Abstract
Salinity is a major agricultural problem in the world. Reportedly, the scavenging of reactive oxygen species (ROS) plays a pivotal role for tolerance to salinity stress. While sodium chloride (NaCl) is very toxic for most plant species, some chenopodiaceous halophytes show a dependence on sodium (Na) for growth stimulation. This suggests that chenopodiaceous halophytes have an effective anti-oxidative response system (ARS). In this study, we aimed to research the contribution that anti-oxidative enzymes, super oxide dismutase (SOD), catalase (CAT), ascorbic acid peroxidase (APX), and glutathione reductase (GR) have on the growth stimulation of chenopodiaceous halophytes. We cultivated three species of chenopodiaceous halophytes, Suaeda salsa (L.) Pall., Kochia scoparia (L.) Schrad. and Swiss chard (Beta vulgaris (L.) var. cicla) in various NaCl concentration of 5, 50, 200 and 400 mM. Up to 200 mM, the specimens had no significant decrease in dry weight, although the exogenous Na enhanced the content of Na in the leaves and limited the accumulation of other cations. Moreover, at 200 mM NaCl, S. salsa significantly increased dry weight, but K. scoparia and Swiss chard did not show much growth stimulation. ROS-scavenging enzymes might have played pivotal roles, because up to 200 mM NaCl, all of the plant species did not increase their content of the oxidative stress marker, malondialdehyde (MDA). Among all the species, only CAT activity significantly correlated with dry weight. Additionally, APX activity correlated only with dry weight of Swiss chard. However, the growth stimulation of S. salsa at 200 mM NaCl could not be accounted for by water content, MDA and chlorophyll content. This is the first suggestion of CAT-dependent growth stimulation of chenopodiaceous halophytes under NaCl-stress conditions.
INTRODUCTION
Salinity is one of the most serious problems for agricultural production (Wahid and Ghazanfar Citation2006; Belkheiri and Mulas Citation2013), leading to a limit of food production in the world. More than 23% of irrigated lands have been salinized (Tanji Citation1990) and its adverse effect is increasing (Ghanem et al. Citation2008). Excessive sodium chloride (NaCl), a major salt in these lands, causes many types of stress symptoms in plants, such as potassium (K) deficiency (Dodd et al. Citation2010; Fraile-Escanciano et al. Citation2010) and osmotic stress (Yang et al. Citation2008). Therefore, preventing crop yield reductions in salinized areas is one of the solutions to avoid a food crisis while the world population is rising. Cultivating highly salt-tolerant plants is considered an effective way to avoid yield reduction in salinized areas.
Chenopodiaceous plants generally tend to possess higher salt tolerance than other families of plants (Maas and Hoffman Citation1977), and sodium (Na) is an essential element for some of them (Wang et al. Citation2004). Moreover many chenopodiaceous halophytes are valuable as agricultural products (Mir et al. Citation1991; Kirkpatrick et al. Citation1999). Therefore, chenopodiaceous halophytes are promising plants for effective utilization of salinized irrigated lands.
To elucidate mechanisms to maintain or promote dry matter production of halophytes is considered useful to generate new transgenic salt-tolerant plants. Positive physiological responses to Na for dry matter production such as co-transport of pyruvic acid (Furumoto et al. Citation2011) and osmotic adjustment (Yang et al. Citation2008) have been reported, but little is known for chenopodiaceous halophytes about mechanisms by which dry matter production is protected against salinized conditions.
This study focused on the anti-oxidative response system (ARS) in chenopodiaceous halophyte under salinity conditions. Excessive Na enhances osmotic pressure in the rhizosphere and the inside of the plant body, and closes stoma causing emergence of reactive oxygen species (ROS)—singlet oxygen, super oxide radical (O2 −), hydrogen peroxide (H2O2) and hydroxyl radical (−OH)—diminishing plant growth (Hernández 1995; Asada Citation1999). Oxygen reduction by electrons emerging from photosystem I is the main source of ROS. Therefore, anti-oxidative enzymes scavenging O2 − (initial product) and H2O2 (product from O2 − in the scavenging process) play key roles to protect plant cell from oxidative stress.
Among the enzymes in ARS, super oxide dismutase (SOD), catalase (CAT), ascorbic acid peroxidase (APX) and glutathione reductase (GR) work not only for effective ROS scavenging but also to maintain photosynthesis capacity (Asada Citation1999). Reportedly, plants with inherently high antioxidative enzyme activities or highly activated enzymes by gene manipulation showed higher salt tolerance (Mittova et al. Citation2002; Tseng et al. Citation2007), suggesting that SOD, CAT, APX and GR play an important role to enhance salt tolerance. Therefore, there have been many reports about plant ARS under salinity conditions. However, plants examined for ARS to salinity have been mostly glycophytes, and little is known for chenopodiaceous halophytes under salinity conditions. Moreover, many chenopodiaceous halophytes are stimulated in their growth by NaCl conditions (Lu et al. Citation2002; Wang et al. Citation2001, Citation2004). It appears that chenopodiaceous halophytes have effective ARS supporting the promotion of growth by salt.
In this study, three species of chenopodiaceous halophytes, Suaeda salsa (L.) Pall., Kochia scoparia (L.) Schrad. and Swiss chard (Beta vulgaris var. cicla (L.)), were cultivated with varying concentrations of NaCl in the medium, and antioxidative enzyme activities were measured. This study unveiled that CAT activity had a strong correlation with the dry weight of the three species examined, suggesting that dry matter production was maintained by H2O2 scavenging.
MATERIALS AND METHODS
Cultivation and sampling
Seeds of S. salsa, K. scoparia and Swiss chard were sown and germinated in moistened vermiculite. Fourteen days after sowing, seedlings were transplanted into 4-L plastic pots filled with basic nutrient solution. Components of basic nutrient solution were according to the method of Yamanouchi et al. (Citation1987). At 14 d after transplanting, nutrient solution was gradually salinized with daily increments of NaCl concentration in the order of 5, 50, 200, and 400 mM NaCl to accustom plants to NaCl conditions. Specifically, at 14, 15, 16, and 17 d after transplanting, salinization was started for 400, 200, 50, and 5 mM NaCl plots, respectively. Then, at 17 d after transplanting, final NaCl concentrations of 5, 50, 200, and 400 mM were achieved and this time was defined as the initiation of NaCl treatments. S. salsa and K. scoparia were harvested at 11 and 12 d after initiation of NaCl treatments, respectively. Swiss chard was harvested at 4 d after NaCl treatments because leaves then started to wither. All plants were grown in a greenhouse in the Faculty of Agriculture, Tottori University. In this study, 5 mM NaCl was defined as the control imitating cation contents in the natural soil environment and avoiding Na-deficient stress.
Dry weight, leaf water and chlorophyll contents
Plant samples were separated into leaves, stems including petiole, and roots. After fresh weights of the three parts were measured, stems including petiole and roots were dried in an air-forced oven at 70°C and the dry weight was measured. Leaves were frozen in liquid nitrogen immediately after harvest. A part of the frozen leaves was freeze-dried and the dry matter ratio was calculated to determine total dry weight of leaves. Residues of frozen leaves were put into a deep freeze (–80°C) and stocked for further use.
Leaf water content was calculated by following equation:
To determine the chlorophyll content of the leaf, a leaf sample was homogenized in a mortar with ethanol (99.5%), adding some quartz sand. Absorbance of extracts was measured at 665 nm and 649 nm using a spectrophotometer (U-1800, HITACHI, Tokyo), and chlorophyll (a + b) content was calculated according to the equation by Wintermans and Mots (1965).
Mineral contents
Mineral contents were determined by the wet digestion method. Dried powered samples of 0.05–0.2 g were put into a flask with 15 mL of acid mixture (sulfuric acid (H2SO4):nitric acid (HNO3):perchloric acid (HClO4) = 1:10:4) and digested (350°C). Phosphorus (P) content was measured by the vanadomolybdate yellow method. K, calcium (Ca), magnesium (Mg), and Na were determined by an atomic absorption spectrophotometer (Z-2310, Hitachi, Tokyo).
Leaf malodialdehyde content
Malondialdehyde (MDA) content in leaves was measured as 2-thiobarbituric acid-reactive substances (TBARs) following the method of Ghanem et al. (Citation2008). We used only leaf samples, because excessive NaCl impairs electron transport in chloroplasts (Asada Citation1999). High MDA content indicates that plants were exposed to strong oxidative stress. Specific absorbance (532 nm) of TBARs was calculated subtracting non-specific absorbance (600 nm), and then MDA concentration was determined using its molar extinction coefficient (155 mM−1cm−1).
Crude enzyme extraction
A frozen leaf sample (0.3–0.4g) was ground using mortar and pestle with liquid nitrogen and homogenized with 5 mL 50 mM K-P Buffer containing 1 mM ascorbic acid (pH 7.8). After centrifugation of extracts (4°C, 15,000 g, 30 min), the supernatant was used for an enzyme activity assay.
CAT, APX, GR, and SOD activities
CAT, APX and GR activities in leaves were measured according to the modified method of Tanaka et al. (Citation1982). Specific absorbance of H2O2 (230 nm) was measured in 1 mL of assay mixture containing 100 mM K-P Buffer (pH 7.8), 20 mM H2O2 and 2% [volume/volume (v/v)] crude enzyme extracts, then CAT activity was determined as the amount of scavenged H2O2 per minute. Extinction coefficient (0.04 mM−1cm−1) was used in the calculation.
For measurement of APX activity, specific absorbance of L-ascorbic acid (290 nm) was measured in 1 mL of assay mixture containing 100 mM K-P Buffer (pH 7.8), 0.5 mM L-ascorbic acid and 2 % (v/v) crude enzyme extracts, then APX activity was determined as the amount of oxidized L-ascorbic acid per minute. Extinction coefficient (2.8 mM−1cm−1) was used in the calculation.
For GR activity, specific absorbance of oxidized form of NADPH (340 nm) was measured in 1mL of assay mixture containing 50 mM K-P Buffer (pH 7.8), 0.1 mM ethylenediaminetetraacetic acid (EDTA), 0.02 mM reduced nicotinamide adenine dinucleotide phosphate (NADPH), 0.02 mM oxidized form of glutathione, and 5 % (v/v) crude enzyme extract, then GR activity was determined as the amount of oxidized NADPH per min. Extinction coefficient (6.2 mM−1cm−1) was used in the calculation.
SOD activity in leaf was measured according to the modified method of Tanaka and Sugahara (Citation1980). One milliliter of crude enzyme extracts in a dialysis membrane was dialyzed in 500 mL of 10 mM K-P buffer (pH 7.8) for 12 h. The K-P Buffer (pH 7.8) was renewed every 3 h. Dialyzed enzyme extracts were used for SOD activity. Specific absorbance of oxidized cytochrome c (550 nm) was measured in 1 mL assay mixture containing 50 mM K-P Buffer (pH 7.8), 0.1 mM EDTA, 0.1 mM xanthine, 10 µM cytochrome c, 5 % enzyme liquid (v/v) and 1.6 × 10−2 U xanthine oxidase, then the ascent rate of absorbance (v) was determined, where V is the ascent rate of absorbance when 5% enzyme liquid (v/v) was replaced by distilled water. One unit was defined as v/V = 0.5. Activity of SOD was calculated by the following expression:
Protein assay
Protein content in crude enzyme extracts was measured by the Bradford method (Bradford Citation1976). Enzyme activity per protein was calculated using the protein content.
Statistical analyses
Statistics software, the SPSS statistical program, version 19.0 (IBM SPSS Inc, Tokyo, Japan) was used. Means were compared by Student-Newman-Keuls test at a 5% level of significance. Correlation coefficients were determined by Pearson test at a 5% level of significance.
RESULTS
Fresh and dry weight of shoot
In S. salsa, shoot dry weight significantly increased in 200 mM NaCl (). K. scoparia and Swiss chard significantly decreased at 400 mM NaCl; however, no significant change was observed at 5–200 mM NaCl. The effect of NaCl treatments on shoot fresh weight was almost the same as that on dry weight.
Figure 1 Effect of sodium chloride (NaCl) treatment on shoot fresh and dry weight of (a) and (d) Suaeda salsa (L.) Pall., (b) and (e) Kochia scoparia (L.) Schrad., (c) and (f) Swiss chard (Beta vulgaris (L.) var. cicla). Means of four replicates are shown with standard deviation. Differences are significant at P < 0.05; Student-Newman-Keuls test.
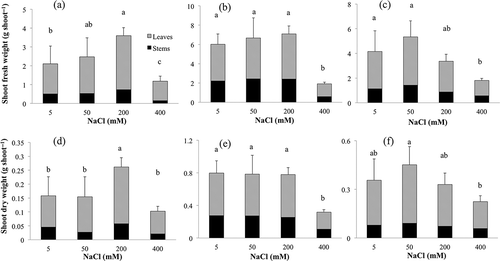
Leaf water content
Leaf water content of S. salsa tended to increase at 50 mM NaCl and decreased significantly at 400 mM NaCl (). In K. scoparia, it was significantly increased at 200 mM NaCl but was significantly decreased at 400 mM NaCl. Swiss chard decreased leaf water content at 400 mM NaCl.
Table 1 Effect of sodium chloride (NaCl) treatment on leaf water content (%)
Chlorophyll content
Leaf chlorophyll (a + b) content in S. salsa did not show a significant difference up to 200 mM NaCl but decreased significantly at 400 mM NaCl (). On the other hand, K. scoparia showed a continuous decrease in leaf chlorophyll (a + b) content with the increase of NaCl concentration. There was no significant difference in chlorophyll (a + b) content in Swiss chard among treatments.
Table 2 Effect of sodium chloride (NaCl) treatment on leaf chlorophyll content
Mineral contents
In S. salsa, Na content was significantly increased with the increase of NaCl concentration in all parts (). In the leaf, P, K, Ca, and Mg contents decreased significantly in higher NaCl treatments. Changes of the five elements above were intensive in the leaf, but sluggish in lower parts.
Table 3 Effect of sodium chloride (NaCl) treatment on phosphorus (P), sodium (Na), potassium (K), calcium (Ca) and magnesium (Mg) contents
In K. scoparia, Na content was significantly increased with the increase of NaCl concentration inall parts. Changes of P, Ca, and Mg affected by NaCl treatments were similar to those in S. salsa, although K content decreased up to 200 mM and increased again at 400 mM in leaf and stem, and continuously decreased with the increase of NaCl concentration in root.
In Swiss chard, only Na content in all parts was affected and increased significantly with the increase of NaCl concentration, but other elements hardly changed with changing NaCl treatments.
MDA content
In S. salsa, MDA content in leaf tended to increase at 50 mM NaCl, but did not show significant differences among NaCl treatments (). In K. scoparia, MDA content was almost constant up to 200 mM and increased significantly at 400 mM NaCl. In Swiss chard, MDA content significantly increased at 400 mM NaCl.
Figure 2 Effect of sodium chloride (NaCl) treatment on leaf malondialdehyde (MDA) content of (a) Suaeda salsa (L.) Pall., (b) Kochia scoparia (L.) Schrad. and (c) Swiss chard (Beta vulgaris (L.) var. cicla). Means of four replicates are shown with standard deviation. Differences are significant at P < 0.05; Student-Newman-Keuls test. FW, fresh weight.
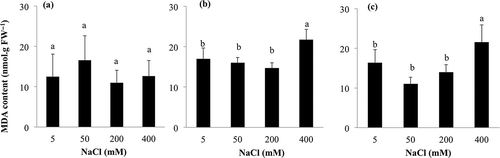
Antioxidant enzyme activities
In S. salsa, SOD activity was not significantly different among NaCl treatments; however, CAT activity significantly increased at 200 mM NaCl (). Activity of APX peaked at 50 mM and dropped significantly at higher NaCl concentrations, and GR activity also peaked at 50 mM, though activities between 5–50 mM were not significantly different, and then dropped significantly to 400 mM NaCl.
Figure 3 Effect of sodium chloride (NaCl) treatment on antioxidative enzymes (A) super oxide dismutase (SOD), (B) catalase (CAT), (C) ascorbic acid peroxidase (APX), (D) glutathione reductase (GR) activity in leaves. (a), (b) and (c) indicate Suaeda salsa (L.) Pall., Kochia scoparia (L.) Schrad. and Swiss chard (Beta vulgaris (L.) var. cicla), respectively. Means of four replicates are shown with standard deviation. Differences are significant at P < 0.05; Student-Newman-Keuls test.
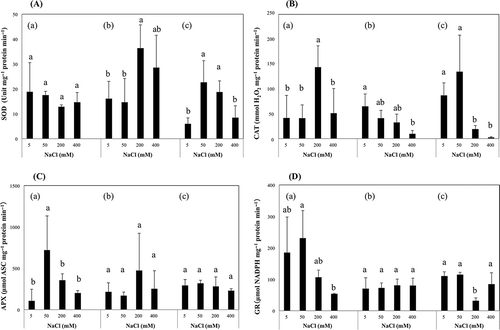
In K. scoparia, SOD activity increased significantly at 200 mM NaCl. CAT activity decreased gradually and significantly with the increase of NaCl concentration. There was no significant change in APX or GR activity.
In Swiss chard, SOD activities were significantly higher at 50 mM NaCl, and those were decreased gradually and significantly toward higher NaCl concentrations. CAT activities significantly decreased at 200–400 mM NaCl. The activity of APX was almost constant among NaCl treatments and GR activity significantly decreased only at 200 mM NaCl.
shows correlation coefficients between leaves or shoot dry weights and activities of anti-oxidative enzymes. Significant correlations were shown in the relationships between both dry weights and CAT activity in all three species, and between those and APX activity in Swiss chard.
Table 4 Correlation coefficients between (a) leaves, (b) shoot dry weights and antioxidative enzyme activities in leaves
DISCUSSION
In this study, we measured activities of antioxidant enzymes, SOD, CAT, APX and GR that play important roles, and observed the relationship between dry matter production and antioxidant enzyme activities. Moreover, mineral contents, chlorophyll content and MDA content were measured to unveil their roles in antioxidant enzymes.
S. salsa significantly increased dry weight at 200 mM NaCl (). Interestingly, water content, however, did not change significantly up to 200 mM NaCl (). This suggests that growth stimulation at 200 mM NaCl is independent of water content. S. salsa transported more Na to leaf than to stem including petiole (). Na may have played some role in the leaf. There were, however, competitive inhibitions in nutrient absorption by increasing NaCl concentration in the medium (). shows that leaf P content significantly decreased at 400 mM NaCl, suggesting that a significant decrease in P content was probably a factor to decrease dry weight. Mg is an essential element to synthesize chlorophyll (Cornah et al. Citation2003), and Mg content decreased continuously up to 200 mM NaCl. Chlorophyll (a + b) content was maintained up to 200 mM (), suggesting that Mg was not a limiting factor for chlorophyll synthesis.
Guard cells control turgor to open or close stomata loading K into the cells (Hafsi et al. 2011), while H2O2 in guard cell closes stomata (Jannat et al. Citation2012). Lu et al. (Citation2002) showed that S. salsa decreased transpiration rate with the increase of NaCl concentration in the medium. Leaf K content at 200 mM NaCl decreased to 38% of that at 5 mM NaCl (). It appears that S. salsa closed stomata due to the decrease of leaf K content, possibly resulting in the decrease of transpiration rate. Reportedly, stomatal closure by K deficiency limits carbon dioxide (CO2)-fixiation and increase of inverse effects by ROS (Hafsi et al. Citation2011). Therefore, it appears that antioxidant enzymes reduced inverse effects by ROS and protected from decrease of CO2-fixation capacity.
Since SOD scavenges O2 − having a high reactive character, SOD plays an important role to protect cells from oxidation and maintain photosynthesis capacity (Asada Citation1999). However, S. salsa showed no significant change in SOD activity (), which could be supported by a report that S. salsa increased Fe-SOD activity by NaCl treatment and Cu-SOD compensatively decreased, resulting in no significant change in total SOD activity (Wang et al. Citation2004). Catalase functions effectively in H2O2 scavenging (Jannat et al. Citation2012). CAT activity of S. salsa correlated significantly with dry weight (), suggesting that high CAT activity would be very important for dry matter production. Why did CAT activity correlate with dry weight? Interestingly, MDA and chlorophyll content did not show significant change up to 200 mM NaCl, although ROS causes lipid oxidation and chlorophyll degradation. It was reported that H2O2 causes not only cell tissue oxidation but also inhibition of CO2-fixation (Asada Citation1999). Enzymes relating to the Calvin cycle, nicotinamide adenine dinucleotide phosphate (NADP+) glyceraldehyde 3-phosohate dehydrogenase, fructose 1, 6-bisphosphatase, ribulose 5-phosphate kinase and sedoheptulose 1,7-bisphosphatase are sensitive to H2O2 (Kaiser Citation1979; Tanaka et al. Citation1982).
It was also reported that exogenous CAT scavenged H2O2 and recovered these enzyme activities, resulting in recovery of CO2-fixation capacity (Asada 1992). Moreover, spinach (Spinacia oleracea L.) belonging to the same family as S. salsa decreased CO2-fixation capacity when APX activity decreased (Tanaka et al. Citation1982). Therefore, it appears that scavenging H2O2 is necessary for CO2-fixation in growth stimulation of chenopodiaceous halophytes by NaCl. It was reported that S. salsa increased stability of photosystem II and CO2-fixation capacity by Na absorption (Lu et al. Citation2003). This suggests that stimulated CAT activity by NaCl treatment played a pivotal role in CO2-fixation of S. salsa. Activities of APX and GR didn't correlate with dry weight (). This suggests APX and GR weren't involved in growth stimulation by NaCl treatment. The reduction of APX and GR activities at 50–400 mM NaCl correlated with water content in leaves. This is similar to the behavior of APX and GR activities in seed of Quercus robur L. in drought-stress environments (Pukacka et al. Citation2011). It appears that APX and GR activity in S. salsa was affected by water content.
K. scoparia maintained dry weight up to 200 mM. However, increase of Na content and decrease of Mg content in leaf were clearer than those in stem including petiole and root (). Mg content in K. scoparia leaf was much lower than that in S. salsa, suggesting that decrease of Mg content was a factor to decrease chlorophyll content. Significant decrease of K content in root at higher NaCl concentration suggested inhibition of K absorption by Na (). Our laboratory previously demonstrated that K. scoparia decreased transpiration with the increase of NaCl concentration in the medium as does S. salsa (unpublished data), suggesting that K. scoparia closed stomata due to K deficiency at least up to 200 mM. This condition possibly promoted ROS emergence; therefore, ARS would have been essential for the maintenance of dry weight.
Activity of SOD in K. scoparia significantly increased at 200 mM NaCl (). Since there was no significant change in leaf MDA content (), it appears that SOD contributed to protect cell tissues from oxidative stress. Activity of CAT in K. scoparia also showed significant correlation with dry weight (), which might be due to protection from oxidative stress and maintenance of CO2-fixation like S. salsa. Activity of CAT significantly decreased at 400 mM NaCl while MDA content significantly increased (), suggesting that CAT activity was insufficient to scavenge H2O2. Moreover, the decrease of chlorophyll content in K. scoparia leaf was correlated with the decrease of CAT activity, suggesting that CAT protected chlorophyll from oxidative stress like S. salsa.
Changes of APX and GR activities did not accompany those of dry weight or decrease of MDA contents. This suggests that APX and GR are less effective than SOD and CAT in salinity.
Swiss chard trended to increase dry weight at 50 mM (), suggesting that Swiss chard would be a halophilic plant. However, a treatment term of 4 d, was not long enough to observe significant changes. Swiss chard showed increase in leaf Na content; however, there were no significant changes in other mineral contents in leaf (). It might be due to the shorter treatment term than those of S. salsa and K. scoparia. Accordingly, maintenance of Mg contents leaded to stable chlorophyll content ().
Activity of SOD in Swiss chard correlated with MDA content, suggesting that SOD contributed to alleviate oxidative stress. Activity of CAT had a significant correlation with dry weight as well as S. salsa and K. scoparia (), which might be due to the anti-oxidative effect and maintenance of CO2-fixation. At 400 mM NaCl, loss of CAT activity might be a factor to allow MDA content. Activity of APX in Swiss chard leaf also had a significant correlation with dry weight (). It seemed that APX localizing in chloroplasts scavenged H2O2 which maintained CO2-fixation capacity. Moreover, APX activity correlated with water content; therefore, maintenance of water content might be essential to preserve APX activity.
Activity of CAT significantly correlated with dry weight in the three species examined; however, APX activity only in Swiss chard correlated with dry weight (). Therefore, it could be obvious that chenopodiaceous halophytes have common features to enhance CAT activity to preserve dry matter production under NaCl conditions. This prompted the next question: why they elevated CAT but not APX. As previously described, CAT is more tolerant to oxidative stress than APX (Asada Citation1999). It appears that chenopodiaceous halophytes developed a strategy to enhance CAT activity through the course of evolution, because APX easily loses activity in stress condition. Shikanai et al. (Citation1998) reported that Nicotiana tabacum L. introduced with the gene for CAT of Escherichia coli with low Km value showed maintenance of CO2-fixation capacity under drought stress conditions with the strength to deactivate APX activity, which supports the idea that CAT in this study contributed to maintenance of CO2-fixation. Moreover, a recent study unveiled that the AM fungus, Glomus mosseae, enhanced CAT activity in S. salsa at 400 mM NaCl and led to higher salt tolerance (Li et al. Citation2012). These results support us in hypothesizing that CAT plays a pivotal role in dry matter production.
The result of this study showed that dry matter production of chenopodiaceous halophytes significantly correlated with CAT activity under NaCl conditions. We attributed that to efficient H2O2 scavenging, mainly by CAT, which enhanced enzyme activities involved with CO2-fixation in chenopodiaceous halophytes. This study suggests CAT is a new Na-dependent growth stimulation factor. Providing high CAT activity to chenopodiaceous plants using gene modification could possibly lead to much higher salt-tolerance and yield on the future salinized irrigated lands in the world. It must be further investigated whether increased CAT activity can really contribute to the activities of enzymes relating to CO2-fixation capacity in the Calvin cycle.
ACKNOWLEDGMENTS
We sincerely thank Mr. Bryan Worrell, Department of Computer Science and Engineering, University of Nevada, Reno, for English corrections.
REFERENCES
- Asada , K . 1999 . The water-water cycle in chloroplasts: Scavenging of active oxygens and dissipation of excess photons . Annu. Rev. Plant Physiol. Plant Mol. Biol. , 50 : 601 – 639 .
- Belkheiri , O and Mulas , M . 2013 . The effects of salt stress on growth, water relations and ion accumulation in two halophyte Atriplex species . Environ. Exp. Bot. , 86 : 17 – 28 .
- Bradford , MM . 1976 . A rapid and sensitive method for the quantitation of microgram quantities of protein utilizing the principle of protein-dye binding . Annu. Biochem. , 72 : 248 – 254 .
- Cornah , JE , Terry , MJ and Smith , AG . 2003 . Green or red: What stops the traffic in the tetrapyrrole pathway? . Trends Plant Sci , 8 : 224 – 230 .
- Dodd , K , Guppy , C , Lockwood , P and Rochester , I . 2010 . The effect of sodicity on cotton: Plant response to solutions containing high sodium concentrations . Plant Soil , 330 : 239 – 249 .
- Fraile-Escanciano , A , Kamisugi , Y , Cuming , AC and Rodríguez-Navarro , A . 2010 . The SOS1 transporter of Physcomitrella patens mediates sodium efflux in planta . New Phytol. , 188 : 750 – 761 .
- Furumoto , T , Yamaguchi , T and Ohshima-Ichie , Y . 2011 . A plastidial sodium-dependent pyruvate transporter . Nature , 476 : 472 – 475 .
- Ghanem , ME , Albacete , A , Martines-Andujar , C , Acosta , M , Romero-Aranda , R , Dodd , IC , Lutts , S and Perez-Alfocea , F . 2008 . Hormonal changes during salinity-induced leaf senescence in tomato (Salanum lycopersicum L.) . J. Exp. Bot. , 59 : 3039 – 3050 .
- Hafsi , C , Atia , A , Lakhdar , A , Debez , A and Abdelly , C . 2011 . Differential responses in potassium absorption and use efficiencies in the halophytes Catapodium rigidum and Hordeum maritimum to various potassium concentrations in the medium . Plant Prod. Sci. , 14 : 135 – 140 .
- Hernandez , JA , Olmos , E , Corpas , FJ , Sevilla , F and del Rio , LA . 1995 . Salt-induced oxidative stress in chloroplasts of pea plants . Plant Sci. , 105 : 151 – 167 .
- Jannat , R , Uraji , M , Hossain , MA , Islam , MM , Nakamura , Y , Mori , IC and Murata , Y . 2012 . Catalases negatively regulate methyl jasmonate signaling in guard cells . J. Plant Physiol. , 169 : 1012 – 1016 .
- Kaiser , WM . 1979 . Reversible inhibition of Calvin cycle and activation oxidative of pentose phosphate cycle in isolated intact chloroplasts by hydrogen peroxide . Planta , 145 : 377 – 382 .
- Kirkpatrick , JG , Helman , RG , Burrows , GE , von Tungeln , D , Lehenbauer , T and Tyrl , RJ . 1999 . Evaluation of hepatic changes and weight gains in sheep grazing Kochia scoparia . Vet. Hum. Toxicol. , 41 : 67 – 70 .
- Li , T , Liu , RJ , He , XH and Wang , BS . 2012 . Enhancement of superoxide dismutase and catalase activities and salt tolerance of euhalophyte Suaeda salsa L. by mycorrhizal fungus Glomus mosseae . Pedosphere , 22 : 217 – 224 .
- Lu , CM , Qiu , NW , Lu , QT , Wang , BS and Kuang , TY . 2002 . Does salt stress lead to increased susceptibility of photosystem II to photoinhibition and changes in photosynthetic pigment composition in halophyte Suaeda salsa grown outdoors? . Plant Sci , 163 : 1063 – 1068 .
- Lu , CM , Qiu , NW , Wang , BS and Zhang , JH . 2003 . Salinity treatment shows no effects on photosystem photochemistry, but increases the resistance of photosystem to heat stress in halophyte Suaeda salsa . J. Exp. Bot. , 54 : 851 – 860 .
- Maas , EV and Hoffman , GL . 1977 . Crop salt tolerance-current assessment . ASCE J. Irrig. Drain. Div. , 103 : 115 – 134 .
- Mir , Z , Bittman , S and Townleysmith , L . 1991 . Nutritive-value of Kochia (Kochia scoparia) hay or silage grown in black soil zone in Northeastern Saskatchewan for sheep . Can. J. Anim. Sci. , 71 : 107 – 114 .
- Mittovaa , V , Talb , M , Volokitaa , M and Guya , M . 2002 . Salt stress induces up-regulation of an efficient chloroplast antioxidant system in the salt-tolerant wild tomato species Lycopersicon pennellii but not in the cultivated species . Physiol. Plant. , 115 : 393 – 400 .
- Pukacka , S , Malec , M and Ratajczak , E . 2011 . ROS production andantioxidative system activity in embryonic axes of Quercus robur seeds under different desiccation rate conditions . Acta Physiol. Plant. , 33 : 2219 – 2227 .
- Shikanai , T , Takeda , T , Yamauchi , H , Sano , S , Tomizawa , K , Yokota , A and Shigeoka , S . 1998 . Inhibition of ascorbate peroxidase under oxidative stress in tobacco having bacterial catalase in chloroplasts . FEBS Lett. , 428 : 47 – 51 .
- Tanaka , K , Otubo , T and Kondo , N . 1982 . Participation of hydrogen peroxide in the inactivation of Calvin-cycle SH enzymes in SO2-fumugated spinach leaves . Plant Cell Physiol. , 36 : 1089 – 1095 .
- Tanaka , K and Sugahara , K . 1980 . Role of superoxide dismutase in defense against SO2 toxicity and an increase in superoxide dismutase activity with SO2 fumigation . Plant Cell Physiol. , 21 : 601 – 611 .
- Tanji , KK . 1990 . “ Nature and extent of agricultural salinity ” . In Agricultural Salinity Assessment and ManagementAmerican Society of Civil Engineers Edited by: Tanji , KK . 1 – 18 . New York
- Tseng , MJ , Liu , CW and Yiu , CJ . 2007 . Enhanced tolerance to sulfur dioxide and salt stress of transgenic Chinese cabbage plants expressing both superoxide dismutase and catalase in chloroplasts . Plant Physiol. Biochem. , 45 : 822 – 833 .
- Wahid , A and Ghazanfar , A . 2006 . Possible involvement of some secondary metabolites in salt tolerance of sugarcane . J. Plant Physiol. , 163 : 723 – 730 .
- Wang , B , Luttge , U and Ratajczak , R . 2001 . Effects of salt treatment and osmotic stress on V-ATPase and V-PPase in leaves of the halophyte Suaeda salsa . J. Exp. Bot. , 52 : 2355 – 2365 .
- Wang , B , Luttge , U and Ratajczak , R . 2004 . Specific regulation of SOD isoforms by NaCl and osmotic stress in leaves of the C3 halophyte Suaeda salsa L . J. Plant Physiol. , 161 : 285 – 293 .
- Wintermans , J and De Mots , A . 1965 . Spectrophotometric characteristics of chlorophyll a and b and their pheiphytins in ethanol . Biochim. Biophys. Acta. , 109 : 448 – 453 .
- Yamanouchi , M , Maeda , Y and Nagai , Y . 1987 . The mechanism of salinity tolerance. Relationship between the varietal difference of salinity tolerance and characteristics of absorption and translocation of sodium ion, 1: Rice plant . J. Soil Sci. Plant Nutr. , 58 : 591 – 594 .
- Yang , C , Shi , D and Wang , D . 2008 . Comparative effects of salt and alkali stress on growth, osmotic adjustment and ionic balance of an alkali-resistant halophyte Suaeda glauca (Bge.) . Plant Growth Regul. , 56 : 179 – 190 .