Peng et al. 2008). Methanosaetaceae prevailed over Methanosarcinace in the present study, and this might be due to low concentration of acetate in the paddy field soil, although acetate concentrations were not measured in this study. Zoige cluster I (ZC-I) was another dominant group of Methanosarcinales in the present study. This group was observed from a wetland of the Tibetan plateau under cold conditions (annual temperature around 1°C) and assumed to utilize acetate, H2/CO2, methanol or trimethylamine, but not formate (Zhang et al. Citation2008). Thus, Zoige cluster I (ZC-I) might have priority to utilize these substrates and played an important role in the studied paddy field soil.
The real-time PCR indicated that the numbers of 16S rRNA genes were higher than those of mcrA genes. The 16S rRNA gene could be present as multiple copies in the bacterial chromosome (Masco et al. Citation2007), and this may have affected the results. Correlation analysis showed a linear positive relationship between numbers of the two genes. However, the results were not identical with the findings of Wang et al. (Citation2010), in which the numbers of the 16S rRNA gene were 10 fold higher than those of the mcrA gene. In the present study, all of the excised DGGE bands belonged to methanogenic archaea. On the other hand, only 85.7% and 84.6% of the excised DGGE bands by Wang et al. (Citation2010) and Watanabe et al. (Citation2006) were affiliated to methanogenic archaea, where the same primer set (1106F-GC and 1378R) as in the present study was used for the PCR amplification. This may also have influenced the quantification of the 16S rRNA gene of methanogenic archaea.
Furthermore, other possible factors which may have affected the data were regions or soil type. Some differences in methanogenic archaeal communities in rice field soil from different regions were found (Ramakrishnan et al. Citation2001). In the studied field, the soil type was Andosol. Andosols possess many unique chemical and physical properties such as high porosity and small bulk density (Shoji et al. Citation1993; Nanzyo Citation2002), which are favorable for keeping aerobic conditions. The number of mcrA gene copies (107–108 copies g–1 soil) in the paddy fields in the present study was comparable with the number in paddy fields with alluvial lowland soils (non-volcanic soils) reported by Watanabe et al. (Citation2007), although the number of 16S rRNA genes in the present study was one order smaller than the number obtained by Watanabe et al. (Citation2007).
The micro-environments in Andosols may be appropriate for a number of methanogenic archaea to survive. In addition, this study first revealed methanogenic archaeal communities in paddy fields of Andosols and also showed that they survived without a drastic shift in the paddy field for two years as reported in non-volcanic paddy field soils. Members of the Methanobacetriaceae were easily enriched from paddy field soil by cultivation with hydrogen, probably due to the relatively lower affinity for H2 (Sakai et al. Citation2009), and were also found as inhabitants in paddy field soil by molecular analyses though they were not always a predominant group (Watanabe et al. Citation2010a). In the present study, however, no sequence of Methanobacteriaceae was obtained (), which may also be attributable to the properties of Andosols. Further investigation is needed for methanogenic archaeal communities in Andosol paddy fields.
In conclusion, the structure of the methanogenic archaeal community was stable through two years and did not show drastic differences among OwF, OwD and CwD fields. The numbers of 16S rRNA and mcrA genes also exhibited no significant difference among OwF, OwD and CwD fields. Thus, the methanogenic archaeal community was not affected by the management of winter flooding, though methane production was promoted by the treatment.
Acknowledgments
This study was supported in part by a Grant-in-Aid (Soil eDNA) from the Ministry of Agriculture, Forestry and Fisheries of Japan and by a Grant-in-Aid (C; 23580089) from the Japan Society for the Promotion of Science.
References
- Akita , K , Ito , T and Uno , T . 2010a . Effects of winter-flooding with organic farming on soil nutrients and methane emission from paddy field . Abst. Meet. Jpn. Soc. Soil Sci. Plant Nutr. , 56 : 103 (in Japanese)
- Akita , K , Ito , T and Uno , T . 2010b . Effects of winter-flooding on soil nutrients and rice yield in paddy field with organic farming . J. Integ. Field Sci. , 7 : 113
- Akita , K , Uno , T , Suzuki , K , Tajima , R , Saito , M and Ito , T . 2012: Aquatic biota in winter flooded paddy field with organic farming: Case study in Field Science Center, Tohoku University, Japan. J. Integ. Field Sci., 9, in press
- Aoki , S . 1996: Black-box: Data analysis on the WWW. http://www.aoki2.si.gunma-u.ac.jp/BlackBox/BlackBox.html (January, 2012)
- Asakawa , S , Akagawa-Mastushita , M , Morii , H , Koga , Y and Hayano , K . 1998 . Communities of methanogenic bacteria in paddy field soils with long-term application of organic matter . Soil Biol. Biochem. , 30 : 299 – 303 .
- Asakawa , S and Hayano , K . 1995 . Population of methanogenic bacteria in paddy field soil under double cropping conditions (rice-wheat) . Biol. Fertil. Soils , 20 : 113 – 117 .
- Cai , ZC , Tsuruta , H , Gao , M , Xu , H and Wei , C . 2003 . Option for mitigation methane emission from a permanently flooded rice field . Global Change Biol. , 9 : 37 – 45 .
- Cai , ZC , Tsuruta , H and Minami , K . 2000 . Methane emission from rice fields in China: Measurements and influencing factors . J. Geophys. Res. , 105 : 17231 – 17242 .
- Chen , Y-H and Prinn , RG . 2005 . Atmospheric modeling of high- and low-frequency methane observations: Importance of interannually varying transport . J. Geophys. Res. , 110 : D10303 doi:10.1029/2004JD005542
- Denman , KL , Brasseur , G Chidthaisong , A . 2007 . “ Couplings between changes in the climate system and biogeochemistry ” . In Climate Change 2007: The Physical Science Basis. Contribution of Working Group I to the Fourth Assessment Report of the Intergovernmental Panel on Climate Change , Edited by: Solomon , S , Qin , D , Manning , M , Chen , Z , Marquis , M , Avery , KB , Tignor , M and Miller , HL . 499 – 587 . Cambridge , UK : Cambridge University Press .
- Fey , A and Conrad , R . 2000 . Effect of temperature on carbon and electron flow and on the archaeal community in methanogenic rice field soil . Appl. Environ. Microbiol. , 66 : 4790 – 4797 .
- Fitzgerald , GJ , Scow , KM and Hill , JE . 2000 . Fallow season straw and water management effects on methane emissions in California rice . Global Biogeochem. Cycles , 14 : 767 – 776 .
- Forster , P , Ramaswamy , V Artaxo , P . 2007 . “ Changes in atmospheric constituents and in radiative forcing ” . In Climate Change 2007: The Physical Science Basis. Contribution of Working Group I to the Fourth Assessment Report of the Intergovernmental Panel on Climate Change , Edited by: Solomon , S , Qin , D , Manning , M , Chen , Z , Marquis , M , Avery , KB , Tignor , M and Miller , HL . 129 – 234 . Cambridge , UK : Cambridge University Press .
- Japan Meteorological Agency 2011: Weather Observations, Temperature. http://www.jma.go.jp/jp/amedas (September, 2011)
- Krüger , M , Frenzel , P , Kemnitz , D and Conrad , R . 2005 . Activity, structure and dynamics of the methanogenic archaeal community in a flooded Italian rice field . FEMS Microbiol. Ecol. , 51 : 323 – 331 .
- Kumar , S , Dudley , J , Nei , M and Tamura , K . 2008 . MEGA: A biologist-centric software for evolutionary analysis of DNA and protein sequences . Brief. Bioinform. , 9 : 299 – 306 .
- Lee , CG , Watanabe , T , Sato , Y , Murase , J , Asakawa , S and Kimura , M . 2011 . Bacterial populations assimilating carbon from 13C-labeled plant residue in soil: Analysis by a DNA-SIP approach . Soil Biol. Biochem. , 43 : 814 – 822 .
- Lopes , AR , Faria , C , Prieto-Fernández , Á , Trasar-Cepeda , C , Manaia , CM and Nunes , OC . 2011 . Comparative study of the microbial diversity of bulk paddy soil of two rice fields subjected to organic and conventional farming . Soil Biol. Biochem. , 43 : 115 – 125 .
- Liu , Y and Whitman , WB . 2008 . Metabolic, phylogenetic, and ecological diversity of the methanogenic archaea . Ann. N.Y. Acad. Sci. , 1125 : 171 – 189 .
- Luton , PE , Wayne , JM , Sharp , RJ and Riley , PW . 2002 . The mcrA gene as an alternative to 16S rRNA in the phylogentic analysis of methanogen populations in landfill . Microbiol. , 148 : 3521 – 3530 .
- Ma , K , Conrad , R and Lu , YH . 2012 . Responses of methanogen mcrA genes and their transcripts to an alternate dry/wet cycle of paddy field soil . Appl. Environ. Microbiol. , 78 : 445 – 454 .
- Mäder , P , Fließbach , A , Dubois , D , Gunst , L , Fried , P and Niggli , U . 2002 . Soil fertility and biodiversity in organic farming . Science , 296 : 1694 – 1697 .
- Manley , SW , Kaminski , RM , Reinecke , KJ and Gerard , PD . 2005 . Agronomic implications of waterfowl management in Mississippi ricefields . Wildlife Soc. Bull. , 33 : 981 – 992 .
- Masco , L , Vanhoutte , T , Temmerman , R , Swings , J and Huys , G . 2007 . Evaluation of real-time PCR targeting the 16S rRNA and recA genes for the enumeration of bifidobacteria in probiotic products . Int. J. Food Microbiol. , 113 : 315 – 357 .
- Matsumoto , J , Minamiyama , Y , Akahori , S and Takahashi , K . 2002 . Suppression of methane emission from paddy field supplemented with organic matter . Jpn. J. Soil Sci. Plant Nutr. , 73 : 315 – 318 (in Japanese) .
- Minami , K . 1994 . Methane from rice production . Fertil. Res. , 37 : 167 – 179 .
- Mineta , T , Koizumi , N and Ishida , K . 2009 . Contribution of winter flooding in multifunctionality of paddy fields: Some notes in a case of biota conservation function in Tajiri-Shinpo, Miyagi prefetture . J. Rural Plan. Assoc. , 27 : 335 – 340 . (in Japanese with English summary)
- Nanzyo , M . 2002 . Unique properties of volcanic ash soils . Glob. Environ. Res. , 6 : 99 – 112 .
- Peng , J , Lu , Z , Rui , J and Lu , Y . 2008 . Dynamics of methanogenic archaeal community during plant residue decomposition in an anoxic rice field soil . Appl. Environ. Microbiol. , 74 : 2894 – 2901 .
- Ramakrishnan , B , Lueders , T , Dunfield , PF , Conrad , R and Friedrich , MW . 2001 . Archaeal community in rice soils from different geographical regions before and after initiation of methane production . FEMS Microbiol. Ecol. , 37 : 175 – 186 .
- Sakai , S , Imachi , H , Sekiguchi , Y , Tseng , I-C , Ohashi , A , Harada , H and Kamagata , Y . 2009 . Cultivation of methanogens under low-hydrogen conditions by using the coculture method . Appl. Environ. Microbiol. , 75 : 4892 – 4896 .
- Shoji , S , Nanzyo , M and Dahlgren , RA . 1993 . Volcanic ash soils: Genesis, properties and utilization . Dev. Soil Sci. , 21 : 1 – 288 .
- Tamura , K , Dudley , J , Nei , M and Kumar , S . 2007 . MEGA4: Molecular Evolutionary Genetics Analysis (MEGA) software version 4.0 . Mol. Biol. Evol. , 24 : 1596 – 1599 .
- Wang , G , Watanabe , T , Jin , J , Liu , X , Kimura , M and Asakawa , S . 2010 . Methanogenic archaeal communities in paddy field soils in northeast China as evaluated by PCR-DGGE, sequencing and real-time PCR analyses . Soil Sci. Plant Nutr. , 56 : 831 – 838 .
- Watanabe , T , Kimura , M and Asakawa , S . 2006 . Community structure of methanogenic archaea in paddy field soil under double cropping (rice-wheat) . Soil Biol. Biochem. , 38 : 1264 – 1274 .
- Watanabe , T , Kimura , M and Asakawa , S . 2007 . Dynamics of methanogenic archaeal communities based on rRNA analysis and their relation to methanogenic activity in Japanese paddy field soils . Soil Biol. Biochem. , 39 : 2877 – 2887 .
- Watanabe , T , Kimura , M and Asakawa , S . 2009 . Distinct members of a stable methanogenic archaeal community transcribe mcrA genes under flooded and drained conditions in Japanese paddy field soil . Soil Biol. Biochem. , 41 : 276 – 285 .
- Watanabe , T , Kimura , M and Asakawa , S . 2010a . Diversity of methanogenic archaeal communities in Japanese paddy field ecosystem, estimated by denaturing gradient gel electrophoresis . Biol. Fertil. Soils , 46 : 343 – 353 .
- Watanabe , T , Wang , G , Taki , K , Ohashi , Y , Kimura , M and Asakawa , S . 2010b . Vertical changes in bacterial and archaeal communities with soil depth in Japanese paddy fields . Soil Sci. Plant Nutr. , 56 : 705 – 715 .
- Yoshida , O , Fujiwara , S , Ushiyama , K , Imai , S and Kubota , C . 2010a . Methane emission from early pooled and organic paddy rice field around the lake Miyajimanuma in Bibai city, Hokkaido . J. Rakuno Gakuen Univ. , 34 : 211 – 221 . (in Japanese with English summary)
- Yoshida , O , Sawamoto , T , Fujiwara , S , Kobayashi , K , Imai , S , Kubota , C , Okazaki , Y , Ogimoto , H and Ushiyama , K . 2010b . Methane emission of early pooled and organic paddy rice field around the lake Miyajimanuma in Bibai city, Hokkaido during 2008–2009 . J. Rakuno Gakuen Univ. , 35 : 93 – 102 . (in Japanese with English summary)
- Yuan , YL , Conrad , R and Lu , YH . 2009 . Response of methanogenic archaeal community to oxygen exposure in rice field soil . Environ. Microbiol. Rep. , 1 : 347 – 354 .
- Zhang , G , Tian , J , Jiang , N , Guo , X , Wang , Y and Dong , X . 2008 . Methanogen community in Zoige wetland of Tibetan Plateau and phyentype characterization of a dominant uncultured methanogen cluster ZC-I . Environ. Microbiol. , 10 : 1850 – 1860 .