Abstract
During the early time after transplanting, the ability of nitrogen (N) uptake in roots of tree stocks is crucial to their future performance. However, it is not clear about the relationship between size or nutrient reserves in nursery-cultured tree seedlings and root N flux (N flow within or out of root) after transplanting. In this study, we pre-fertilized Changbai larch (Larix olgensis Henry.) seedlings with three top-dress applications [N-phosphorus (P)-potassium (K), 20-4-10] at rates of 0 (control), 31.58 (E-Low), 200 (E-Medium), and 525.08 (E-High) kg N ha–1 to different levels of nutrient storage during the first growing season. After being graded into large and medium sizes, stock N status was indicated to be discriminative in response to pre-fertilization by vector analysis. Overwinter stocks were transplanted into aerated distilled water or nutrient solution to detect root N influx or N efflux, respectively, at the second and fourth weeks under laboratory conditions. Larger stocks had higher net N influx and efflux rates. In large stocks, N influx tended to increase within one month’s time in the E-Medium and E-High treatments, whereas N efflux tended to rise as well. In pre-transplanted stocks, root biomass linearly correlated with both N influx and N efflux, but root N concentration only correlated with N influx for large stocks. In conclusion, proper nursery fertilization could induce seedling N reserves, which would result in a higher N influx rate for larger stocks, whose roots, however, would also risk more N efflux in humid but infertile sites during the early time after planting.
INTRODUCTION
The field performance of nursery-cultured tree seedlings determines the success of reforestation. Use of large stocks for reforestation is restricted by budget and the relative benefits for their establishment. Larger stocks have been widely proven to survive and grow better in the Mediterranean region (Oliet et al. Citation2009; Cuesta et al. Citation2010a, Citation2010b), boreal areas (Jobidon et al. Citation2003), eastern Asia (Li et al. Citation2011, 2013), and humid temperate regions (Pinto et al. Citation2011). However, although the ability for initial nutrient uptake and assimilation in newly planted stocks was crucial to their future performance (Timmer Citation1996), quite a few studies have found a relationship between pre-transplanting stock size and nutritional characteristics in post-transplanted stocks.
In newly out-planted stocks, abundant initial nutrient storage has a positive effect on bud flush (van den Driessche Citation1985; Fløistad and Kohmann Citation2004), new root extension (Cuesta et al. Citation2010a) and initial nutrient retranslocation (Salifu and Timmer Citation2003), which can help to overcome the infertility shock (Timmer Citation1996). Larger and better-fertilized seedlings have been demonstrated to have greater organ growth, gas exchange, carbon (C) cycling and higher pre-dawn shoot water potential (Cuesta et al. Citation2010a, Citation2010b). However, we are unaware whether better pre-fertilized larger stocks have a higher rate of nutrient influx after out-planting. For a given species, nutrient uptake capacity appears to be determined by seedling hereditary traits, nutrient status and current nitrogen (N) availability (Miller and Hawkins Citation2007; Yanai et al. Citation2009), but there is no evidence indicating a significant effect of stock size on nutrient influx in roots.
During N influx, strong N efflux may occur at the same time (Kronzucker et al. Citation1997; Pritchard and Guy Citation2005), which may originate from active or passive release of soluble materials from intact cells, sloughing and decomposition of epidermal cells, release of cell contents via lysis by pathogens, and senescence of roots (Brophy and Heichel Citation1989). Release of nutrients with these substrates from tree seedling roots has important physiological and ecological implications. Newly planted stocks usually suffer soil hydraulic and nutrient stresses (Grossnickle Citation2005), which may stimulate inordinate nutrient exudation (Nambiar and Fife Citation1991). Evidence from agricultural crops has demonstrated that larger roots exude more N compounds (Shepherd and Davies Citation1994), but no investigation has determined the effect of tree stock size on nutrient efflux. Although moderate root exudation could improve the performance of some plantations (Yang et al. Citation2010), in infertile sites initial nutrient leachate loss after out-planting may influence success of reforestation.
Seedling grading is a popular and operational practice at the end of nursery culture before seedling quality evaluation. Larger transplanted stocks graded out from one seedling batch were demonstrated to perform better in the field (Jacobs et al. Citation2005; Li et al. Citation2011). The results, however, are not in agreement with all tree species (Jacobs et al. Citation2005). Changbai larch (Larix olgensis Henry.) is widely distributed and planted in the temperate areas of the Changbai Mountains, Northeast China (42°23′N, 128°6′E), Olga bay in the Sea of Japan (43°41′N, 135°15′E), eastern Asiatic Russia (45°18′N, 137°6′E), and North Korea (41°52’N, 128°23′E). Current studies have documented nutritional responses of Changbai larch seedlings to nursery fertilization, such as nutrient reserves establishment (Wei et al. Citation2012) and out-planting performance (Li et al. Citation2011, 2013). According to these studies, it has been concluded that traditional fertilizer application could not create full nutrient reserves at the end of nursery culture (Wei et al. Citation2012; Li et al. Citation2013) and transplanted larger stocks performed better in shoot growth and survival (Li et al. Citation2011, Citation2013). The results, however, are not consistent, and no research has linked nutrient reserves within different sizes of seedlings with their nutritional performance after transplanting.
N usually limits tree growth. In wetlands, where spring waterlogging events are frequent (Girardin et al. Citation2001) and N availability is variable owing to anaerobic conditions caused by the high water table and low soil temperature (Islam and Macdonald Citation2005), larch is one of the dominant tree species, but its survival and growth have been found to be decreased by the poor soil N conditions and waterlogging (Roy et al. Citation2000). According to current evidence, we are not aware whether larger and better-fertilized larch seedlings are suitable for forestation in wetlands. In this study, we pre-fertilized bare-root Changbai larch seedlings using modified exponential fertilization to various N storage levels at the end of nursery culture, and then investigated their newly-transplanted N flux (influx and efflux) under laboratory conditions. The two stock types were selected by grading according to local criteria at the end of nursery culture (Li et al. Citation2011). A hydroponic system was employed to facilitate determination of N flux in a simulated wetland for transplanting. Distilled water and nutrient-supplied solution were used to simulate infertile and fertile conditions, respectively. We investigated the early time after transplanting which is harsh and crucial for tree seedling establishment (Timmer Citation1996; Grossnickle Citation2005; Zhu et al. Citation2008). Our objectives were to determine: (a) whether seedlings with different sizes could be discriminative in N reserves by three-time exponential fertilization at the end of nursery culture; (b) what is the relationship between stock size and N flux in seedlings with various initial nutrient storages, and (c) whether larger stocks would have higher N efflux.
MATERIALS AND METHODS
This study comprised a nursery pre-fertilization phase followed by a hydroponic experiment phase. Time lines, treatment, sampling and harvest schedules were summarized (). Details of seedlings culture and experiment procedures are described next.
Table 1 Two-year schedule of treatments and measurements for experiments during nursery phase and hydroponic phase with Changbai larch (Larix olgensis Henry.) seedlings
Seedling material (from May to mid-June 2009)
Seeds of Changbai larch were collected by employees of Xiaobeihu Forest Station (128°28′E, 44°03′N), Heilongjiang Province, Northeast China. After collection, seeds were transported to Jiangmifeng Nursery (43°45′N, 126°45′E), Jilin City, Jilin Province, Northeast China, and stored at 0–4°C. In Jilin, the annual precipitation is 650–750 mm with less than 200 mm from May to mid-June, and the mean annual temperature is 3–5°C with the average early growing season temperature ranging from 4 to 9°C.
Prior to seeding, 12 randomly located soil cores (inner diameter 4 cm) were collected from the nursery bed (0–20 cm depth), and soil analysis was conducted on a bulked sample from the cores, according to the methods of Wei et al. (Citation2012). This analysis indicated an organic C content of 1.73 %, a pH of 5.8, and extractable P and K concentrations of 18.49 and 33.56 mg kg–1, respectively, in soils. Also, ammonium-nitrogen (NH4+-N) and nitrate-nitrogen (NO3–-N) concentrations of the soil were 61.72 mg kg–1 and 173.73 mg kg–1, respectively, from which available soil N was estimated as 556.18 kg ha–1. Before sowing, seeds were soaked in a 5% potassium permanganate [weight/weight (w/w)] solution for 24 h and stratified for 5 d at 0–4°C. Seeds were sown at a density of 700 seeds m–2 in a nursery bed of Jiangmifeng nursery on 3 May 2009; then in mid-June 2009, germinated seedlings were thinned to 550 seedlings m–2.
Pre-fertilization treatment (from 5 July to 10 October 2009)
In order to create differing levels of nutrient reserves within seedlings at the end of nursery culture, we fertilized the seedlings at a wide range of N supply rates, plus an unfertilized control. The fertilizers were applied using an exponential function (Duan et al. 2013):
where Nt is the amount of N to be added at time for a given relative addition rate r. Ns is the initial quantity of N in the germinant seedlings, which was determined to be 5.36 kg N ha–1. N(t-1) is the cumulative amount of N added, up to and including the last fertilizer addition. In northeastern China, bare-root Changbai larch seedlings are operationally fertilized with top-dress granular fertilizers (20-4-10), usually three times during summer, and we performed this local protocol.
In order to calculate the relative addition rate in exponential treatments, r, another equation was used:
where NT is the desired amount of N to be added over the number of fertilization applications. The operational fertilization rate for Changbai larch seedlings in northeastern China is approximately 200 kg N ha–1 over a growing season (Wei et al. Citation2012), therefore this was chosen as the total amount of N added in the medium exponential (E-Medium) treatment. In the low exponential (E-Low) treatment, it was assumed that all N initially present in the soil could be taken up by the seedlings, thus NT was calculated to be 31.58 kg N ha–1 [593.12 kg N ha–1 (total N uptake in 5500 seedlings ha–1; unpublished pre-investigation) – 556.18 kg available N ha–1 in soil – 5.36 kg N ha–1 in germinants]. In the high exponential (E-High) treatment, a very high rate of fertilizer application was desired, therefore it was assumed that very little of the soil N would be taken up and NT was set at 525.08 kg N ha–1.
Fertilizer was applied as three top-dress applications of a solution of ammonium sulfate ((NH4)2SO4) and dipotassium phosphate (K2HPO4) (N-P-K, 20-4-10) on 5, 16, and 25 July 2009. These dates for application were chosen practically according to local top-dress application protocol and specific weather conditions (Li et al. Citation2013). There were three randomly located replicate plots of each treatment within the nursery bed. Each plot had an area of 1 m × 1 m with 0.1–0.2 m buffers between plots and plastic barriers inserted to a depth of 0.2 m within each buffer to eliminate lateral movement of fertilizer between plots. Weed control was conducted manually and irrigation was performed by automatic sprayers installed along the seed beds. These cultural practices depended on seedling growth rates and weather conditions throughout the growing season until September 2009.
Seedling sampling and determination
On 10 October 2009, 384 seedlings, 16 seedlings for each size category per plot, were randomly selected according to local morphological grading criteria [large: height 11–16 cm, root collar diameter (RCD) 3–4 mm; medium: height 6–11 cm, RCD 2–3 mm], and excavated. These seedlings were chosen because their size met the local requirement for transplanting for one more year (Li et al. Citation2011). All seedlings were sealed in plastic bags and transported to the laboratory on ice (0–2°C) where roots were washed free of soil.
Two hundred and forty seedlings for each size category per 2009 plot were divided into shoots and roots at the cotyledon scar, and then shoots were divided into needles and stems. For each of the four seedling parts, samples were bulked by size class and plot, weighed for their dry biomass after oven-drying for 48 h at 70°C, ground to pass a 1-mm sieve, and analyzed for seedling N concentration with 0.2 g sample digested in 5 mL solution of hydrogen peroxide (H2O2) and sulfuric acid (H2SO4). The digestion solution was diluted to 50 mL. Seedling N concentration was measured using 5 mL of diluted digest solution with an automatic N analyser (UDK 152 automatic N analyser, VELP Co., Usmate [MB], Italy) (Wei et al. Citation2012).
Hydroponic experiment (from 28 April to 26 May 2010)
On 28 April 2010, 144 seedlings, six seedlings per nursery plot, were moved from cold storage and transported to the Key Laboratory of Forest Silviculture and Conservation, Ministry of Education, Beijing Forestry University, Beijing, China. The overwinter storage protocol for Changbai larch is typical in northeastern China (Li et al. Citation2011, Citation2013). Pairs of seedlings of the same size from one 2009 plot were placed in a 750-mL or 130-mL volume container, filled with 700 or 100 mL solution and covered with an opaque plate or a small lump of sponge for large or medium seedlings, respectively. All containers were filled with aerated nutrient solution or distilled water to simulate fertile or infertile conditions, respectively. Mineral concentrations of nutrient solution included: ammonium nitrate (NH4NO3) 4 mmol, K2HPO4 0.5 mmol, potassium chloride (KCl) 0.5 mmol, calcium chloride (CaCl2) 1 mmol, magnesium sulfate (MgSO4·7H2O) 0.6 mmol, ferric chloride (FeCl3·6H2O) 20 μmol, manganese chloride (MnCl2·4H2O) 6 μmol, boric acid (H3BO3) 16 μmol, zinc chloride (ZnCl2) 0.3 μmol, cupric chloride (CuCl2·2H2O) 0.3 μmol, sodium molybdate (NaMoO4·2H2O) μmol. There were two seedling pairs per solution type per nursery fertilization treatment. Every 7 d both the nutrient solution and distilled water were replaced.
Throughout the hydroponic experiment, air temperature was maintained at 24/20°C (day/night) by an air conditioner and relative humidity was 40–50%. Photoperiod was prolonged to 18 h with a night photosynthetic photon flux density (PPFD) of 100–150 μmol m–2 s–1 measured at the tops of seedlings. Light was provided by a 125-W PhytoliteTM plant growth lamp (Oudi Co., Huzhou, Zhejiang, China). The 18-h photoperiod has been considered sufficient for seedling active growth (Roberts and Zwiazek Citation2001), and PPFD from 100 to 150 μmol m–2 s–1 has been measured as the natural light characteristic for juvenile Changbai larch seedlings growing in forests (Zhu et al. Citation2008). Every 3–4 d, all containers were rearranged randomly and the pH of the solution in each container was adjusted to 5.5–6.
On 12 and 26 May 2010, at the end of the second (from 5 to 12 May) and fourth (from 19 to 26 May) weeks of the solution experiment, the volume of liquid left in each container was measured, then at least 50 mL liquid was collected for subsequent N concentration analysis using the method described above.
Data calculation and analysis
Net N flux in roots in the hydroponic experiment was calculated on 12 and 26 May 2010, as follows:
where FN was net N flux (net N efflux in distilled water or net N influx in nutrient solution) in one container, C2 and C1 were N concentration (mg L–1) in nutrient solution or distilled water on date t2 (terminal) and t1 (initial), respectively, and V2 and V1 were liquid volume at t2 and t1, respectively. The specific net N flux for either seedling was determined by half of the FN value for each container.
All data were analyzed by SAS® 9.0 (SAS Institute Inc., NC, USA). For pre-transplanting seedlings, effects of nursery grading (G), nursery fertilization treatment (F), and their interaction (G × F) were tested with analysis of variance (ANOVA) carried out by the General Linear Model (GLM) procedure on biomass and N concentration of needles, stems, and roots, which were all calculated from the bulked measurements of 10 large or medium seedlings from each 2009 plot. Vector analysis involves comparing nutrient concentration, nutrient content and biomass in a graphic format know as a vector diagram, where vector directions could be used to evaluate nutritional symptoms in plants. Results of biomass and N status were used to evaluate the effect of pre-fertilization on initial stock N status according to the vector diagnosis method adapted from Salifu and Timmer (Citation2003) and Salifu and Jacobs (Citation2006). For the 2010 hydroponic experiment, values of net N influx and efflux rates were averaged for three containers in one pre-fertilization treatment. Then these values were compared separately for each of the two stock-types. All factors were considered fixed. When differences were indicated to be significant, Tukey’s studentized range test was used to determine significant differences for each effect and interaction (P = 0.05). Pearson regression was performed by the Corr procedure of SAS® to test the correlation between pre-transplanting root biomass or root N concentration and post-transplanting N flux. When regression was detectable, linear correlation was employed by Sigmaplot® (Systat Software Inc., Chicago, IL, USA) using scatter splashes of means ± standard error in pre-fertilization treatments.
RESULTS
Seedling response to pre-fertilization
Pre-fertilization had a significant effect on needles and stems (). For large stocks, compared to the control, the E-Low and E-Medium treatments increased needle biomass by 76% and 141%, respectively (P = 0.0002), while these two treatments increased stem biomass by 38% and 43%, respectively (P = 0.0048) (). These two treatments also increased stem biomass by 61% and 67%, respectively, compared to the E-High treatment. Pre-fertilization did not affect biomass of medium stocks.
Table 2 Analysis of variance (ANOVA) effect of nursery grading (G), pre-fertilization (F), and their interactions (G × F) on biomass and nitrogen (N) concentration in needles, stems and roots of Changbai larch (Larix olgensis Henry.) at the end of nursery culture and post-transplanting N influx and efflux from 5 to 12 May and from 19 to 26 May 2010
Table 3 Biomass and nitrogen (N) concentration (mean ± standard error) of needles, stems, coarse roots and fine roots of large and medium Larix olgensis Henry. stocks in response to the control, E-Low, E-Medium and E-High pre-fertilization treatments at the end of nursery culture
For large stocks, compared to the control, the E-Medium treatment increased N concentration in needles, stems and roots by 21% (P = 0.0002), 29% (P = 0.0183) and 20% (P = 0.0127), respectively. Medium-sized seedlings were not sensitive to pre-fertilization in N concentration, and only needle N concentration increased by 25% (P = 0.0041) in response to the E-Medium treatment relative to the control.
Results of vector nutrient diagnosis on needles indicated that, compared to the control, the E-High treatment resulted in an excess N concentration and both E-Low and E-medium treatments alleviated the N deficiency at different levels ().
Net N flux characteristics
Both stock size and pre-fertilization influenced N flux in transplanted stocks (). From 5 to 12 May 2010, both net N efflux in distilled water and net N influx in nutrient solution were higher for large stocks than medium stocks in all pre-fertilization treatments (). For large stocks, compared to the results from 5 to 12 May 2010, net N efflux rate tended to rise, and net N influx rate tended to increase in the pre-fertilization treatments of E-Medium and E-High. From 19 to 26 May 2010, both net N efflux and influx rates for large stocks were higher than those for medium stocks in all pre-fertilization treatments ().
Figure 2 Net nitrogen (N) influx rate in nutrient supplied solution (left) and net N efflux rate in distilled water (right) of large and medium Larix olgensis Henry. Stocks subjected to the control and the E-Low, E-Medium and E-High nursery fertilization treatments from 5–12 May and 19–26 May 2010. The asterisk indicates the significant difference of N flux between large and medium stocks.
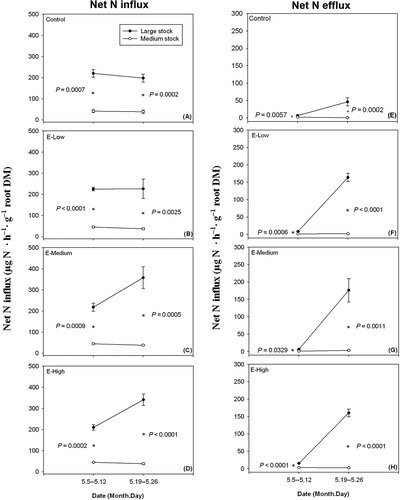
Pre-transplanting root biomass was linearly correlated with both net N influx and net N efflux in post-transplanting stock roots (). Although pre-transplanting root N concentration did not correlate with N flux for both sizes of stocks, correlation for large stocks was significant [f(x) = 2.4118x – 28.4111, n = 4, R = 0.959, P = 0.041].
Table 4 Linear correlations between seedling root nitrogen (N) concentration or root biomass before harvest in October 2009 and net N flux in transplanted stocks of Changbai larch (Larix olgensis Henry.) from 5 to 12 May and from 19 to 26 May 2010 (n = 8)
DISCUSSION
Seedling response to pre-fertilization
We did not determine initial nutrient reserves within overwinter Changbai larch stocks, but vector analysis indicated that our seedlings were pre-fertilized to discriminative nutritional statuses (), which would be subsequently conserved until transplanting because all seedlings were handled in the same experimental condition throughout the study.
Although no fertilizer was added in the control treatment, available N content in nursery soils (556.18 kg available N ha–1) fed controlled seedlings for a whole growing season. On the basis of soil N, pre-fertilization treatments of E-Low and E-Medium at rates of 31.58 and 200 kg N ha–1, respectively, tended to increase needle biomass and N accumulation relative to the control treatment (), therefore these two treatments were indicted to alleviate nutrient deficiency (). The E-High treatment at a high rate of 525.08 kg N ha–1 induced not only a nutrient excess relative to the control treatment (), but also a nutrient toxicity (Salifu and Timmer Citation2003; Salifu and Jacobs Citation2006) relative to other two fertilization treatments, which is probably be due to NH4+ toxicity (Britto and Kronzucker Citation2002). The lack of any significant effect of pre-fertilization treatment on medium stock biomass could be attributed to high seedling density (~550 seedling m–2) (Wei et al. Citation2012).
Though three-stage fertilization has been found to lose more nutrients than exponential nutrient loading for containerized Larix seedlings (Park et al. Citation2012), the “three-time” fertilization may be a proper option for bare-root seedling culture because of the high cost of fertilizer application. The exponential nutrient feeding pattern performed in our study may also be more suitable than traditional ones (Wei et al. Citation2012; Li et al. Citation2013) in late-season nutrient reserves establishment because it followed seedling growth rhythm (Duan et al. Citation2013). However, nutrient reserves within our seedlings may be diluted in fall (Timmer Citation1996) because our fertilization ended in late summer (25 July) prior to seedling hardening. This could be avoided if the last fertilizer application was carried out in fall.
Net N flux characteristics
Our results proved that, during the first month after transplanting, larger stocks had a higher N influx rate in roots in all pre-fertilization treatments (). This could be attributed to the larger root morphology which accelerated nutrient uptake in a certain time, while our net N influx rate was calculated by root biomass. Also, accelerated rise of amino acids in the xylem sap following bud burst (Malaguti et al. Citation2001) and subsequently growing shoots probably draws N to flow into large stock roots. In our study, relative to the medium stock, large stock had a higher bud break ratio (20.36 ± 4.53% and 47.87 ± 5.35%, respectively, F = 17.04, P = 0.0002, determined on the second day after hydroponics began) and greater new shoot biomass (19.53 ± 2.34 mg and 118.57 ± 8.44 mg, respectively, F = 185.06, P < 0.0001, determined on 26 May 2010).
As with the results of N influx, net N efflux was found to be higher for larger stocks in all pre-fertilization treatments (). This concurs with findings for alfalfa (Medicago sativa L.) and soybean (Glycine max L. Merr.) (Brophy and Heichel Citation1989), Zea mays L. (Jones and Darrah Citation1994), clover (Trifolium repens L.) and ryegrass (Lolium perenne L.) (Paynel et al. Citation2001). Larger stocks had a more developed root system, which enlarged the contact between the root surface and water and hence increased the opportunity for root N exudation. As large stock roots grew, net N efflux increased within one month’s time ( E–H). Nambiar and Fife (Citation1991) suggested that N release from tree roots might be largely due to root exudation, which often increases when plants are stressed (Brophy and Heichel Citation1989). Newly transplanted tree seedlings usually suffer the stress of low soil moisture and nutrient availability (Grossnickle Citation2005; Islam and Macdonald Citation2005), which may have induced N efflux to be an evolutionary trait for tree seedlings. In our study, the pots filled with distilled water simulated a humid but infertile condition for transplanted Changbai larch seedlings, therefore insufficient current nutrient supply stimulated amounts of net N efflux, although before the hydroponic experiment N was reserved in all seedlings fed with nutrients in the nursery.
Studies have proven that N efflux usually happens with N influx (Kronzucker et al. Citation1997; Pritchard and Guy Citation2005), and N efflux is often correlated with N influx (Kronzucker et al. Citation1997; Britto and Kronzucker Citation2002; Miller and Hawkins Citation2007), although some N may be reabsorbed even when net N efflux is high (Brophy and Heichel Citation1989). In pre-fertilization treatments of E-Medium and E-High, net N influx rate in large stock roots obviously increased within one month’s time (Fig. and D). Pearson correlation indicated that net N influx increased linearly with net N efflux in roots from seedlings subjected to these two treatments for large stocks (R = 0.992, P < 0.001), but no correlation was detected for medium stocks (R = –0.091, P = 0.775). These results suggest that well pre-fertilized larger stocks would risk more in N release loss in humid but infertile sites although their N influx was higher.
Relationship between end-of-nursery roots and post-transplanting N flux
Larch is one of the typical tree species distributed in infertile wetlands (Girardin et al. Citation2001), although its survival and growth are impacted by low N availability and flood events (Roy et al. Citation2000; Islam and Macdonald Citation2005). Target seedling culture may be a meaningful practice to improve the performance of larch in wetlands, but the issue of root N flux during waterlogging has not drawn enough attention. Our study found that seedling root biomass measured at the end of nursery culture linearly correlated with N influx in roots one month after transplanting (). These findings support the suggestion of forestation using stocks with large roots (Jacobs et al. Citation2005; Li et al. Citation2013); meanwhile, however, our results also put forward a shortcoming of forestation in infertile wetlands or sites that are vulnerable to flood because of the risk of N leaching loss from roots. For large stocks, we also found a linear correlation between pre-transplanting root N concentration and root N influx in the first month after transplanting. Better initial N reserves created by pre-fertilization could result in higher root enzyme activity helping to absorb and assimilate external nutrients. Another possible explanation was that sufficient internal N within roots may induce released N to be mainly NH4+-N (Paynel et al. Citation2001; Miller and Hawkins Citation2007) or amino-N (Brophy and Heichel Citation1989; Jones and Darrah Citation1994) which could be absorbed efficiently by conifers, although we did not determine the compounds of root exudation of Changbai larch stocks.
ACKNOWLEDGMENTS
Funding for this study was provided by the Special funds for scientific research public welfare project of forestry (201004021), “948” International Cooperation Project (2011-4-58), National forestry technology achievements development project of 2011 (2011-44), “11th Five-Year Plant” National Key Technology and R&D Program (Grant No. 2006BAD24B01) from Chinese State Forestry Administration, and Program of Supporting PhD Candidates in Beijing Forestry University for Visiting Internationally Famous Universities Abroad of China. Editors and reviewers are appreciated for their corresponding works for submission. We thank Dr. Barbara Hawkins for her insightful comments on an earlier manuscript edition, which improved the quality of this paper.
REFERENCES
- Britto DT, Kronzucker HJ 2002: NH4+ toxicity in higher plants: A critical review. J. Plant. Physiol., 159, 567–584.
- Brophy LS, Heichel GH 1989: Nitrogen release from roots of alfalfa and soybean grown in sand culture. Plant. Soil, 116, 77–84.
- Cuesta B, Vega J, Villar-Salvador P, Rey-Benayas JM 2010a: Root growth dynamics of Aleppo pine (Pinus halepensis Mill.) seedlings in relation to shoot elongation, plant size and tissue nitrogen concentration. Trees, 24, 899–908.
- Cuesta B, Villar-Salvador P, Puértolas J, Jacobs DF, Rey Benayas JM 2010b: Why do large, nitrogen rich seedlings better resist stressful transplanting conditions? A physiological analysis in two functionally contrasting Mediterranean forest species. For. Ecol. Manage., 260, 71–78.
- Duan J, Chengyang X, Jacobs DF, Ma LY, Wei HX, Jiang LN, Ren J 2013: Exponential nutrient loading shortens the cultural period of Larix olgensis seedlings. Scand. J. For. Res., 28, 409–418. doi: org/10.1080/02827581.2013.778328
- Fløistad IS, Kohmann K 2004: Influence of nutrient supply on spring frost hardiness and time of bud break in Norway spruce (Picea abies (L.) Karst.) seedlings. New. For., 27, 1–11.
- Girardin M-P, Tardif J, Bergeron Y 2001: Gradient analysis of Larix laricina dominated wetlands in Canada’s southeastern boreal forest. Can. J. Bot., 79, 444–456.
- Grossnickle SC 2005: Importance of root growth in overcoming planting stress. New. For., 30, 273–294.
- Islam MA, Macdonald SE 2005: Effects of variable nitrogen fertilization on growth, gas exchange, and biomass partitioning in black spruce and tamarack seedlings. Can. J. Bot., 83, 1574–1580.
- Jacobs DF, Salifu KF, Seifert JR 2005: Relative contribution of initial root and shoot morphology in predicting field performanceof hardwood seedlings. New. For., 30, 235–251.
- Jobidon R, Roy V, Cyr G 2003: Net effect of competing vegetation on selected environmental conditions and performance of four spruce seedling stock sizes after eight years in Quebec (Canada). Ann. For. Sci., 60, 691–699.
- Jones DL, Darrah PR 1994: Amino-acid influx at the soil-root interface of Zea mays L. and its implications in the rhizosphere. Plant Soil, 163, 1–12.
- Kronzucker HJ, Siddiqi MY, Glass ADM 1997: Conifer root discrimination against soil nitrate and the ecology of forest succession. Nature, 385, 59–61.
- Li GL, Liu Y, Zhu Y, Yang J, Sun HY, Jia ZK, Ma LY 2011: Influence of initial age and size on the field performance of Larix olgensis seedlings. New. For., 42, 215–216.
- Li GL, Zhu Y, Liu Y, Jiang L, Shi HW, Liu JJ, Wang JX, Cheng ZQ 2013: Effect of nursery nitrogen application of bare-root Larix olgensis seedlings on growth, nitrogen uptake and initial field performance. J. Environ. Bio., 34, 79–85.
- Malaguti D, Millard P, Wendler R, Hepburn A, Tagliavini M 2001: Translocation of amino acids in the xylem of apple (Malus domestica Borkh.) trees in spring as a consequence of both N remobilization and root uptake. J. Exp. Bot., 52, 1665–1671.
- Miller BD, Hawkins BJ 2007: Ammonium and nitrate uptake, nitrogen productivity and biomass allocation in interior spruce families with contrasting growth rates and mineral nutrient preconditioning. Tree Physiol., 27, 901–909.
- Nambiar EKS, Fife DN 1991: Nutrient retranslocation in temperate conifers. Tree Physiol., 9, 185–207.
- Oliet JA, Planelles R, Artero F, Valverde R, Jacobs DF, Segura ML 2009: Field performance of Pinus halepensis planted in Mediterranean arid conditions: Relative influence of seedling morphology and mineral nutrition. New. For., 37, 313–331.
- Park B, Cho MS, Lee SW, Yanai RD, Lee DK: 2012. Minimizing nutrient leaching and improving nutrient use efficiency of Liriodendron tulipifera and Larix leptolepis in a container nursery system. New. For., 43, 57–68.
- Paynel F, Murray PJ, Cliquet JB 2001: Root exudates: A pathway for short-term N transfer from clover and ryegrass. Plant Soil, 229, 235–243.
- Pinto JR, Marshall JD, Dumroese RK, Davis AS, Cobos DR 2011: Establishment and growth of container seedlings for reforestation: A function of stocktype and edaphic conditions. For. Ecol. Manage., 261, 1876–1884.
- Pritchard ES, Guy RD 2005: Nitrogen isotope discrimination in white spruce fed with low concentrations of ammonium and nitrate. Trees, 19, 89–98.
- Roberts JJ, Zwiazek JJ 2001: Growth, morphology, and gas exchange in white spruce (Picea glauca) seedlings acclimated to different humidity conditions. Can. J. For. Res., 31, 1038–1045.
- Roy V, Ruel J-C, Plamondon AP 2000: Establishment, growth and survival of natural regeneration after clearcutting and drainage on forested wetlands. For. Ecol. Manage., 129, 253–267.
- Salifu KF, Jacobs DF 2006: Characterizing fertility targets and multi-element interactions in nursery culture of Quercus rubra seedlings. Ann. For. Sci., 63, 231–237.
- Salifu KF, Timmer VR 2003: Nitrogen retranslocation response of young Picea mariana to nitrogen-15 supply. Soil. Sci. Soc. Am. J., 67, 309–318.
- Shepherd T, Davies HV 1994: Patterns of short-term amino acid accumulation and loss in the root-zone of liquid-cultured forage rape (Brassica napus L.). Plant Soil, 158, 99–109.
- Timmer VR 1996: Exponential nutrient loading: a new fertilization technique to improve seedling performance on competitive sites. New. For., 13, 275–295.
- van den Driessche R 1985: Late-season fertilization, mineral nutrient reserves, and retranslocation in planted Douglas-fir (Pseudotsuga menziesii (Mirb.) Franco) seedlings. For. Sci., 31, 485–496.
- Wei HX, Xu CY, Hawkins BJ, Ma LY, Jiang LN 2012: Organic amendment and inorganic fertilization affect soil properties and quality of Lairx olgensis bareroot stock. New. For., 43, 155–168.
- Yanai RD, McFarlane KJ, Lucash MS, Kulpa SE, Wood DM 2009: Similarity of nutrient uptake and root dimensions of Engelmann spruce and subalpine fir at two contrasting sites in Colorado. For. Ecol. Manage., 258, 2233–2241.
- Yang LX, Wang P, Kong CH 2010: Effect of larch (Larix gmelini Rupr.) root exudates on Manchurian walnut (Juglans mandshurica Maxim.) growth and soil juglone in a mixed-species plantation. Plant Soil, 329, 249–258.
- Zhu JJ, Liu ZG, Wang HX, Yan QL, Fang HY, Hu L, Yu LZ 2008: Effects of site preparation on emergence and early establishment of Larix olgensis in montane regions of northeastern China. New. For., 36, 247–260.