Abstract
The need for salt-tolerant turfgrasses in arid and semi-arid regions has increased substantially owing to shortages of fresh water and to soil salinization. We hypothesized that salt tolerance in turfgrasses is achieved by ion regulation and the accumulation of compatible solutes. We investigated changes in ionic concentrations and compatible solutes in Kentucky bluegrass (Poa pratensis L.; a salt-sensitive species) and Tall fescue (Festuca arundinacea Schreb.; a moderately salt-tolerant species) in response to elevated sodium chloride (NaCl) concentration. Sodium (Na+) and chloride (Cl–) concentrations in shoots and roots increased with increasing salinity in both turfgrasses. Kentucky bluegrass accumulated more Na+ and Cl− under NaCl stress than did Tall fescue. NaCl stress induced more significantly mineral nutrient imbalances in Kentucky bluegrass than in Tall fescue. The concentrations of potassium (K+), calcium (Ca2+), magnesium (Mg2+) and nitrate in Tall fescue were much less affected than those in Kentucky bluegrass. The proline concentration increased significantly in both grasses with increasing salinity. The total soluble sugar (TSS) concentration of Tall fescue was significantly higher than that of Kentucky bluegrass under elevated NaCl concentration. Inorganic ions, especially Na+, were more important in osmoregulation for Kentucky bluegrass and Tall fescue to adapt to NaCl stress. The estimated contribution of K+ to osmotic potential (Ψs) decreased with increasing salinity, and it was higher in Tall fescue than in Kentucky bluegrass. The estimated contribution of TSS to Ψs was higher in Tall fescue than in Kentucky bluegrass under NaCl stress. These results indicate that Tall fescue tolerates salt better by inhibiting the accumulation of Na+ and Cl−, which helps to maintain ion balance, and by accumulating sugars, which decrease the osmotic potential of the cytoplasm.
Introduction
Salt stress is one of the most significant factors limiting crop productivity. About 20% of the world's cultivated land and nearly half of all irrigated soils are affected by salinization (Zhu Citation2001). Salt problems on golf courses are also becoming more pronounced especially in arid and semi-arid regions. One of the reasons is that turfgrasses are increasingly being irrigated with saline water as fresh water shortages are occurring with expanding population growth and urbanization (Marcum Citation2006). The use of effluent or other secondary, often saline, water sources for golf courses irrigation has increased dramatically and will accelerate in the future. A 1978 survey reported that 26 golf courses across the USA were using recycled wastewater. However, the National Golf Foundation (NGF) of America reported that approximately 13% of golf courses (approximately 2000 golf courses) nationwide used recycled water for irrigation in 2003 (Qian and Harivandi Citation2008). Seawater intrusion in coastal areas has also added to the salinity problems on turfgrass (McCarty and Dudeck Citation1993; Marcum Citation2006). For these reasons, the need for turfgrass that can tolerate stresses associated with salt-affected sites and even irrigation with recycled wastewater has increased in recent decades.
Salt can harm turfgrasses in various ways. Direct effects include osmotic stress, ion toxicity, nutrient imbalance, membrane dysfunction, and inhibition of metabolic processes such as photosynthesis and respiration (Orcutt and Nilsen Citation2000). Specific effects on plant metabolism, especially in leaf senescence, have been related to the accumulation of toxic ions [sodium (Na+) and chloride (Cl−)] or to potassium (K+) and calcium (Ca2+) depletion (Demiral and Türkan Citation2005). Salt tolerance has been associated with ion regulation in many plants (Flowers and Colmer Citation2008; Chen et al. Citation2009). To mitigate ion injury, many plants maintain a low Na+ concentration in shoots by accumulating Na+ in the roots and preventing its translocation to shoots (Yamanouchi et al. Citation1997). The maintenance of intracellular K+/Na+ homeostasis is crucial for salt tolerance (Zhu Citation2002, Citation2003).
Salt-tolerant plants accumulate inorganic ions and various organic osmolytes to balance external osmotic stresses through the process of osmoregulation, or osmotic adjustment. To avoid toxicity to the cytosol, Na+ and Cl− are compartmentalized mainly in the vacuoles of shoot and root cells (Flowers et al. Citation1977; Lerner et al. Citation1994). Under these conditions, the osmotic potential of the cytoplasm is maintained by the accumulation of compatible solutes such as proline, glycinebetaine, sugars, and cyclitols, which can be accumulated in sufficient concentrations to balance the osmotic potential of Na+ and Cl− accumulated in the vacuole (Flowers and Colmer Citation2008). Glycinebetaine and proline typically accumulate in turfgrasses and other grasses (Marcum Citation2008).
Turfgrasses exhibit a wide range of salinity tolerance. An understanding of the mechanisms of salinity tolerance can aid the development of superior salt-tolerant cultivars, and of drainage water reuse schemes and land reclamation under saline conditions. Kentucky bluegrass (KBG; Poa pratensis L.), a salt-sensitive C3 species with an average electrical conductivity (EC) threshold of 3 dS m−1 (Carrow and Duncan Citation1998), is widely used for lawn, golf fairway, sports field, and other general-purpose turf from subarctic to temperate regions (Turgeon Citation2008). Tall fescue (TF; Festuca arundinacea Schreb.), a moderately salt-tolerant C3 species with an EC threshold of 6–10 dS m−1 (Harivandi Citation1988), is becoming an increasingly important lawn species and is widely used as a utility turfgrass in both warm and cool subtropical areas (Turgeon Citation2008). Several studies have assessed the effects of high salinity on KBG and TF (Horst and Beadle Citation1984; Qian et al. Citation2001; Suplick-Ploense et al. Citation2002; Wipff and Rose-Fricker Citation2003). However, the contributions of specific inorganic ions and compatible solutes to osmotic potential under saline conditions are not clear.
Salt-tolerant plants can accumulate inorganic ions, especially Na+ and Cl−, and synthesize compatible solutes for reducing osmotic potential in saline soil, and thus they can absorb water to support growth (Bohnert and Shen Citation1999). Recent research has shown that C4 turfgrass [bermudagrass (Cynodon dactylon × C. transvaalensis Burtt-Davey), zoysiagrass (Zoysia Willd.) and St. Augustinegrass (Stenotaphrum secundatum Walt.)] can adjust to saline growing media by complete osmotic adjustment (Marcum and Murdoch Citation1994). Salt stress inhibits the growth of KBG and TF (Alshammary et al. Citation2004). However, osmotic adjustment of KBG and TF is not reported yet. Qian et al. (Citation2001) assayed two KBG cultivars for compatible solutes; they found proline at concentrations too low for osmotic adjustment, and no glycinebetaine. We hypothesized that the degree of salt tolerance of KBG and TF is related to ion regulation and sugar accumulation. Understanding the physiological and biochemical processes of salinity adaptation could help us to choose salt-tolerant turfgrass cultivars that can be irrigated with saline water.
The aim of the present study was to compare the inorganic ion concentrations, compatible solute accumulation and the contributions of specific inorganic ions and compatible solutes to osmotic potential in shoots and roots of KBG and TF in response to different levels of sodium chloride (NaCl) stress.
Materials and methods
Plant materials and salt stress treatments
Seeds of KBG (Poa pratensis L. cv. Blue Star) and TF (Festuca arundinacea Schreb. cv. Little Hero) were sterilized in 0.5% (w/v) sodium hypochlorite (NaClO) and placed on a magnetic stirrer for 10 min before being thoroughly rinsed with distilled water and then sown in trays containing vermiculite in a growth chamber. The air temperature was maintained at 24 ± 2°C and the relative humidity (RH) at 60%. Three-week-old seedlings, uniform in size and development, were transplanted into 4-L plastic pots (five plants per pot) in a glasshouse. The composition of the nutrient solution was as follows: (mmol L−1) 2.0 N (NH4NO3), 0.4 P (NaH2PO4), 2.0 K (KCl), 1.0 Ca (CaCl2 · 2H2O) and 2.0 Mg (MgSO4 · 7H2O) for the macronutrients, and (mg L−1) 2.0 Fe (FeSO4 · 7H2O), 0.5 Mn (MnSO4 · 5H2O), 0.2 B (H3BO3), 0.1 Zn (ZnSO4 · 7H2O), 0.01 Cu (CuSO4 · 7H2O) and 0.005 Mo (NH4)6Mo7O24 · 4H2O for the micronutrients. The pH of the solution was adjusted to 6.5 with H2SO4 or NaOH. The solution was aerated constantly and replaced twice a week throughout the experiment. The temperature in the glasshouse during the experiment was 20–32°C and the RH was 60–70%.
Plants were grown under non-saline conditions for 15 d to ensure full establishment before salt treatments were started. Five salinity levels of 0 (control), 50, 100, 150, and 200 mmol L−1 (1.1, 6.7, 12.0, 17.2 and 21.6 dS m−1) were prepared by dissolving NaCl in nutrient solution according to the relative salinity tolerance of KBG and TF (Torello and Symington Citation1984; Alshammary et al. Citation2004; Kobayashi et al. Citation2004). To avoid osmotic shock, salinity was gradually increased by 50 mmol L−1 per day until a final salinity level of 200 mmol L−1 NaCl was reached. Afterwards, plants in each treatment were grown for 40 d. After harvest, shoots and roots were washed with deionized water and dried at 70°C for 48 h for determining inorganic concentrations and TSS.
Osmotic potential
Shoot and root samples were collected from three plants, thoroughly rinsed, cut into small pieces (5 mm in length), placed in Eppendorf tubes perforated with four small holes, and immediately frozen in liquid nitrogen. After being encased individually in a second intact Eppendorf tube, they were allowed to thaw for 30 min and centrifuged at 15,000 × g for 20 min at 4°C. The osmolality of the collected sap was analyzed with a vapor pressure osmometer (Osmometer 5520, Wescor, Logan, UT, US). The Ψs was calculated using the van't Hoff equation (Ψs = −cRT), where c is the osmolality (mmol kg−1), R is the gas constant, and T is the temperature (K) (Nobel Citation1991).
Ionic concentrations
To determine the concentrations of Na+, K+, Ca2+, and Mg2+, 50 mg of ground shoot and root samples were digested in 1 mL sulfuric acid and hydrogen peroxide at 200°C on a dry block bath. The concentrations were determined by atomic absorption spectrophotometry (Polarization Zeeman Z-6100; Hitachi, Ibaraki, Japan). To determine the concentrations of Cl− and nitrate , 100 mg of ground plant samples were heated in 10 mL boiling deionized water in a beaker on a hot plate (250°C) for 1 min, then we immediately filtered the extract through a Toyo No. 6 filter paper. Chloride and
were determined using an ion chromatography (CDD-10A SP, HIC-10A Super; Shimadzu, Kyoto, Japan).
Total soluble sugars and proline
TSS was extracted as described by Angelov et al. (Citation1993). Briefly, 0.25 g of dry sample was extracted in 5 mL 80% (v/v) ethanol in a boiling water bath for 10 min and centrifuged at 10,000 × g for 15 min. The pellet was re-extracted several times with 80% ethanol until the extract became colorless. The ethanol-soluble fractions from each sample were pooled, dried at 55°C under vacuum, redissolved in 100 mL of distilled water, and frozen (−20°C) until analysis. TSS was estimated by the method of McCready et al. (Citation1950) using 10.3 mmol L−1 anthrone in concentrated H2SO4. Spectrophotometric readings were taken at 630 nm. A standard curve was plotted with 0–200 ppm of glucose.
The proline concentration was determined by the method of Bates et al. (Citation1972). Samples were ground in a mortar and homogenized in 120 mmol L−1 sulfosalicylic acid, and the homogenate was centrifuged at 10,000 × g for 15 min. The supernatant was mixed with 2 mL acid ninhydrin and 2 mL glacial acetic acid and then boiled at 100°C for 1 h. The reaction was stopped by cooling in an ice bath, and the sample was extracted with 4 mL toluene. The absorbance of the organic phase was determined at 520 nm.
Contribution of specific inorganic ions and compatible solute to osmotic potential
The osmotic potential of each of Na+, K+, Cl−, proline, and TSS was calculated as
Statistical analyses
The experiment was set up as a completely randomized design with both species and five salinity levels. The effects of species, salinity levels, and their interactions on variables were analyzed by two-way analysis of variance (ANOVA) with SPSS version 10.0J software (SPSS Japan, Inc.). When significant differences were detected at P = 0.05, mean values were compared by Duncan's multiple range tests.
Results
Ion concentrations in shoots and roots
Shoot dry weights of both species and root dry weight of KBG decreased significantly with increasing NaCl concentration (Xu et al. 2013). However, there was not a significant difference in the root dry weight of TF between 100, 150 and 200 mmol L−1 NaCl. The decrease of shoot and root dry weight in KBG was more drastic than that in TF under salinity treatment. These results indicated that growth inhibition by NaCl was more severe in KBG than in TF.
Na+ in the shoots of KBG and TF increased as salinity increased (); that in KBG increased significantly relative to the control. That in TF did not increase above 100 mmol L−1 NaCl. Na+ was 30% to 122% higher in KBG than in TF at 50 to 200 mmol L−1 NaCl. K+ in the shoots of KBG decreased as NaCl increased (). However, that of TF decreased from 0 to 100 mmol L−1 NaCl and increased slightly at 150 and 200 mmol L−1 NaCl. K+ was higher in TF than in KBG at the same salinity. Ca2+ and Mg2+ in the shoots of KBG and TF decreased as NaCl increased (); concentrations of these cations did not differ significantly between grasses except at 100 mmol L−1 NaCl.
Figure 1. Cation concentrations in the shoots of Kentucky bluegrass (KBG; Poa pratensis L.) and Tall fescue (TF; Festuca arundinacea Schreb.) at different sodium chloride (NaCl) concentrations. (A) sodium (Na+), (B) potassium (K+), (C) calcium (Ca2+), and (D) magnesium (Mg2+). Bars indicate standard error (n = 3). Different lower case letters indicate significant differences between means at P = 0.05 according to Duncan's multiple range tests. DW, dry weight.
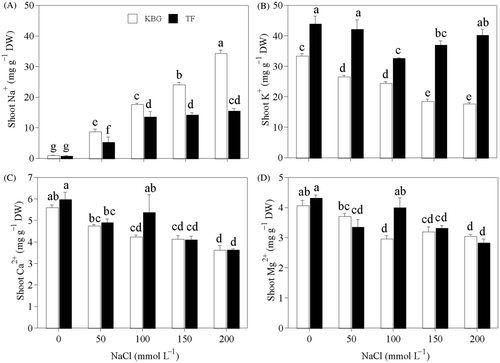
Cl− in the shoots of KBG increased as salinity increased (). That of TF did not increase above 50 mmol L−1 NaCl (). Cl− was higher in KBG than in TF, except at 50 mmol L−1 NaCl. in the shoots of KBG decreased as salinity increased, but did not decrease significantly above 100 mmol L−1 NaCl (). That of TF decreased gradually as salinity increased ().
of shoots was 58% to 111% higher in TF than in KBG at 50 to 200 mmol L−1 NaCl.
Figure 2. Anion concentrations in the shoots of Kentucky bluegrass (KBG; Poa pratensis L.) and Tall fescue (TF; Festuca arundinacea Schreb.) at different sodium chloride (NaCl) concentrations. (A) chlorine (Cl−) and (B) nitrate (). Bars indicate standard error (n = 3). Different lower case letters indicate significant differences between means at P = 0.05 according to Duncan's multiple range tests. DW, dry weight.
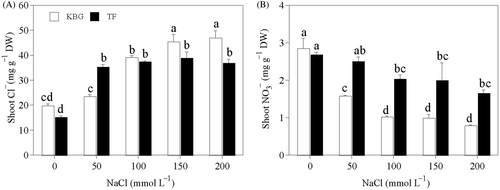
Na+ in the roots of KBG and TF increased significantly as salinity increased, but that in KBG was significantly higher than in TF (). Na+ was 36% to 59% higher in KBG than in TF at 50 to 200 mmol L−1 NaCl. K+ in the roots of KBG and TF decreased as salinity increased (). K+ was higher in TF than in KBG at all salinities. Ca2+ in the roots of KBG and TF did not change significantly under NaCl stress, except in KBG at 200 mmol L−1 (). Mg2+ in the roots of KBG under salt stress was less than that in the control (); however, that in TF increased slightly at NaCl concentrations above 50 mmol L−1 ().
Figure 3. Cation concentrations in the roots of Kentucky bluegrass (KBG; Poa pratensis L.) and Tall fescue (TF; Festuca arundinacea Schreb.) at different sodium chloride (NaCl) concentrations. (A) sodium (Na+), (B) potassium (K+), (C) calcium (Ca2+), and (D) magnesium (Mg2+). Bars indicate standard error (n = 3). Different lower case letters indicate significant differences between means at P = 0.05 according to Duncan's multiple range tests. DW, dry weight.
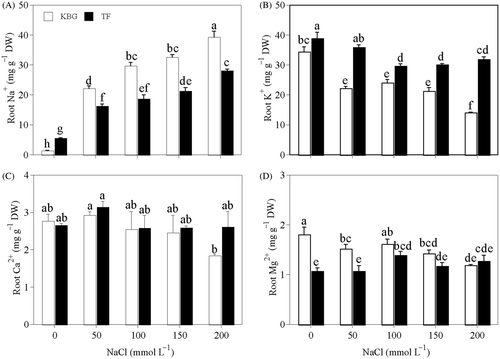
Cl− in the roots of KBG and TF increased significantly as salinity increased (). Cl− in KBG increased by 117% to 191% and that in TF increased by 39% to 99% at 50 to 200 mmol L−1 NaCl. Under salt stress Cl− was higher in KBG than in TF. in the roots of KBG and TF decreased significantly as salinity increased (). The
of root was 22% to 63% higher in TF than in KBG at 50 to 200 mmol L−1 NaCl.
Figure 4. Anion concentrations in the roots of Kentucky bluegrass (KBG; Poa pratensis L.) and Tall fescue (TF; Festuca arundinacea Schreb.) at different sodium chloride (NaCl) concentrations. (A) chlorine (Cl−) and (B) nitrate (). Bars indicate standard error (n = 3). Different lower case letters indicate significant differences between means at P = 0.05 according to Duncan's multiple range tests. DW, dry weight.
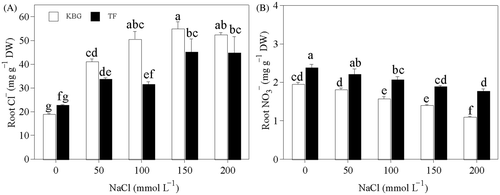
Shoot Na+/root Na+ ratio and Na+/K+ ratio of shoots and roots
In KBG, the shoot Na+/root Na+ ratio increased significantly from 50 to 200 mmol L−1 NaCl (). In TF, it increased from control to 100 mmol L−1 NaCl, and then decreased from 100 to 200 mmol L−1 NaCl (). The shoot Na+/root Na+ ratio of KBG was higher than that of TF except at 100 mmol L−1 NaCl. The Na+/K+ ratios in the shoots and roots of KBG increased significantly as salinity increased (). The shoot Na+/K+ ratio of TF did not change significantly above 100 mmol L−1 NaCl, but the root Na+/K+ ratio increased slightly (). Similar to Na+, the Na+/K+ ratios in the shoots and roots of KBG were significantly higher than those of TF at the same salinity.
Figure 5. Shoot Na+/root Na+ ratios of Kentucky bluegrass (KBG; Poa pratensis L.) and Tall fescue (TF; Festuca arundinacea Schreb.) at different sodium chloride (NaCl) concentrations. Bars indicate standard error (n = 3). Different lower case letters indicate significant differences between means at P = 0.05 according to Duncan's multiple range tests. Na+, sodium cations.
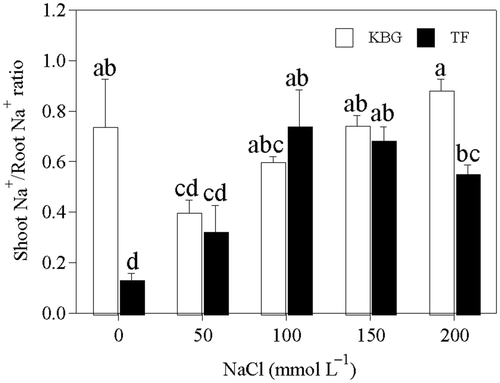
Figure 6. Na+/K+ ratio in the shoots and roots of Kentucky bluegrass (KBG; Poa pratensis L.) and Tall fescue (TF; Festuca arundinacea Schreb.) at different sodium chloride (NaCl) concentrations. Bars indicate standard error (n = 3). Different lower case letters indicate significant differences between means at P = 0.05 according to Duncan's multiple range tests. Na+, sodium cations; K+, potassium cations.
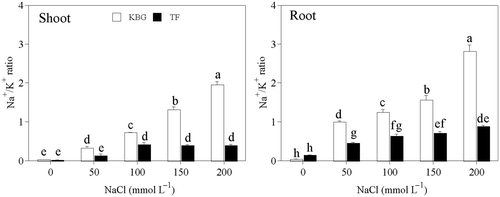
Proline and TSS concentrations of shoots and roots
The proline concentrations in shoots and roots of both species increased significantly as salinity increased (). That in the shoots was significantly higher in KBG than in TF at the same salinity, but that in the roots was significantly higher in TF than in KBG.
Figure 7. Proline concentrations in the shoots and roots of Kentucky bluegrass (KBG; Poa pratensis L.) and Tall fescue (TF; Festuca arundinacea Schreb.) at different sodium chloride (NaCl) concentrations. Bars indicate standard error (n = 3). Different lower case letters indicate significant differences between means at P = 0.05 according to Duncan's multiple range tests. FW, fresh weight.
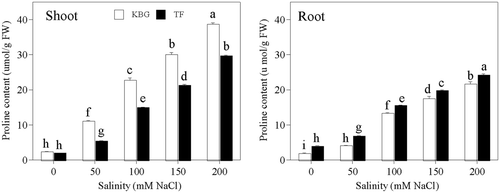
TSS in the shoots and roots of KBG decreased gradually as salinity increased (). However, TSS in the shoots of TF increased as salinity increased to 100 mmol L−1 NaCl, but then decreased at higher salinities. TSS in the roots of TF increased significantly as salinity increased. TSS of shoots was 42% to 97% higher in TF than in KBG, and that of roots was 26% to 311% higher in TF than in KBG, at 50 to 200 mmol L−1 NaCl ().
Figure 8. Total soluble sugar (TSS) concentrations in the shoots and roots of Kentucky bluegrass (KBG; Poa pratensis L.) and Tall fescue (TF; Festuca arundinacea Schreb.) at different sodium chloride (NaCl) concentrations. Bars indicate standard error (n = 3). Different lower case letters indicate significant differences between means at P = 0.05 according to Duncan's multiple range tests. DW, dry weight.
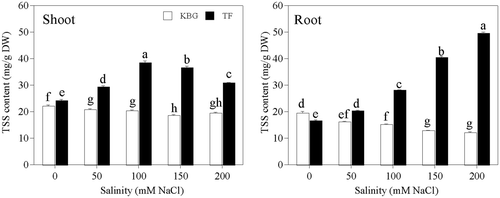
Estimated contributions of inorganic ions and compatible solutes to osmotic potential
There was no difference in Ψs between the two species in the control ( and ). The 50, 100, 150 and 200 mmol L−1 NaCl treatments decreased the Ψs of the nutrient solution from −0.06 to −0.24, −0.43, −0.69 and −0.84 MPa, respectively. This resulted in a decline in root and shoot Ψs in both KBG and TF. Ψs became more negative in shoots of KBG and TF as salinity increased, and decreased more significantly in TF than in KBG (). The estimated contribution of shoot Na+ to Ψs (C Na) in KBG increased significantly as salinity increased; that of TF remained unchanged from 100 to 200 mmol L−1 NaCl (). C Na of KBG was significantly higher than that of TF. The estimated contributions of shoot Cl− to Ψs (C Cl) in KBG changed significantly from 100 to 200 mmol L−1 NaCl; those in TF under salt stress were significantly higher than in the controls and did not represent a significant change, at 100 to 200 mmol L−1 NaCl (). The estimated contribution of shoot K+ to Ψs (C K) decreased more significantly in KBG than TF as the concentration of NaCl increased (). The estimated contribution of shoot TSS (C TSS) to Ψs in KBG increased at 150 and 200 mmol L−1 NaCl, but did not change significantly in TF (). However, C TSS was significantly higher in TF than in KBG at the same salinity. The estimated contribution of shoot proline to Ψs (C Pro) increased in both species as salinity increased (); it was lower than those of the other solutes.
Table 1. Effects of salinity on osmotic potential (Ψs) and the estimated contributions of sodium (Na+) (C Na), potassium (K+) (C K), chlorine (Cl−) (C Cl), total soluble sugar (TSS) (C TSS), and proline (C Pro) to Ψs in shoots of Kentucky bluegrass (KBG; Poa pratensis L.) and Tall fescue (TF; Festuca arundinacea Schreb.)
Table 2. Effects of salinity on osmotic potential (Ψs) and the estimated contributions of sodium (Na+) (C Na), potassium (K+) (C K), chlorine (Cl−) (C Cl), total soluble sugar (TSS) (C TSS), and proline (C Pro) to Ψs in roots of Kentucky bluegrass (KBG; Poa pratensis L.) and Tall fescue (TF; Festuca arundinacea Schreb.)
Similar to Ψs in shoots, Ψs in roots of KBG and TF became more negative as salinity increased, and the difference between KBG and TF was large at 200 mmol L−1 NaCl (). The estimated contribution of root Na+ to Ψs (C Na) in KBG and TF increased significantly as salinity increased (). C Na was higher in KBG than in TF. The estimated contribution of root Cl− to Ψs (C Cl) in KBG increased with the salinity treatment compared with the control. That in TF also increased at 50, 150 and 200 mmol L−1 NaCl (). However, there was no significant change in estimated contribution of root Cl− to Ψs (C Cl) between species under NaCl treatments. The estimated contribution of K+ to Ψs (C K) in roots was similar to that in shoots: C K decreased in KBG and TF as salinity increased, and more significant reduction was observed in KBG (). The estimated contribution of root TSS to Ψs (C TSS) in KBG increased as salinity increased (); that in TF did not change significantly, except at 200 mmol L−1 NaCl (). The estimated contribution of root TSS to C TSS of TF was significantly higher than that of KBG at the same salinity. The estimated contribution of root proline to Ψs (C Pro) increased in KBG and TF as salinity increased ().
Discussion
Ion toxicity and ion imbalance
In the present study, KBG exhibited greater growth reduction in shoots and roots with increasing salinity than did TF. The concentrations of Na+ and Cl− in shoots and roots of both species increased with increasing salinity, especially for KBG (, , , ). Salinity tolerance of TF was related to limiting the uptake of Na+ and Cl−. These results indicated that the different ionic concentrations between KBG and TF had an apparent relationship to salinity tolerance of both species.
Na+ and Cl− are the dominant toxic ions in saline soil. Higher Na+, often in conjunction with Cl−, results in specific ion toxicity and growth inhibition (Ashraf and Harris Citation2004; Zhu et al. Citation2008). High Na+ can replace Ca2+ and decrease the cell membrane integrity of the root for water and nutrient uptake. Accumulation of Na+ in the leaf leads to dehydration, reduced turgor and death of cells; accumulation of Cl− in the leaf can lead to leaf burn and desiccation in sensitive plants (Carrow et al. 2001a). In the present study, KBG accumulated higher Na+ and Cl− concentrations than TF in both shoots and roots. This result indicates the injurious effect of ion toxicity for KBG was greater than that for TF.
High salinity can affect essential cation uptake and nutrient balance. At the level of individual cells, one of the most damaging consequences of salt stress is an influx of Na+ and a decrease in K+ in plant tissues (Li et al. Citation2010). K+ is often considered as second in importance behind nitrogen (N) as a nutrient in turfgrass. K+ strongly influences turfgrass tolerance to drought, cold, high temperature, wear and salinity stresses (Carrow et al. 2001b). In comparison to the control, the concentrations of K+ in the shoots and roots of both species decreased with salt stress. Those in TF were significantly higher than in KBG at the same salinity (, ). K+ was the most important cation related to the shoot and root growth of many plants subjected to salt stress (Marschner Citation1995; Grattan and Grieve Citation1999; Lee et al. Citation2007). The higher K+ concentration in TF might be one of the reasons for the better growth under salt stress.
The Ca2+ and Mg2+ concentrations in the shoots of both species and Mg2+ in the roots of KBG decreased as salinity increased (, ), and the decreased extent of Ca2+ and Mg2+ concentrations in the roots were higher in KBG than that in TF. The decreases were probably due to interactive substitution with Na+ (El-Hendawy et al. Citation2005). Saline-induced Ca2+ deficiency may reduce certain salinity tolerances such as ion exclusion and selective transport. Ca2+ improves the tolerance of higher plants to salt stress, and its availability plays a major role in counteracting salt stress (Breckle Citation2002; Carrow et al. 2001a). However, we found no significant difference in Ca2+ concentration in shoots between KBG and TF under salinity stress. Mg2+ deficiency is relatively common in turfgrass grown in soils naturally when high Na+ is present. A high external Na+ concentration will easily displace Mg2+ and decrease Mg2+ uptake (Carrow et al. 2001a). However, Mg2+ in TF roots did not decrease under salt stress (). It should be further investigated whether Mg2+ accumulation is a special adaptation of TF under salt stress.
Under saline condition, Cl− competes with and depresses its uptake, which may cause the ion imbalance (Hu et al.
Citation2005). N is usually the most growth-limiting nutrient for plants. Thus, the great decrease in the
concentration of KBG may reduce its growth significantly.
in the shoots and roots of TF was higher than that in KBG, indicating that effects of salinity on uptake vary considerably with species. TF maintains more healthy growth and stress tolerance by active
accumulation under salt stress.
High salinity can affect essential ion uptake and nutrient balance. Compared to TF, KBG accumulated higher Na+ and Cl− concentrations in shoots and roots, which reduced K+, Ca2+, Mg2+, and concentrations greatly. The Na+ and Cl− toxicity and nutrient imbalance was more significant in KBG than in TF under salt stress. The difference concentrations of K+ and
between KBG and TF appeared to be related to salinity tolerance. These results would be important information for managing this species in salt-affected environments. When turfgrasses are grown on salt-affected soils or irrigated with recycled wastewater, increasing K and N fertilizer properly may improve plant growth.
Ion regulation and Na/K ratio
Salinity tolerance is associated with the capacity to limit uptake and transport saline ions from the root zone to the aerial parts (Greenway and Munns Citation1980). In both species, Na+ concentrations were lower in shoots than in roots ( and ). Thus, the roots play an important role in limiting the transport of Na+ to the shoots of both species. However, TF had a lower Na+ concentration in the roots and a lower shoot Na+/root Na+ ratio compared with KBG ( and ). This result indicates a strong ability of TF to limit Na+ uptake by roots and to prevent Na+ transport from roots to shoots.
With respect to K+ concentration significant species differences were observed with apparent relationship to salinity tolerance ( and ). Comparisons between KBG and TF exhibited some difference in Na+/K+ ratios in the shoots and roots with increasing salinity. The Na+/K+ ratios in the shoots and roots of KBG increased significantly as salinity increased. However, under salt stress those of TF were significantly lower than those of KBG (). The Na+/K+ ratio of TF was higher in the roots than in the shoots, and did not reach 1 in either organ. The capacity of plants to maintain a low cytosolic Na+/K+ ratio is likely to be one of the key determinants of salt tolerance (Yeo Citation1998; Maathuis and Amtmann Citation1999). This result indicates that TF had a better selectivity for K+ over Na+ than KBG via roots and transporting K+ from roots to shoots under salt stress.
Osmotic adjustment
Osmotic adjustment is one of the main strategies by which plants ensure water uptake during salinity or drought stress conditions (Greenway and Munns Citation1980). In TF, shoot and root osmotic potential changed significantly as salinity increased ( and ). TF was able to maintain a stable gradient of Ψs between shoots, roots and saline nutrient solution, suggesting that osmotic adjustment is an important mechanism in relation to TF's better salinity tolerance. Na+, Cl−, and K+ were the principal inorganic ions for osmotic adjustment in both species under salt stress ( and ). However, their contributions to the total measured osmotic potential differed with salinity treatment and grass species. Under salt stress, Na+ and Cl− made a larger contribution to osmotic potential in KBG than in TF. The availability of Na+ and Cl− as cheap osmoregulator is generally beneficial. However, excessive accumulation of Na+ and Cl− results in ion toxicity and growth inhibition. The estimated contribution of K+ to Ψs (C K) in KBG decreased significantly as salinity increased. However, TF had a higher, and stable, C K from 100 to 200 mmol L−1 NaCl. K+ has been reported to be involved in activation of several enzymes, membrane transport, maintenance of cystolic osmotic potential, and maintenance of osmotic potential in vacuoles (Marschner Citation1995). These results suggest that TF has a strong mechanism for active uptake of K+ as an osmoregulator for osmotic adjustment.
Osmotic potential of the cytoplasm is maintained by the accumulation of organic solutes to osmotically balance Na+ and Cl− within the vacuole. In this study, TSS played an important role in balancing osmotic potential under salt stress. C TSS in KBG decreased as salinity increased, but that in TF was significantly higher at the same salinity ( and ). These results suggest that TF, but not KBG, can efficiently mitigate NaCl stress by accumulating TSS for osmotic adjustment. Sugar accumulation is strongly correlated with salt tolerance (Streeter et al. Citation2001; Taji et al. Citation2002). More TSS was accumulated in TF than in KBG at the same salinity (), indicating the importance of TSS in salinity tolerance of TF.
The accumulation of proline in response to abiotic stresses contributes to osmotic adjustment in the cytoplasm (Ashraf and Foolad Citation2007). The proline concentrations in shoots and roots of both species increased significantly as salinity increased (). However, it contributed less than 7% of the total osmotic potential ( and ). Thus, neither species could accumulate enough proline to mitigate NaCl stress by osmotic adjustment. The accumulation of proline by many plant species under salt stress has been correlated with salinity tolerance, and the concentration of proline is generally higher in stress-tolerant than in stress-sensitive plants (Ashraf and Foolad Citation2007). However, this correlation is controversial: For example, under salt stress, proline levels of the salt-sensitive KBG were similar to those of salt-tolerant alkalinegrass (Puccinellia distans L. Parl.) (Torello and Rice Citation1986); sensitive rice (Oryza sativa L.) cultivars accumulated greater amount of proline than did the tolerant genotypes (Lutts et al. Citation1999). The accumulation of proline in rice and KBG coincided with a sharp increase in leaf burn (Lutts et al. Citation1999; Qian et al. Citation2001), indicating a symptom of salt injury rather than salt tolerance. In this study, the accumulated proline was very low and the accumulation pattern was not related to salinity tolerance, suggesting that proline accumulation was a reaction to salt stress and not a salinity tolerance mechanism in KBG and TF.
The percentages of individual estimated contributions to osmotic potential under salt stress show that KBG achieved osmotic adjustment more by inorganic ions than through organic osmolytes. The high reliance on inorganic ions for osmotic adjustment is vital for water uptake under a saline environment. However, osmotic adjustment of TF was achieved by both inorganic ion and organic osmolytes based on percentage contribution to Ψs, respectively. TF maintained higher concentrations of K+ and TSS in the cytoplasm to achieve cytoplasmic osmotic adjustment, which may explain its greater salinity tolerance compared to KBG.
In summary, our results indicate that the different physiological responses of KBG and TF to salt stress were highly related to ionic distribution and the accumulation of compatible osmolytes. TF had a high salt tolerance due to its restricted uptake of Na+ and Cl− in conjunction with maintenance of higher K+ in shoots and roots and the accumulation of enough total soluble sugars to make osmotic adjustment.
Acknowledgments
This study was supported by a grant from the Global Center of Excellence for Dryland Science, a project of the Ministry of Education, Science, Culture, Sports and Technology of Japan.
References
- Alshammary , SF , Qian , YL and Wallner , SJ . 2004 . Growth response of four turfgrass species to salinity . Agric. Water Manage. , 66 : 97 – 111 .
- Angelov , MN , Sun , J , Byrd , GT , Brown , RH and Black , CC . 1993 . Novel characteristics of cassava, Manihot esculenta Crantz, a reputed C3-C4 intermediate photosynthetic species . Photosynth. Res. , 38 : 61 – 72 .
- Ashraf , M and Foolad , MR . 2007 . Roles of glycine betaine and proline in improving plant abiotic stress resistance . Environ. Exp. Bot. , 59 : 206 – 216 .
- Ashraf , M and Harris , PJC . 2004 . Potential biochemical indicators of salinity tolerance in plants . Plant Sci. , 166 : 3 – 16 .
- Bates , LS , Waldren , RP and Teare , ID . 1972 . Rapid determination of free proline for water stress studies . Plant Soil , 39 : 205 – 207 .
- Bohnert , HJ and Shen , B . 1999 . Transformation and compatible solute . Sci. Hortic. , 78 : 237 – 260 .
- Breckle , SW . 2002 . “ Salinity, halophytes and salt affected natural ecosystems ” . In Salinity: Environment-Plants-Molecules , Edited by: Läuchli , A and Lüttge , U . 53 – 77 . Dordrecht : Kluwer Academic Publishers .
- Carrow , RN and Duncan , RR . 1998 . Salt-Affected Turfgrass Sites: Assessment and Management , Chelsea , MI : Ann Arbor Press .
- Carrow , RN , Waddington , DV and Rieke , PE . (Eds.) 2001a: Basics of salt-affected soils. In Turfgrass Soil Fertility and Chemical Problems: Assessment and Management. pp. 104–106. John Wiley and Sons, Hoboken, NJ
- Carrow , RN , Waddington , DV and Rieke , PE . (Eds.) 2001b: Potassium. In Turfgrass Soil Fertility and Chemical Problems: Assessment and Management. pp. 211–214. John Wiley and Sons, Hoboken, NJ
- Chen , JB , Yan , J , Qian , YL , Jiang , YQ , Zhang , TT , Guo , HL , Guo , AG and Liu , JX . 2009 . Growth responses and ion regulation of four warm season turfgrasses to long-term salinity stress . Sci. Hortic. , 122 : 620 – 625 .
- Demiral , T and Türkan , İ . 2005 . Comparative lipid peroxidation, antioxidant defense systems and protein in roots of two rice cultivars differing in salt tolerance . Environ. Exp. Bot. , 53 : 247 – 257 .
- El-Hendawy , SE , Hu , Y and Schmidhalter , U . 2005 . Growth, ion content, gas exchange, and water relations of wheat genotypes differing in salt tolerances . Aust. J. Agric. Res. , 56 : 123 – 134 .
- Flowers , TJ and Colmer , TD . 2008 . Salinity tolerance of halophytes . New Phytol. , 179 : 945 – 963 .
- Flowers , TJ , Troke , PF and Yeo , AR . 1977 . The mechanism of salt tolerance in halophytes . Ann. Rev. Plant Physiol. , 28 : 89 – 121 .
- Grattan , SR and Grieve , CM . 1999 . Salinity-mineral nutrient relations in horticultural crops . Sci. Hort. , 78 : 127 – 157 .
- Greenway , H and Munns , R . 1980 . Mechanism of salt tolerance in non-halophytes . Annu. Rev. Plant Physiol. , 31 : 149 – 190 .
- Harivandi , A . 1988 . Irrigation water quality: One key to success in golf course management . Golf Course Manage. , 106–107 : 189 – 193 .
- Horst , GL and Beadle , NB . 1984 . Salinity affects germination and growth of tall fescue cultivars . J. Am. Soc. Hort. Sci. , 109 : 419 – 422 .
- Hu , Y , Fricke , W and Schmidhalter , U . 2005 . Salinity and the growth of non-halophytic grass leaves: The role of mineral nutrient distribution . Funct. Plant Biol. , 32 : 973 – 985 .
- Kobayashi , H , Sato , S and Masaoka , Y . 2004 . Tolerance of grasses to calcium chloride, magnesium chloride and sodium chloride . Plant Prod. Sci. , 7 : 30 – 35 .
- Lee , GJ , Duncan , RR and Carrow , RN . 2007 . Nutrient uptake responses and inorganic ion contribution to solute potential under salinity stress in halophytic seashore paspalums . Crop Sci. , 47 : 2410 – 2419 .
- Lerner , HR , Amzallag , GN , Friedman , Y and Golobinoff , P . 1994 . The response of plants to salinity: From turgor adjustments to genome modification . Isr. J. Plant Sci. , 42 : 285 – 300 .
- Li , R , Shi , F , Fukuda , K and Yang , Y . 2010 . Effects of salt and alkali stresses on germination, growth, photosynthesis and ion accumulation in alfalfa (Medicago sativa L.) . Soil Sci. Plant Nutr. , 56 : 725 – 733 .
- Lutts , S , Majerus V and Kinet , JM . 1999 . NaCl effects on proline metabolism in rice (Oryza sativa) seedlings . Physiol. Plant. , 105 : 450 – 458 .
- Maathuis , FJM and Amtmann , A . 1999 . K+ nutrition and Na+ toxicity: The basis of cellular K+/Na+ ratios . Ann. Bot. , 84 : 123 – 133 .
- Marcum , KB . 2006 . Use of saline and non-potable water in turfgrass industry: Constraints and developments . Agric. Water Manage. , 80 : 132 – 146 .
- Marcum , KB . 2008 . “ Physiological adaptations of turfgrass to salinity stress ” . In Handbook of Turfgrass Management and Physiology , Edited by: M , Pessarakli . 407 – 418 . Boca Raton , FL : CRC Press .
- Marcum , KB and Murdoch , CL . 1994 . Salinity tolerance mechanisms of six C4 turfgrasses . J. Am. Soc. Hort. Sci. , 119 : 779 – 784 .
- Marschner , H . 1995 . Mineral Nutrition of Higher Plants , San Diego : Academic Press .
- McCarty , LB and Dudeck , AE . 1993 . Salinity effects on bentgrass germination . HortScience , 28 : 15 – 17 .
- McCready , RM , Guggolz , J , Silviera , V and Owens , HS . 1950 . Determination of starch and amylase in vegetables . Anal. Chem. , 22 : 1156 – 1158 .
- Nobel , PS . 1991 . Physicochemical and Environmental Plant Physiology , New York : Academic Press .
- Orcutt , DM and Nilsen , ET . 2000 . Physiology of Plants Under Stress: Soil and Biotic Factors , New York , NY : John Wiley and Sons .
- Qian , YL and Harivandi , A . 2008 . “ Salinity issues associated with recycled wastewater irrigation of turfgrass landscapes ” . In Handbook of Turfgrass Management and Physiology , Edited by: M , Pessarakli . 419 – 429 . New York , NY : CRC Press .
- Qian , YL , Wilhelm SJ and Marcum , KB . 2001 . Comparative responses of two Kentucky bluegrass cultivars to salinity stress . Crop Sci. , 41 : 1895 – 1900 .
- Song , J , Ding , X , Feng , G and Zhang , F . 2006 . Nutritional and osmotic roles of nitrate in a euhalophyte and a xerophyte in saline conditions . New Phytol. , 171 : 357 – 366 .
- Streeter , JG , Lohnes , DG and Fioritto , RJ . 2001 . Patterns of pinitol accumulation in soybean plants and relationships to drought tolerance . Plant Cell Environ. , 24 : 429 – 438 .
- Suplick-Ploense , MR , Qian , YL and Read , JC . 2002 . Relative NaCl tolerance of Kentucky bluegrass, Texas bluegrass, and their hybrids . Crop Sci. , 42 : 2025 – 2030 .
- Taji , T , Ohsumi , C , Iuchi , S , Seki , M , Kasuga , M , Kobayashi , M , Yamaguchi-Shinozaki , K and Shinozaki , K . 2002 . Important roles of drought- and cold-inducible genes for galactinol synthase in stress tolerance in Arabidopsis thaliana . Plant J. , 29 : 417 – 426 .
- Torello , WA and Rice , LA . 1986 . Effects of NaCl stress on proline and cation accumulation in salt sensitive and tolerant turfgrasses . Plant Soil , 93 : 241 – 247 .
- Torello , WA and Symington , AG . 1984 . Screening of turfgrass species and cultivars for NaCl tolerance . Plant Soil , 82 : 155 – 161 .
- Turgeon , AJ . 2008 . “ Turfgrass species ” . In Turfgrass Management , 8th , Edited by: J , Jones-Renger . 63 – 123 . Upper Saddle River , NJ : Pearson Prentice Hall .
- Wipff , JK and Rose-Fricker , CA . 2003: Salt tolerance comparisons among tall fescue cultivars. In Annual Meetings Abstracts [CD-ROM]. ASA, CSSA and SSSA, Madison, WI
- Xu , R , Yamada , M and Fujiyama , H . 2013: Lipid peroxidation and antioxidant enzymes of two turfgrass species under salinity stress. Pedosphere, 23, 213–222
- Yamanouchi , M , Tanaka , S and Fujiyama , H . 1997 . The cultivarietal differences in salt-tolerance and effect of NaCl on the absorption and translocation of K, Ca and Mg ions in Phaseolus vulgaris L . J. Jpn. Soc. Hort. Sci. , 65 : 737 – 745 .
- Yeo , AR . 1998 . Molecular biology of salt tolerance in the context of whole-plant physiology . J. Exp. Bot. , 49 : 915 – 929 .
- Zhu , J . 2001 . Plant salt tolerance . Trends Plant Sci. , 6 : 66 – 71 .
- Zhu , J . 2002 . Salt and drought stress signal transduction in plants . Ann. Rev. Plant Biol. , 53 : 247 – 273 .
- Zhu , J . 2003 . Regulation of ion homeostasis under salt stress . Curr. Opin. Plant Biol. , 6 : 441 – 445 .
- Zhu , J , Bie , ZL , Huang , Y and Han , X . 2008 . Effect of grafting on the growth and ion concentrations of cucumber seedlings under NaCl stress . Soil Sci. Plant Nutr. , 54 : 895 – 902 .