Abstract
Soil erosion has resulted in serious land degradation in Yuanmou dry-hot valley, Yunnan province, China. Vegetation restoration had been widely developed in this hot and arid area. In this work we investigate the quality of rhizosphere soils under four restoration species in the towns of Zuolin (gully bed) and Yuanma (slope area). The vegetation included two trees [Leucaena leucocephala (Lam.) de Wit. and Eucalyptus camaldulensis L.] for the slope area, one shrubland [Dodonaea viscosa (Linn.) Jacq. Enum.] and one native, natural grassland [Heteropogon contortus (L.) Beauv.] for gully bed. Soil physical (bulk density and soil moisture content), chemical [pH, soil organic matter, nitrogen (N), phosphorus (P) and potassium (K) contents], and microbial (microbial biomass and microbe quantity) properties and soil enzyme (catalase, protease and urease) activities were determined. Compared to the soils collected from other vegetation types, the soil under Leucaena leucocephala had the highest content of soil organic matter, total N, available N, total P, available P, available K, microbial biomass carbon (C), and the highest microbe quantity and enzyme activities. Five parameters (organic matter, pH, microbial biomass N, total K and protease activity), which had the greatest weight in the principal components analysis, were selected to calculate the soil quality index (SQI). The final SQI values implied that restoring Leucaena leucocephala greatly improved the soil quality, while restoration with Eucalyptus camaldulensis decreased the soil quality of the slope area. Plant recovery with Dodonaea viscosa and Heteropogon contortus on the gully bed affected the population of soil microbial communities but didn't improve the SQI values. It can therefore be seen that types of plants used for recovery in gully-developed regions in dry-hot valleys must be chosen cautiously: Leucaena leucocephala is a good choice for soil revegetation of the slope area, but the revegetation patterns on the gully bed still need further study.
1. INTRODUCTION
Soil degradation caused by erosion is a serious problem in many parts of the world. It not only destroys the function of the ecological environment, but also threatens economic and cultural development at regional and global scales (Lal Citation2001). Yuanmou (E 101°35′∼102°06′, N 25°23′∼26°06′) is located in a dry-hot valley of the Jinsha River, which is the upper reach of the Yangtse River, the longest river in China. Yuanmou dry-hot valley is among the regions that are suffering from soil degradation mainly caused by erosion. Gully bed, caused by gully erosion, can be seen everywhere. The vegetation of Yuanmou is termed valley-type savanna vegetation, and is predominantly composed of grasses and bushes, with a few trees (Jin and Ou Citation2000). In the last 50 years, the Chinese government has made great efforts in vegetation restoration in this area. Despite the recognition of the effects of these vegetation restorations on soil water reserves, nutrient status and soil enzymatic activities (Chen et al. Citation2003; K.Q. Wang et al. Citation2004; Y.C. Zhang Citation2005; Li et al. Citation2006; Nie et al. Citation2008; Y.L. Wei et al. Citation2011), the degrees of soil quality amelioration under different vegetation types were not clear. In order to inform vegetation restoration practice objectively, there is an urgent need to assess comprehensively the effects of different vegetation types on soil quality in Yuanmou dry-hot valley.
Soil quality relates to a large number of soil properties, including physical, chemical and microbial properties. Soil physical and chemical properties, such as soil organic matter, which is a nutrient source in the soil (Gregorich et al. Citation1994), generally change slowly and are not able to quickly and thoroughly reflect the variations resulting from disturbance. In contrast, soil microbial indices are sensitive to small changes in the environment, and there is growing evidence that microbial indices can be used as indicators of soil quality. For instance, microbial communities are involved in many important soil functions such as the carbon (C) and nitrogen (N) cycle, soil structure and plant fertility (Rillig and Mummey Citation2006; French et al. Citation2009; Ollivier et al. Citation2011; Chaparro et al. Citation2012). Soil microbial biomass is a labile and active component of soil organic matter and plays a key role in soil nutrient cycles and energy flow (Jenkinson and Ladd Citation1981). Soil enzymes participate in a wide range of metabolic processes (Burns Citation1982), including, for example, the mineralization of organic matter, the metabolism of N and the release of nutrients. Consequently, soil microbial indices have been considered to be an important indicator of soil quality because they integrate physical and chemical soil properties.
How is it possible to assess the quality of soil effectively and simply with these numerous indices? The development of a soil quality index (SQI) based on a combination of different soil properties has been shown to solve this problem. The establishment of an SQI involves three main steps: (1) selecting appropriate parameters for a minimum data set, (2) transforming the indicators to scores and (3) combining the scores into an index (Andrews et al. Citation2002a). This framework, which is based on mathematical theory, was later applied by different experts. Andrews et al. (Citation2002b) evaluated the effects of different soil management practices on soil quality under different crops. A soil microbiological degradation index (MDI) based on five parameters (dehydrogenase activity, water-soluble carbohydrates, urease activity, water-soluble C and respiration) was proposed in a semiarid climate (Bastida et al. Citation2006). Sinha et al. (Citation2009) determined that tree species with a higher rhizosphere soil microbial index (RSMI) could be used for revegetation in a degraded coal mining ecosystem. Recently, C. Zhang et al. (Citation2011a and Citation2011b) introduced this approach into their evaluation of the impact of revegetation types on soil quality on the Loess Plateau, China. However, little knowledge is available concerning soil quality assessment by a comprehensive index in the Yuanmou dry-hot valley.
The current study investigated the quality of rhizosphere soils under four revegetation species in the towns of Zuolin (gully bed) and Yuanma (slope area) in the Yuanmou dry-hot valley. The vegetation included two trees [Leucaena leucocephala (Lam.) de Wit. and Eucalyptus camaldulensis L.] for the slope area, one shrubland [Dodonaea viscosa (Linn.) Jacq. Enum.] and one native, natural grassland [Heteropogon contortus (L.) Beauv.] for gully bed. The objectives of this study were: (1) to assess changes in soil properties, including physical, chemical and microbial parameters, after four areas had been revegetated; (2) to determine a minimum set of soil properties for an SQI which is capable of assessing soil quality in Yuanmou dry-hot valley and (3) to select suitable plant species for land degradation recuperation in gully-developed regions.
2. MATERIALS AND METHODS
2.1. Study site
The study sites are located in Yuanmou dry-hot valley, Yunnan province, China (). Yuanmou dry-hot valley (E 101°35′∼102°06′, N 25°23′∼26°06′) is in the lower reaches of the Jinsha River, which is the upper reach of the Yangtze River. This area has an arid climate, with a mean annual temperature of 21.5°C and an average annual precipitation of 600–800 mm, mostly falling from May to September. The potential evaporation is 3400–3900 mm, which is approximately six times as great as the precipitation. The soil is mainly dry red soil [Chromic cambisol in Food and Agriculture Organization (FAO) taxonomy, ustochrept in United States Department of Agriculture (USDA) taxonomy], which is vulnerable to water erosion. Because of the special natural and geographical conditions, gully erosion and broken topography in the area are both particularly severe.
2.2. Experiment design and soil sampling
The sampling areas were located in the towns of Zuolin and Yuanma in Yuanmou county, at the Research Station for Gully Erosion and Collapse (jointly established by the Institute of Mountain Hazards and Environment, Chinese Academy of Sciences, and the Ministry of Water Resources and the Yunnan Research Institute for Tropical Eco-agricultural Sciences, Yunnan Academy of Agricultural Sciences, called Zuolin and Yuanma stations, respectively) (). Each experimental site covered an area of approximately 2 ha. At Yuanma station, three vegetation types were established at three adjacent sloping fields about 10 years ago: Leucaena leucocephala, Eucalyptus camaldulensis and bare soils (no coverage, which were subject to erosion, CK1). The trees were planted on a 4 m × 4 m grid. At Zuolin station, three vegetation patterns on the gully bed were included in this site: one shrubland (Dodonaea viscosa, planted on a 0.5 m × 0.5 m grid 10 years ago), one natural, native grassland (Heteropogon contortus) and one bare soil (no coverage, which was subject to erosion, CK2). Three fields at each station were all similar in terms of slope, altitude, soil type and previous land use pattern, so we can assume that the differences in soil properties could be attributed to the type of vegetation. All the plants have been growing without irrigation, fertilization and disturbance after planting. Detailed information on the six vegetation types is shown in .
Table 1 Sample site description
Samplings were conducted in March 2012. Three subplots were established randomly under each vegetation type: 1 m × 1 m for Heteropogon contortus, CK1 and CK2; 2.5 m × 2.5 m for Dodonaea viscosa and 20 m × 20 m for Leucaena leucocephala and Eucalyptus camaldulensis. After litter was removed, three rhizosphere soil samples from the top 20 cm of the soil profile were collected randomly in each subplot of Heteropogon contortus, Dodonaea viscosa, CK1 and CK2, and then the three samples were thoroughly mixed. In each subplot of trees (Leucaena leucocephala and Eucalyptus camaldulensis), five rhizosphere soil samples were collected along an “S” pattern line, and then the five samples were thoroughly mixed, too. The distance between the sampling sites and the tree trunks was about 0.5 m. The soil that was strongly adhering to roots or found within the space explored by roots was considered to be the rhizosphere soil (Garcia et al. Citation2005). Each sample was divided into two parts. One part was immediately sieved through a 2-mm mesh and then stored at 4°C until soil microbial properties could be analysed; the other section was air-dried for analysis of physicochemical soil properties.
2.3. Analytical procedures
The physicochemical properties of soils were analysed according to the standard methods recommended by the Chinese Society of Soil Science (Lu Citation2000). Bulk density (BD) was determined from the soil cores with a fixed volume of 100 cm3 at each sampling point. Natural soil moisture (SM) content was determined by oven-drying the samples at 105°C over night, and the water content was expressed as a percentage of the dry weight. Soil pH was determined with a pH meter (PHS-3C, Leizi, China) in 1:2.5 (soil: water, weight/volume, air-dried soil) suspensions. Soil organic matter (SOM) and total organic C were detected by the potassium dichromate-volumetric method [digested by K2Cr2O7-sulfuric acid (H2SO4)], and total nitrogen (TN) by the Kjeldahl method [digested by sulfuric acid (H2SO4) and cupric sulfate-sodium sulfate (CuSO4-Na2SO4) as the catalyst agent]. Soil total phosphorus (TP) and total potassium (TK) were determined by the molybdenum (Mo)-antimony (Sb) colorimetric method and flame atomic absorption spectrophotometry, respectively, after wet digestion with sodium hydroxide (NaOH). Soil available nitrogen (AN) was determined using a micro-diffusion technique after alkaline hydrolysis. Soil available phosphorus (AP) was determined by the Olsen method. Soil available potassium (AK) was measured in 1 M ammonium acetate (NH4OAc) extracts by flame atomic absorption spectrophotometry. Dissolved organic carbon (DOC) was extracted by 0.5 M potassium sulfate (K2SO4) and measured using volumetric analysis (Bolan et al. Citation1996).
Microbial biomass carbon (MBC) and microbial biomass nitrogen (MBN) were measured using the fumigation extraction method (Vance et al. Citation1987; Wu et al. Citation1990; Wu et al. Citation2006). Oven-dry equivalent field-moist soil (12.5 g) was fumigated for 24 h at 25°C with ethanol-free chloroform (CHCl3). After fumigant removal, the soil was treated with 50 mL of 0.5 M K2SO4 by horizontal shaking for 30 min at 200 rpm and was then filtered. Soil organic carbon and TN in the extraction were measured by volumetric analysis and the Kjeldahl method, respectively. MBC and MBN were calculated using conversion factors of 0.38 (kEC) and 0.54 (kEN) (Vance et al. Citation1987).
Soil enzyme activities were measured according to the methods described by Lin (Citation2010). Catalase activity (CA) was assayed using 5 g fresh soil with 40 mL distilled water and 5 mL 0.3% hydrogen peroxide (H2O2), shaken for 30 min at 120 rpm, followed by the addition of 5 mL 1.5 M H2SO4 to end the reaction, and the mixture was then filtered. The filtrate was titrated with 0.01 M potassium permanganate (KMnO4) and the results were expressed as ml 0.01M KMnO4 g−1 soil. Protease activity (PA) was extracted using 5 g fresh soil with 1 mL methylbenzene and 25 mL Tyrosine matrix solution at 30°C for 48 h, and 25 mL protein precipitant was then added to facilitate precipitation for 30 min, and the solution was then filtered. The filtrate was assayed by Folin-colorimetry and the results were expressed as a μg glycine g−1 soil 24 h enzymatic reaction. Urease activity (UA) was determined using 10 g fresh soil, 20 mL of citrate solution (pH = 6.7) and 10 mL of 10% urea solution. The samples were incubated at 38°C for 3 h, and then diluted to 100 mL with distilled water (heated to 38°C). The suspension was filtered, and 1 mL filtrate was then treated with 4 mL of sodium phenol solution and 3 mL of 0.9% sodium hypochlorite solution. The released ammonium was quantified by colorimetry at 578 nm. The results were expressed as mg ammonium-nitrogen (NH4 +-N) g−1 soil h−1.
The populations of three microbial communities, including bacteria (the population of bacteria community, BCP), fungi (the population of fungi community, FCP) and actinomycetes (the population of actinomycetes community, ACP) were detected using the traditional dilution plate method (Dong Citation1997). The microbes were cultivated in different media. Viable total counts of bacteria, fungi and actinomycetes were determined as colony-forming units, on a beef extract peptone medium, Martin's medium and Gause's No. 1 synthetic medium, respectively. They were cultivated at 28°C in a constant-temperature incubator. The media growth results were read on days 2, 3 and 7 and the results were expressed as cfu g−1 dry soil (DS).
2.4. Soil quality index (SQI)
According to the method described by Bastida et al. (Citation2006) and Masto et al. (Citation2008), a soil quality index (SQI) was established. A principal component analysis (PCA) was used to select the appropriate indices and their weighting factors. Only the variables with high factor loadings were retained for each principal component (PC), and high factor loadings were defined as having absolute values within 10% of the highest factor loading (Andrews et al. Citation2002a). When more than one variable was retained under a single PC, multivariate correlation was employed to determine if the variables could be considered redundant and, therefore, eliminated from the SQI. If the high factor variables were not correlated with each other, then each was considered important and thus retained. Among well-correlated parameters, the parameter with the highest factor loading was chosen for the SQI (Bastida et al. Citation2006; Masto et al. Citation2008; Sinha et al. Citation2009; C. Zhang et al. Citation2011b). Each PC represented a certain amount of variation (%) in the total dataset and this percentage provided the weighting for the parameters under a given PC. The final SQI equation based on the PCA was as follows:
2.5. Data analysis
Differences between mean values were evaluated by a one-way analysis of variance (ANOVA). Correlation analyses, scoring and PCA were performed using Microsoft Excel and SPSS 13.0 for Windows packages.
3. RESULTS
3.1. Soil physicochemical properties
The average values of physicochemical parameters of soil are presented in . SOM, TN, AN and AP in the soils collected from the sites in the town of Yuanma were higher than these from the sites in the town of Zuolin. The highest contents of SOM, TN, AN, AP, AK were found in the rhizosphere soils of Leucaena leucocephala, but the highest value of TK (13.6 g kg−1) was found in the soil from the gully bed with no vegetation (CK2). At Yuanma town site vegetation restoration with Leucaena leucocephala dramatically increased SOM, pH (6.85), TN, AN, TP, AP and AK when compared to the control site with no vegetations (CK1). However, vegetation restoration using Eucalyptus camaldulensis significantly decreased the values of soil pH (5.41), TN and TP, and there was also a declining trend for AP, TK and AK. At the Zuolin town site, the rhizosphere soil of Dodonaea viscosa had the highest SOM, TN and AP contents, but the lowest value for soil pH and TP (0.15 g kg−1). Soil moisture content in the natural field was very low, ranging from 4.1% under Eucalyptus camaldulensis to 0.8% in the gully bed with no vegetation (CK2). Bulk densities under tree species were significantly lower than for all the other vegetation covers.
Table 2 Physicochemical soil properties of different vegetation types
3.2. Soil microbial biomass and microbe quantity
The populations of BCP, FCP and ACP, and the TCP varied intensively among the different vegetation types (). BCP, ACP and TCP were greatest in the rhizosphere soils of Leucaena leucocephala, while the highest FCP was found under Heteropogon contortus. At the Yuanma town site, vegetation restoration using Eucalyptus camaldulensis decreased BCP, FCP and TCP. At the Zuolin town site, although both BCP and ACP under Dodonaea viscosa were found in greater quantity than those in the rhizosphere soils of Heteropogon contortus, TCP in the rhizosphere soils of Heteropogon contortus had a higher value. In general, BCP was larger than FCP and ACP under each vegetation; vegetation restoration using Leucaena leucocephala and Heteropogon contortus significantly increased FCP and TCP ().
Table 3 The populations of microbial communities, including bacteria (BCP), fungi (FCP), actinomycetes (ACP) and total populations (TCP) of different vegetation types
The microbial biomass contents (MBC and MBN) and DOC differed significantly between vegetation types (). MBC values varied from 524.4 mg kg−1 under Leucaena leucocephala to 69.7 mg kg−1 under Heteropogon contortus, while DOC ranged from 44.24 mg kg−1 under Eucalyptus camaldulensis to 15.3 mg kg−1 in the soil of the gully bed (CK2), which is experiencing heavy erosion. The greatest content of MBN (10.4 mg kg−1) was found under Leucaena leucocephala, which was approximately 10 times higher than that under Heteropogon contortus (1.0 mg kg−1). At the Yuanma town site, revegetation by Eucalyptus camaldulensis significantly increased DOC and MBN relative to bare soil (CK1), but significantly decreased MBC. Revegetation using Leucaena leucocephala showed a trend of augmenting the DOC, MBC and MBN content. The soil under Dodonaea viscosa at the Zuolin town site, showed the highest DOC, MBC and MBN contents.
Figure 2 Dissolved organic carbon (DOC), microbial biomass carbon (MBC) and microbial biomass nitrogen (MBN) under different vegetation types. Values with the same letter in a group are not significantly different at the p < 0.05 level. CK1, bare soil (no coverage) in Yuanma station (slope area); CK2, bare soil (no coverage) in Zuolin station (gully bed).
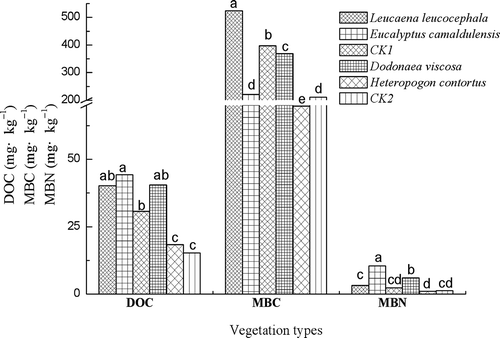
3.3. Soil enzyme activities
shows the enzyme activity (catalase, protease and urease) in the rhizosphere soils under different vegetation types. Catalase, protease and urease activities were highest under Leucaena leucocephala, with values of 26.4 mL 0.01 mol L−1 KMnO4 g−1, 80.6 μg glycine g−1 24h−1 and 20.0 mg NH4 +-Ng−1h−1, respectively. Catalase activity was significantly divided into four groups. The first group for Leucaena leucocephala had the highest value. The second group contained Eucalyptus camaldulensis and Dodonaea viscosa. The third group was the bare soil from Yuanma town (CK1). The last group had the lowest values of 9.1–9.3 mL 0.01M KMnO4 g−1. At the Yuanma town site, vegetation restoration with Eucalyptus camaldulensis significantly improved catalase and protease activity, whereas urease activity significantly decreased. At the Zuolin town site, vegetation restoration using Dodonaea viscosa increased catalase and urease but reduced protease activity.
Figure 3 Soil enzymes activities (catalase, protease and urease) under different vegetation types. Values with the same letter in a group are not significantly different at the p < 0.05 level. CK1, bare soil (no coverage) in Yuanma station (slope area); CK2, bare soil (no coverage) in Zuolin station (gully bed).
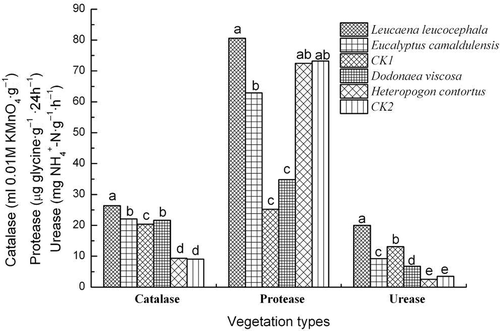
3.4. Soil quality index (SQI)
All of the soil parameters that were significantly different were selected through PCA for the SQI. The eigenvalues of the first four PCs were above 1 (). The highly weighted factors in PC-1 were organic matter (OM), TN, catalase activity (CA) and urease activity (UA). OM had the greatest loading weight and was highly correlated with TN, CA and UA (correlation coefficient r > 0.90; ), which were therefore eliminated from the SQI and only OM was retained. In PC-2, soil pH and MBN were highly loaded. The correlation between pH and MBN was less (r < 0.90; ), and both pH and MBN were therefore included in the SQI. Similarly, TK and PA from PC-3 and PC-4 were included in the SQI. The final parameters chosen from the PCA were OM, pH, MBN, TK and PA, and their weighting factors were determined by the percentage of variation explained by the PCs (). The final normalized SQI equation was:
Table 4 Principal component analysis of soil parameters
Table 5 Correlation matrix between the different properties determined (sample size=18)
Table 6 Parameters for the scoring curves
shows the significant differences for SQI found among the different vegetations. Leucaena leucocephala had the highest SQI value of 0.705 and the lowest was found in the soil at the gully bed with Dodonaea viscosa (0.380). Compared to the values of the soils from Zuolin town, the soils from Yuanma town had generally higher SQIs. Relative to the bare soil from the Yuanma town site, revegetation of Leucaena leucocephala increased the value of SQI, whereas Eucalyptus camaldulensis decreased it. At the Zuolin town site, the SQI under three vegetations had no significant difference.
4. DISCUSSION
The maintenance of soil quality is critical for ensuring the sustainability of both the environment and the biosphere (Bastida et al. Citation2008). As we know, soil quality is a complex concept because it is determined by multiple parameters. There are two approaches that are mainly found for indicator selection in the literature: the opinion of experts (Doran and Parkin Citation1994; Bastida et al. Citation2008) and different mathematical-statistical systems such as regression equations and PCA (Andrews and Carroll Citation2001; Sharma et al. Citation2005). In our study, we used the synthetic soil quality index (SQI), which was based on a mathematical-statistical system (Andrews et al. Citation2002a), to assess the soil quality of the rhizosphere under different vegetation types in the Yuanmou dry-hot valley. Five parameters (OM, pH, MBN, TK and PA), which were considered to be the most important indicators based on the PCA analysis, were selected to calculate SQI. The variables involved traditional chemical parameters, biological indicators and soil enzyme activity, as suggested by previous investigators. The final SQI values implied that restoring Leucaena leucocephala greatly improved the soil quality, while restoration with Eucalyptus camaldulensis decreased the soil quality of the slope area. However, compared to the bare soil on gully bed, plant recovery with Dodonaea viscosa and Heteropogon contortus didn't improve the soil quality. Additionally, the SQI of the bare soil from the Yuanma site (slope area) was significantly larger than that from the Zuolin site (gully bed), which may be mainly attributed to the more intensive erosion in gully bed than on slope area. In general, SQI is useful in evaluating the soil quality under different revegetation types and aids in selection of the most suitable plant recovery types in dry-hot valleys.
Eucalyptus is known to be a fast-growing and drought-resistant woody plant, which is widespread in its use for plant recovery in China (Yang and Peng Citation2001; Zhao et al. Citation2006). However, the introduction of Eucalyptus is still a controversial issue and has triggered criticism surrounding understory plant diversity, soil fertility and soil biodiversity. In this study, three measured soil properties (pH, TN and TP; ) beneath Eucalyptus camaldulensis dramatically decreased and there was also a declining trend for AP, TK, and AK (). That is to say, restoring Eucalyptus camaldulensi led to soil nutrient depletion which resulted in soil degradation (Jagger and Pender Citation2003).
Microbial community populations (BCP, FCP and TCP) decreased in the rhizosphere soil of Eucalyptus camaldulensis (). The plant roots provide a habitat for a variety of microbes, and resource availability for microbial populations in the soil is determined by the amounts and types of organic compounds entering the soil from plant litter (Myers et al. Citation2001). Eucalyptus commonly produces litter with low nutrient concentrations which decomposes slowly (Guo and Sims Citation1999; Cao et al. Citation2010). Additionally, soil pH plays a dominant role in determining the composition of the general soil microbial community, e.g., that bacteria are favored compared to fungi at high pH, and vice versa (Hоgberg et al. Citation2007). Small populations of BCP and TCP are partly attributed to the low pH in the rhizosphere soil of Eucalyptus camaldulensis (). However, the population of FCP also decreased. We infer that the allelopathic substances of root exudates may be the reason that has led to the observed decreasing trend after 10 years' growth.
MBC content and urease activity in the rhizosphere soil of Eucalyptus camaldulensis decreased profoundly ( and ). The soil microbial biomass has been considered as an early indicator for changes in the SOM status (Powlson et al. Citation1987). The microbial biomass pool plays a key role in controlling the nutrient cycling and energy flow in terrestrial ecosystems (Jenkinson and Ladd Citation1981), notwithstanding its small size. The decreased in MBC content has been reported in other studies (Chander et al. Citation1995; Behera and Sahani Citation2003; Araujo et al. Citation2010). These studies suggested that a toxic compound (allelochemical) in the leaves of Eucalyptus caused the decrease in MBC, and the establishment of an exotic plant species had an initial negative effect on soil microbial biomass. Soil enzymes, as biological catalysts of almost all biochemical reactions, play important roles in the nutrient cycling and flux in the soil. Enzyme activities can be use as supplementary information in quantifying biological soil quality (Trasar-Cepeda et al. Citation2000; Sicardi et al. Citation2004). The activities of urease activity were closely related to the SOM, N and soil microbial C contents (), indicating that these properties were important for maintaining soil enzymatic activity (Salam et al. Citation1998).
Leucaena leucocephala is a fast-growing leguminous N-fixing tree. Due to its low requirements for growth conditions and environmental friendliness, Leucaena leucocephala has been a pioneer afforestation tree in dry-hot valleys (Bai et al. Citation2004). Many researchers consider it can improve soil quality through SOC sequestration, enhancement of soil fertility and compatibility with soil microbes (Isaac et al. Citation2003; Mutegi et al. Citation2008; Habte and Antal Citation2010). Relative to the bare soil and Eucalyptus camaldulensis, restoring Leucaena leucocephala to the slope area positively affected SOM content and N content (). C input and increase in soil N content can enhance the capacity of the system to support more complex community (Macedo et al. Citation2008; F.M. Wang et al. Citation2010). Soil microbial communities are responsible for various biogeochemical cycles and play pivotal roles in the cycling of organic compounds (Kirk et al. Citation2004). Larger populations of microbial communities implied the more active biogeochemical cycles beneath Leucaena leucocephala (). Valpassos et al. (Citation2007) made a similar comparison in an Atlantic forest in which they found that the microbial populations (bacteria, fungi, actinomycetes) in Leucaena leucocephala soil were significantly higher than was determined for bare soil. They attributed this result to different amounts of total organic C. In our study, BCP, ACP and TCP were closely related to organic matter, N and P content (), so we can see these parameters of the soil interacted with each other.
Gully bed plays an important role in sediment transportation, and vegetation in gully beds is efficient in trapping sediment (Burylo et al. Citation2007; Molina et al. Citation2009). Vegetation restoration with Dodonaea viscosa and Heteropogon contortus had obvious effects on the population of soil microbial communities (BCP, ACP and TCP), mainly due to the fine roots of the grass and shrub which provided a habitat for the microbes (X.R. Wei et al. Citation2009; C. Zhang et al. Citation2011b). However, restoring Dodonaea viscosa and Heteropogon contortus on gully beds did not increase the SQI, compared to the bare soil. This could be attributed to the sampling season and rehabilitation age. We sampled in March when the Dodonaea viscosa and Heteropogon contortus in the dry-hot valley were in a withering period. The changes of soil biochemical indicators in growing seasons and soil quality amelioration at different vegetation recovery stages still need to be further studied.
5. CONCLUSIONS
Different vegetation types established in Yuanmou dry-hot valley have a significant effect on the physicochemical and microbial properties of the rhizosphere soil. Compared to the soils collected from other plant types, the soil under Leucaena leucocephala had the highest OM, TN, AN, TP, AP, AK, MBC, BCP, ACP, TCP, and activities of catalase, protease and urease. Five parameters (OM, pH, MBN, TK and PA), which showed the greatest weight in PCA, were selected to calculate SQI. The final SQI values implied that restoration using Leucaena leucocephala greatly improved soil quality, while vegetation restoration with Eucalyptus camaldulensis decreased soil quality for slope area. Plant recovery with Dodonaea viscosa and Heteropogon contortus on gully beds affected the population of soil microbial communities but didn't improve the SQIs. Thus, it can be concluded that plants used for vegetation recovery in gully-developed regions in dry-hot valleys must be chosen cautiously: Leucaena leucocephala is a good choice for soil revegetation of the slope area, but the revegetation patterns on gully beds still need to be further studied.
ACKNOWLEDGMENTS
This work was financially supported by the Foundation of Key Laboratory of Mountain Hazards and Earth Surface Processes, Chinese Academy of Sciences and the Natural Science Foundation of China (Grant No. 40971168).
REFERENCES
- Andrews , SS and Carroll , CR . 2001 . Designing a soil quality assessment tool for sustainable agroecosystem management . Ecol. Appl. , 11 : 1573 – 1585 .
- Andrews , SS , Karlen , DL and Mitchell , JP . 2002a . A comparison of soil quality indexing methods for vegetable production systems in Northern California . Agr. Ecosyst. Environ. , 90 : 25 – 45 .
- Andrews , SS , Mitchell , JP , Mancinelli , R , Karlen , DL , Hartz , TK , Horwath , WR , Pettygrove , GS , Scow , KM and Munk , DS . 2002b . On-farm assessment of soil quality in California's central valley . Agron. J. , 94 : 12 – 23 .
- Araujo , ASF , Silva , EFL , Nunes , LAPL and Carneiro , RFV . 2010 . The effect of converting tropical native Savanna to Eucalyptus grandis forest on soil microbial biomass . Land Degrad. Dev. , 21 : 540 – 545 .
- Bai , DZ , Ji , ZH , Yang , YX , Fang , HD and Liao , CF . 2004 . Effect study of water and soil conservation on Leucaena harnessing area in rushing gully . Res Soil Water Conserv , 11 : 226 – 228 .
- Bastida , F , Moreno , JL , Hernandez , T and Garcia , C . 2006 . Microbiological degradation index of soils in a semiarid climate . Soil Biol. Biochem. , 38 : 3463 – 3473 .
- Bastida , F , Zsolnay , A , Hernandez , T and Garcia , C . 2008 . Past, present and future of soil quality indices: A biological perspective . Geoderma , 147 : 159 – 171 .
- Behera , N and Sahani , U . 2003 . Soil microbial biomass and activity in response to Eucalyptus plantation and natural regeneration on tropical soil . For. Ecol. Manage. , 174 : 1 – 11 .
- Bolan , NS , Baskaran , S and Thiagarajan , S . 1996 . An evaluation of the methods of measurement of dissolved organic carbon in soils, manures, sludges, and stream water . Commun. Soil Sci. Plant Anal. , 27 : 2723 – 2737 .
- Burns , RG . 1982 . Enzyme activity in soil: Location and a possible role in microbial ecology . Soil Biol. Biochem , 14 : 423 – 427 .
- Burylo , M , Rey , F and Delcros , P . 2007 . Abiotic and biotic factors influencing the early stages of vegetation colonization in restored marly gullies (Southern Alps, France) . Ecol. Eng. , 30 : 231 – 239 .
- Cao , YS , Fu , SL , Zou , XM , Cao , HL , Shao , YH and Zhou , LX . 2010 . Soil microbial community composition under Eucalyptus plantations of different age in subtropical China . Eur. J. Soil Biol. , 46 : 128 – 135 .
- Chander , K , Goyal , S and Kapoor , KK . 1995 . Microbial biomass dynamics during the decomposition of leaf litter of Poplar and Eucalyptus in a sandy loam . Biol. Fert. Soils , 19 : 337 – 362 .
- Chaparro , JM , Sheflin , AM , Manter , DK and Vivanco , JM . 2012 . Manipulating the soil microbiome to increase soil health and plant fertility . Biol. Fert. Soils , 48 : 489 – 499 .
- Chen , QB , Wang , KQ , Li , YM , Wang , JY , Wang , PL and Da , Q . 2003 . Effect of different vegetation types on soil amelioration in dry-hot valley of Jinshajiang River Basin . J. Soil Water Conserv , 17 : 67 – 70 .
- Dong , M . 1997 . Survey, Observation, and Analysis of Terrestrial Biocommunities , Beijing , , China : Standard Press of China .
- Doran , JW and Parkin , TB . 1994 . “ Defining and assessing soil quality ” . In Defining Soil Quality for a Sustainable Environment , Edited by: Doran , JW , Coleman , DC , Bezdicek , DF and Stewart , BA . 3 – 21 . Madison , WI : Soil Science Society of America .
- French , S , Levy-Booth , D , Samarajeewa , A , Shannon , KE , Smith , J and Trevors , JT . 2009 . Elevated temperatures and carbon dioxide concentrations: Effects on selected microbial activities in temperate agricultural soils . World J. Microbiol. Biotechnol. , 25 : 1887 – 1900 .
- Garcia , C , Roldan , A and Hernandez , T . 2005 . Ability of different plant species to promote microbiological processes in semiarid soil . Geoderma , 124 : 193 – 202 .
- Gregorich , EG , Carter , MR , Angers , DA , Monreal , CM and Ellert , BH . 1994 . Towards a minimum data set to assess soil organic-matter quality in agricultural soils . Can. J. Soil Sci. , 74 : 367 – 385 .
- Guo , LB and Sims , REH . 1999 . Litter decomposition and nutrient release via litter decomposition in New Zealand eucalypt short rotation forests . Agr. Ecosyst. Environ. , 75 : 133 – 140 .
- Habte , M and Antal , MJ . 2010 . Reaction of mycorrhizal and nonmycorrhizal Leucaena leucocephala to charcoal amendment of mansand and soil . Commun. Soil Sci. Plant Anal. , 41 : 540 – 552 .
- Hogberg , MN , Hogberg , P and Myrold , DD . 2007 . Is microbial community composition in boreal forest soils determined by pH, C-to-N ratio, the trees, or all three? . Oecologia , 150 : 590 – 601 .
- Isaac , L , Wood , CW and Shannon , DA . 2003 . Pruning management effects on soil carbon and nitrogen in contour-hedgerow cropping with Leucaena leucocephala (Lam.) De Wit on sloping land in Haiti . Nutr. Cycl. Agroecosyst. , 65 : 253 – 263 .
- Jagger , P and Pender , J . 2003 . The role of trees for sustainable management of less-favored lands: The case of eucalyptus in Ethiopia . Forest Policy Econ , 5 : 83 – 95 .
- Jenkinson , DS and Ladd , JN . 1981 . “ Microbial biomass in soil: Measurement and turnover ” . In Soil Biochemistry , Edited by: Paul , EA and Ladd , JN . 415 – 471 . New York : Marcel Dekker .
- Jin , ZZ and Ou , XK . 2000 . Yuanjiang, Nujiang, Jinshajiang, Lancangjiang Vegetation of Dry-Hot Valleys , Kunming , , China : Yunnan University Press .
- Kirk , JL , Beaudette , LA , Hart , M , Moutoglis , P , Khironomos , JN , Lee , H and Trevors , JT . 2004 . Methods of studying soil microbial diversity . J. Microbiol Meth. , 58 : 169 – 188 .
- Lal , R . 2001 . Soil degradation by erosion . Land Degrad. Dev. , 12 : 519 – 539 .
- Li , K , Zhang , CS , Ma , JM and Zheng , ZX . 2006 . Comparative research on soil fertility of different artificial plantations in Yuanmou dry-hot valley, Yunnan Province . For. Res , 19 : 574 – 579 .
- Lin , XG . 2010 . Principles and Methods of Soil Microbiology Research , Beijing : Higher Education Press .
- Lu , RK . 2000 . Chemical Analyzing Method on Soil Agriculture , Beijing : China Agriculture Science and Technology Press .
- Macedo , MO , Resende , AS , Garcia , PC , Boddey , RM , Jantalia , CP , Urquiaga , C , Campello , EFC and Franco , AA . 2008 . Changes in soil C and N stocks and nutrient dynamics 13 years after recovery of degraded land using leguminous nitrogen-fixing trees . For. Ecol. Manage. , 255 : 1516 – 1524 .
- Masto , RE , Chhonkar , PK , Singh , D and Patra , AK . 2008 . Alternative soil quality indices for evaluating the effect of intensive cropping, fertilisation and manuring for 31 years in the semi-arid soils of India . Environ. Monit. Assess , 136 : 419 – 435 .
- Molina , A , Govers , G , Cisneros , F and Vanacker , V . 2009 . Vegetation and topographic controls on sediment deposition and storage on gully beds in a degraded mountain area . Earth Surf. Proc. Land. , 34 : 755 – 767 .
- Mutegi , JK , Mugendi , DN , Verchot , LV and Kung'u , JB . 2008 . Combining napier grass with leguminous shrubs in contour hedgerows controls soil erosion without competing with crops . Agroforest Syst. , 74 : 37 – 49 .
- Myers , RT , Zak , DR , White , DC and Peacock , A . 2001 . Landscape-level patterns of microbial community composition and substrate use in upland forest ecosystems . Soil Sci, Soc, Am. J. , 65 : 359 – 367 .
- Nie , XJ , Zhang , JH , Liu , GC , Nan , L and Su , ZA . 2008 . Effects of vegetation restoration on soil quality of eroded hillslopes in dry-hot valley of Jinsha River . Ecol. Envir , 17 : 1636 – 1640 .
- Ollivier , J , Towe , S , Bannert , A , Hai , B , Kastl , EM , Meyer , A , Su , MX , Kleineidam , K and Schloter , M . 2011 . Nitrogen turnover in soil and global change . FEMS Microbiol Ecol. , 78 : 3 – 16 .
- Powlson , DS , Brookes , PC and Christensen , BT . 1987 . Measurement of soil microbial biomass provides an early indication of changes in total soil organic-matter due to straw incorporation . Soil Biol. Biochem. , 19 : 159 – 164 .
- Rillig , MC and Mummey , DL . 2006 . Mycorrhizas and soil structure . New Phytol. , 171 : 41 – 53 .
- Salam , AK , Katayama , A and Kimura , M . 1998 . Activities of some soil enzymes in different land use systems after deforestation in hilly areas of West Lampung, South Sumatra, Indonesia . Soil Sci. Plant Nutr , 44 : 93 – 103 .
- Sharma , KL , Mandal , UK , Srinivas , K , Vittal , KPR , Mandal , B , Grace , JK and Ramesh , V . 2005 . Long-term soil management effects on crop yields and soil quality in a dryland Alfisol . Soil Till. Res , 83 : 246 – 259 .
- Sicardi , M , Garcia-Prechac , F and Frioni , L . 2004 . Soil microbial indicators sensitive to land use conversion from pastures to commercial Eucalyptus grandis (Hill ex Maiden) plantations in Uruguay . Appl. Soil Ecol. , 27 : 125 – 133 .
- Sinha , S , Masto , RE , Ram , LC , Selvi , VA , Srivastava , NK , Tripathi , RC and George , J . 2009 . Rhizosphere soil microbial index of tree species in a coal mining ecosystem . Soil Biol. Biochem. , 41 : 1824 – 1832 .
- Trasar-Cepeda , C , Leiros , MC , Seoane , S and Gil-Sotres , F . 2000 . Limitations of soil enzymes as indicators of soil pollution . Soil Biol. Biochem , 32 : 1867 – 1875 .
- Valpassos , MAR , Maltoni , KL , Cassiolato , AMR and Nahas , E . 2007 . Recovery of soil microbiological properties in a degraded area planted with Corymbia citriodora and Leucaena leucocephala . Sci. Agr. , 64 : 68 – 72 .
- Vance , ED , Brookes , PC and Jenkinson , DS . 1987 . An extraction method for measuring soil microbial biomass-C . Soil Biol. Biochem. , 19 : 703 – 707 .
- Wang , FM , Li , ZA , Xia , HP , Zou , B , Li , NY , Liu , J and Zhu , WX . 2010 . Effects of nitrogen-fixing and non-nitrogen-fixing tree species on soil properties and nitrogen transformation during forest restoration in southern China . Soil Sci. Plant Nutr , 56 : 297 – 306 .
- Wang , KQ , Shen , YX , Chen , QB and Wang , ZH . 2004 . Soil water environment of artificial vegetations in Jinshajiang dry-hot valley . Chin. J. Appl. Ecol , 15 : 809 – 813 .
- Wei , XR , Shao , MG , Fu , XL , Horton , R , Li , Y and Zhang , XC . 2009 . Distribution of soil organic C, N and P in three adjacent land use patterns in the northern Loess Plateau, China . Biogeochemistry , 96 : 149 – 162 .
- Wei , YL , Guo , FF , Chen , AQ , Nan , L and Liu , GC . 2011 . Effects of vegetation restoration on soil enzyme activity in Yuanmou dry-hot valley, China . J. Agro-Environ. Sci , 30 : 100 – 106 .
- Wu , J , Joergensen , R , Pommerening , B , Chaussod , R and Brookes , P . 1990 . Measurement of soil microbial biomass C by fumigation-extraction-an automated procedure . Soil Biol. Biochem. , 22 : 1167 – 1169 .
- Wu , J , Lin , QM , Huang , QY and Xiao , HA . 2006 . Measurement of Soil Microbial Biomass and Its Application , Beijing : China Meteorological Press .
- Yang , MS and Peng , Y . 2001 . The present situation, processing and utilization prospects of Eucalyptus plantations in China . Eucalypt Sci. Technol , 1 : 1 – 6 .
- Zhang , C , Liu , GB , Xue , S and Song , ZL . 2011a . Rhizosphere soil microbial activity under different vegetation types on the Loess Plateau, China . Geoderma , 161 : 115 – 125 .
- Zhang , C , Xue , S , Liu , GB and Song , ZL . 2011b . A comparison of soil qualities of different revegetation types in the Loess Plateau, China . Plant Soil , 347 : 163 – 178 .
- Zhang , YC . 2005 . Study on ecological effects and mechanisms of soil remediation by restoring native herbage in a xerothermic valley region , 47 – 49 . Chongqing , China : Southwest Agriculture University . in Chinese
- Zhao , Y , Chen , XB and Jiang , CS . 2006 . Advances on studies of Leucaena Bentham in China . Chinese J. Trop. Agr , 26 : 55 – 63 .