Abstract
A short-term study was conducted to investigate the greenhouse gas emissions in five typical soils under two crop residue management practices: raw rice straw (Oryza sativa L., cv) and its derived biochar application. Rice straw and its derived biochar (two biochars, produced at 350 and 500°C and referred to as BC350 and BC500, respectively) were incubated with the soils at a 5% (weight/weight) rate and under 70% water holding capacity for 28 d. Incorporation of BC500 into soils reduced carbon dioxide (CO2) and nitrous oxide (N2O) emission in all five soils by 4−40% and 62−98%, respectively, compared to the untreated soils, whereas methane (CH4) emission was elevated by up to about 2 times. Contrary to the biochars, direct return of the straw to soil reduced CH4 emission by 22−69%, whereas CO2 increased by 4 to 34 times. For N2O emission, return of rice straw to soil reduced it by over 80% in two soils, while it increased by up to 14 times in other three soils. When all three greenhouse gases were normalized on the CO2 basis, the global warming potential in all treatments followed the order of straw > BC350 > control > BC500 in all five soils. The results indicated that turning rice straw into biochar followed by its incorporation into soil was an effective measure for reducing soil greenhouse gas emission, and the effectiveness increased with increasing biochar production temperature, whereas direct return of straw to soil enhanced soil greenhouse gas emissions.
INTRODUCTION
In the past decades, China has produced about 630 Mt of crop straws annually (H. Liu et al. Citation2008). The common management of these crop residues is in situ burning in the field, which is considered as a convenient and economical method for farmers to deal with them (Lu et al. Citation2010). However, the burning of crop residues reduces the possibility of carbon (C) sequestration in the soil and meanwhile enhances the release of some greenhouse gases [GHGs, e.g., carbon dioxide (CO2)] (Pathak et al. Citation2006; Yan et al. Citation2006).
One of the effective ways to resolve the problem of burning is direct return of straw to soil, which can increase the organic C input and thereby probably sequester C in the soil (Y. Singh et al. Citation2004). However, most straw C is labile and readily mineralized to CO2 in a few years (Lehmann et al. Citation2006). Straw returned to soil also tends to stimulate methane (CH4) emission (Zou et al. Citation2005), which would offset part of the C sequestration quantity. Turning straw into biochar under low temperature (< 700°C) and limited oxygen conditions, followed by its return to soil, has recently proven a promising approach for long-term C sequestration in soil (Lehmann and Joseph Citation2009). Biochar usually possesses strong aromatic-C structure and it is more biologically and chemically recalcitrant than many other forms of organic matter in soil (Keiluweit et al. Citation2010; B.P. Singh et al. Citation2010). Such a great recalcitrance makes the biochar undergo a much slower decomposition with turnover rate of hundreds to thousands of years (Lehmann et al. Citation2006; Fowles Citation2007). Moreover, some studies showed that biochar can also reduce soil CH4 and/or nitrous oxide (N2O) emissions (Yanai et al. Citation2007; Castaldi et al. Citation2011), although this was not true in all situations (Kim et al. Citation2011; Knoblauch et al. Citation2011). The variability of the observations could be related to different biochar and soil properties (Shneour Citation1966; Spokas and Reicosky Citation2009) and the relation needs to be further investigated.
Hence, in this study, we investigated GHG emissions in five typical soils in China under two crop residue management practices: raw rice straw (Oryza sativa L., cv.) and its derived biochar application. The five soils were collected from different locations in China varying in climate and soil fertility conditions. A 28-d incubation time was selected since a significant change in soil GHGs emissions from organic amendments often occurs within a short term (Yanai et al. Citation2007; Novak et al. Citation2010; Jones et al. Citation2011; Cheng et al. Citation2012; Nelissen et al. Citation2012). The specific objectives of this study are (1) to quantify GHG emission characteristics related to different treatments in the five soils, (2) to elucidate effects of different amendments and soil properties on the GHG emissions and (3) to prove biochar returned to soil is a better crop residue management practice than straw returned to soil for net GHG mitigation potential.
MATERIALS AND METHODS
Rice straw, biochar, and soils
Rice straw was collected from Changshu Agro-ecological Experimental Station, Chinese Academy of Science, China. The biochar was obtained from the rice straw using a typical slow pyrolysis process (Cao and Harris Citation2010). Briefly, the straw was air-dried, ground to less than 2 mm and put into a lab-scale stainless steel pyrolysis reactor. On the reactor top, there were two small vents (Ф1 cm) equipped with a loose lid which allowed the release of gases during the heating treatment and prevented the inflow of air during the cooling period. After the air in the reactor was replaced completely using pure molecular nitrogen (N2), it was heated in a muffle furnace (SX2-12-10, China) at a speed of approximately 20°C min–1 and held at 350 or 500°C for 4 h. The solid residue in the reactor was C-rich and designated as biochar (Cao and Harris Citation2010). The biochars produced at 350 and 500°C were referred to as BC350 and BC500, respectively. The biochar was then ground to pass through a 1.0-mm sieve for future characterization and amendment. Selected physical and chemical properties of the straw and biochars are given in .
Table 1 Physico-chemical properties of rice (Oryza sativa L., cv.) straw, biochars (BC350 and BC500), and five typical soils (YT, AS, SY, CS, and FQ).
Five typical soil samples were collected from eastern, western, southern, northern, and central parts of China, respectively, based on climate and soil fertility conditions, i.e., Changshu (31°33′ N, 120°42′ E) in Jiangsu Province, Ansai (36°51′ N, 109°19′ E) in Shaanxi Province, Yingtan (28°12′ N, 117°0′ E) in Jiangxi Province, Songyuan (45°11′ N, 124°49′ E) in Jilin Province, and Fengqiu (34°35′ N, 115°34′ E) in Henan Province. For simplicity, the five soil samples were accordingly abbreviated as CS, AS, YT, SY and FQ, respectively. The five soils were classified as Gleyi-Stagnic Anthrosols, Loessi-Orthic Primosols, Argi-Udic Ferrosols, Hapli-Udic Isohumosols and Ochri-Aquic Cambosols, respectively, based on pedogenesis and soil taxonomy according to Z.T. Gong et al. (Citation2006), and Typical Endoaquepts, Ustochnept, Plinthudults, Pachic Udic Haploborolls and Aquic Ustochrepts, respectively, according to USDA Soil Survey Staff (1994). All soil samples were collected from the cultivated layers (0–20 cm), air-dried, crushed, and passed through a 2-mm sieve. Soil properties are given in .
Soil treatment and gas sampling
The four treatments were established with three replicates: Soil, Soil + Straw, Soil + BC350 and Soil + BC500. Specifically, 50 g of dried soil was first placed in a polyvinyl chloride (PVC) plastics column container (60 mm height, 50 mm diameter) and distilled water was added to achieve a moisture content of 40% water holding capacity (WHC). The soil was then incubated at 25 ± 1°C in the dark for 7 d to stabilize microbial activity (J.Y. Wang et al. Citation2011). After 7 d of pre-incubation, the soil was completely mixed with straw, BC350 or BC500 at a ratio of 5% amendment [weight/weight (w/w), dry basis] and water content in all amendments was then kept to 70% WHC. The incubation lasted for 28 d.
The CO2, CH4, and N2O gases were collected at 1, 2, 3, 5, 7, 14 and 28 d using the closed chamber method (Yanai et al. Citation2007). A clean column-PVC lid (150 mm height and 70 mm diameter, 577 mL) was used as a gas-tight chamber. Initially, approximately 5 mL of gas was drawn from the headspace of the chamber using a gas-tight syringe as the initial value. After incubation with closed chamber lid for 2 h, the gas in the chamber headspace was collected as the final value. The real GHGs content change was obtained from the difference between the final value and the initial value. After gas sampling, the soil incubation containers were flushed with ambient air and kept open until the next sampling.
Analytical methods
Specific surface area and pore size distribution of biochars were determined using a BET-N2 SA analyzer (JW-BK222, Jwgb, China). The pH of soil and biochar was measured using pH detector (EUTECH pH 510, USA) with a solid to water ratio of 1: 2.5 [weight/volume (w/v)] for soil and 1:15 (w/v) for biochar. The ammonium nitrogen (NH4+-N) and nitrate nitrogen (NO3–-N) in the biochar were extracted with distilled water (solid to water ratio of 1:200, w/v) for 16 h in a shaker at room temperature, and those in the soil were extracted with 2 mol L–1 potassium chloride (KCl) solution (solid to water ratio of 1:10 w/v) for 1 h in a shaker at room temperature. The suspensions were then filtered through a 0.45-μm membrane filter for determination of NH4+-N and NO3–-N using an ultraviolet spectrophotometer, and dissolved organic carbon (DOC) was measured using a multi N/C 2100 TOC (Germany) analyzer (W. Gong et al. Citation2009). The concentrations of C and nitrogen (N) in soil and biochar were determined using an elemental analyzer (Vario EL III, Elementar, Germany). Soil texture was measured with a laser particle characterization analyzer (Berkman Coulter, Los Angeles, USA).
The concentrations of CO2, CH4, and N2O were simultaneously detected by a gas chromatograph (Agilent 7890A, USA) equipped with two detectors: a hydrogen flame ionization detector applied to detect CO2 and CH4 and an electron capture detector applied to detect N2O. More details on conditions and parameters of the gas chromatograph can be found in J.Y. Wang et al. (Citation2011).
Calculation of greenhouse gas emission andglobal warming potential
The emission rates of CO2, CH4 and N2O were calculated using the following equation:
where F is emission rate (mg CO2-C kg–1 h–1, μg CH4-C kg–1 h–1, and μg N2O-N kg–1 h–1), ρ is the density of CO2-C, CH4-C or N2O-N under standard state (CO2-C and CH4-C normalized, 0.536 kg m–3; N2O-N 1.25 kg m–3); dc/dt is the change in concentrations of the gases per unit time (mg kg–1 h–1 or μg kg–1 h–1), V is the headspace of the apparatus (m3), T is incubation temperature (°C), and W is weight of soil (kg).
The cumulative emission amounts of the three gases in the 28-d incubation were calculated based on the following equation:
where S is the CO2, CH4 or N2O cumulative emission(mg CO2-C kg–1, μg CH4-C kg–1 or μg N2O-N kg–1), Fi is described above in equation (1), ti is incubation days when gas sample is collected.
The global warming potential (GWP) value was calculated from all three GHGs in mg CO2 equivalent per kilogram soil (IPCC Citation2007). The default GWP of CH4 and N2O in a 100-yr time frame were regarded as 25 and 298 respectively, while the GWP value for CO2 was taken as 1 (IPCC Citation2007).
Statistical analysis
Difference between the treatment and soil type was analyzed with two-way analysis of variance (ANOVA). Difference among the treatments was analyzed with one-way ANOVA. All tests of significance (P < 0.05) were conducted with Tukey-Kramer highly significant difference (HSD) test. Statistical analyses were performed using SPSS 17.0 (SPSS software for Windows 7, Version 17.0, 2010).
RESULTS
Characteristics of straw, biochar, and soils
Selected properties of rice straw, straw-derived biochar, and soils are shown in . Both BC350 and BC500 were alkaline, and BC500 pH was about 1 unit higher compared to BC350. By turning rice straw into biochar, the C concentration was increased by 40% for BC350 and 51% for BC500. The C concentration of BC500 was higher than that of BC350, while DOC of BC500 was much lower than that of BC350. The surface area and pore volume of BC500 were much higher than that of BC350, but conversely for the average pore diameter.
Properties of the five soils varied greatly (). Soil pH ranged from acid to alkaline (5.05–8.04), but they were much lower than those of the biochars. The CS and FQ soils contained higher C, N and DOC compared with the YT, AS and SY soils. For particle distribution, the CS and FQ soils had higher silt content (> 71%) than the other three (YT, AS and SY) soils (< 55%).
GHGs emissions from soil influenced by straw or biochar addition
The emission dynamics of CO2, CH4, and N2O gases are shown in –. All three gases emissions showed a similar tendency among the five soils; i.e., the greatest change happened in the first 7 d and remained stable in the latter 21 d. Nevertheless, extent of the effects of different amendments and soil types on the three gases were variable.
Figure 1 Dynamics of soil carbon dioxide (CO2) emission from five soils (a, b, c, d and e stand for soil from YT, Yingtan; AS, Ansai; SY, Songyuan; CS, Changshu; and FQ, Fengqiu respectively) with different treatments and 28 d incubation. Soil, Soil + Straw, Soil + BC350, and Soil + BC500 stand for soil only, soil + 5% rice (Oryza sativa L., cv.) straw, soil + 5% straw biochar produced at 350°C, and soil + 5% straw biochar produced at 500°C, respectively. C, carbon.
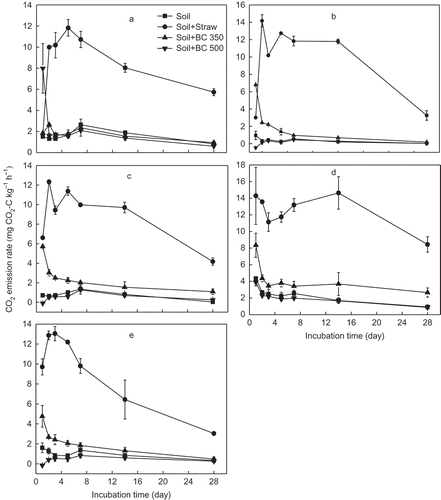
Figure 2 Dynamics of soil methane (CH4) emission from five soils (a, b, c, d and e stand for soil from YT, Yingtan; AS, Ansai; SY, Songyuan; CS, Changshu; and FQ, Fengqiu respectively) with different treatments and 28 d incubation. Soil, Soil + Straw, Soil + BC350, and Soil + BC500 stand for soil only, soil + 5% rice (Oryza sativa L., cv.) straw, soil + 5% straw biochar produced at 350°C, and soil + 5% straw biochar produced at 500°C, respectively. C, carbon.
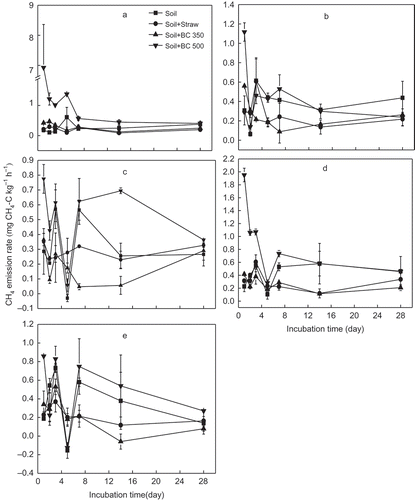
Figure 3 Dynamics of soil nitrous oxide (N2O) emission from five soils (a, b, c, d and e stand for soil from YT, Yingtan; AS, Ansai; SY, Songyuan; CS, Changshu; and FQ, Fengqiu respectively) with different treatments and 28 d incubation. Soil, Soil + Straw, Soil + BC350, and Soil + BC500 stand for soil only, soil + 5% rice (Oryza sativa L., cv.) straw, soil + 5% straw biochar produced at 350°C, and soil + 5% straw biochar produced at 500°C, respectively. N, nitrogen.
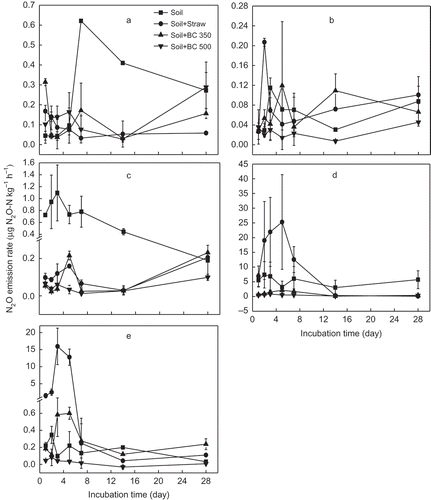
CO2 emission reached a peak at 7 d and then decreased steadily in most soils, except in the CS soil that had the highest emission at 14 d (). The cumulative CO2 emission during the incubation period significantly (p < 0.05) changed from 141 ± 26 to 8298 ± 565 mg C kg–1 with different treatments and different soils (). Compared to the control soil, straw significantly increased the CO2 emissions (p < 0.05) in all five soils by about 4 to 34 times and BC350 elevated the CO2 emissions (p < 0.05) from 78 to 289% except for the YT soil. However, BC500 suppressed the CO2 emission from 4 to 40% except for the YT soil. Overall, the CO2 cumulative emission with rice straw addition was higher than that with biochar addition, following the order of Soil + Straw > Soil + BC350 > Soil > Soil + BC500.
Figure 4 Cumulative emissions of (A) carbon dioxide (CO2), (B) methane (CH4), and (C) nitrous oxide (N2O) from five soils (YT, Yingtan; AS, Ansai; SY, Songyuan; CS, Changshu; and FQ, Fengqiu) with different treatments and 28 d incubation. Soil, Soil + Straw, Soil + BC350, and Soil + BC500 stand for soil only, soil + 5% rice (Oryza sativa L., cv.) straw, soil + 5% straw biochar produced at 350°C, and soil + 5% straw biochar produced at 500°C, respectively. C, carbon; N, nitrogen.
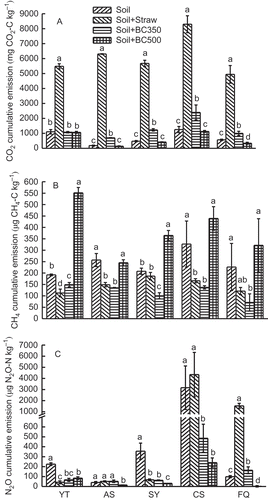
The CH4 release dynamic was not as clear as that of CO2 for all amendments, with great variation in the first week (). The CH4 emission increased with the addition of BC500 while straw and BC350 reduced CH4 emission over the incubation period, which was contrary to the CO2 emission (). The cumulative CH4 emission during the 28-d incubation in the BC350 and straw amendment soils was reduced by 22–69% and 10–49%, respectively, compared to the control soil, while BC500 returned to soils elevated the CH4 emission by up to about 2 times ().
Like CH4, the N2O release dynamic had a great variation in the first week (). Straw returned to YT and SY soils significantly (p < 0.05) suppressed N2O emissions by 80% and 81%, respectively, while the emissions increased by up to 14 times in the other three soils (Fig. ). BC350 addition to YT, SY, and CS soils inhibited the N2O emissions by up to 85%, while the N2O emissions increased in AS and FQ soils by 30 and 66%, respectively. However, BC500 addition to all five soils significantly (p < 0.05) reduced N2O emissions by 62–98%.
Contribution of straw or biochar addition to GWP
As shown above, the effects of straw and biochar on the GHGs emissions were highly variable with the amendments, gas types and soil types. However, when all three greenhouse gases were normalized on a CO2 basis, the overall GWP from the five soils followed the order of CS > YT > SY ≈ FQ > AS (). Compared to the control soil, straw addition significantly (p < 0.05) increased GWP by about 4 to 31 times, while BC500 addition to all five soils consistently decreased GWP by up to 45%, although BC350 elevated GWP by up to 2.6 times which was still much lower than that of returned straw ().
Table 2 The pH, ammonium nitrogen (NH4+-N), nitrate nitrogen (NO3–-N), and dissolved organic carbon (DOC) in the different treatments with 28 d incubation
Table 3 Total carbon dioxide (CO2) equivalent emission [global warming potential (GWP), mg CO2 kg–1 soil] following different treatments with 28 d incubation
Effect of different treatments on soils properties after incubation
In order to obtain the relationship of the GHG emissions between soils and amendments, selected physico-chemical properties of control soils and amended soils including pH, NH4+-N, NO3–-N, and DOC were assessed after the 28-d incubation. BC500 addition increased soil pH, while both straw and BC350 addition reduced soil pH, except for CS soil, compared to control soil (). Cumulative CH4 and CO2 emission rates of all soils except CS soil were significantly (p < 0.05) correlated with pH change. Moreover, there was also a significantly (p < 0.01) negative correlation between N2O cumulative emission rate and pH for AS and FQ soils ().
Table 4 The correlation coefficients between cumulative emission of greenhouse gases (GHGs) and characteristics of five type soils following different treatments after 28 d incubation.
The DOC concentrations in the treatments of the five soils changed smoothly after incubation (). Briefly, straw returned to soils was significantly greater in DOC change than the other treatments. Compared to the control soil, BC350 and BC500 additions reduced the DOC concentrations for YT, AS and SY soils, and the opposite results were found in the other two soils. Cumulative CO2 emission rates of the five soils were significantly (p < 0.01) positively correlated with DOC change. Moreover, for FQ soil, there was significantly (p < 0.05) negative correlation between DOC change and CH4 emission rate, while significantly (p < 0.01) positive correlation existed between that and N2O cumulative emission rate ().
The effects of different treatments in five soils on inorganic N (NH4+-N and NO3–-N) content changed differently (). Briefly, no significant (p > 0.05) differences of NH4+-N content were found in different treatments for SY and FQ soils. Biochar addition to soil significantly (p < 0.05) increased NH4+-N content for YT soil. There were no significant (p > 0.05) differences in NO3–-N content among all treatments of AS and SY soils; however, significant (p < 0.05) differences between treatments were found in YT soil from 0.26mg kg–1 to 41.4 mg kg–1. Compared to the control soil, straw, BC350 and BC500 additions reduced the NO3–-N content in CS and FQ soils (). The cumulative N2O emission rate of the FQ soil was significantly (p < 0.01) correlated with NO3-N change ().
DISCUSSTION
Elevated CO2 emissions were observed from the soils with raw straw and BC350 return, which was probably due to the fact that straw and BC350 were rich in DOC and thus more readily degradable via microbial activity (Lehmann et al. Citation2006). All the treated soils with straw addition showed higher initial CO2 emissions, probably resulting from the rapid mineralization of the fresh straw biomass (Smith et al. Citation2010). The highest emission in the CS soil was probably due to its highest DOC content (). Contrary to the straw and BC350 treatments, BC500 addition inhibited CO2 emission in all five soils, though the emission did not reach a significant (p < 0.05) level except for in the FQ soil, and emission rate remained stable during the incubation period (). BC500 had a strong alkalinity with pH > 10 (). After the BC500 was returned to soil, the soil pH increased (), which may allow the produced CO2 to be trapped in the soil solution as carbonates, resulting in a reduction of CO2 emissions. Our observations agreed with previous work showing that application of biochar to soil can inhibit soil CO2 release (Spokas et al. Citation2009; Y.X. Liu et al. Citation2011; J.Y. Wang et al. Citation2011). Compared to the other four soils, both BC350 and BC500 had less effect on CO2 emissions from the YT soil (), probably due to its acidity (). A liming experiment also showed that acidic soils were less favorable for CO2 production (Mørkved et al. Citation2007). Less CO2 emission in the biochar-treated soil may be also a result of the condensed aromatic nature of biochar that is difficult for microorganisms to degrade/mineralize and is therefore more stable in soil than non-charred straw (Lehmann et al. Citation2006). Bruun et al. (Citation2012) indicated that only 3–12% of added biochar-C had been emitted as CO2 within 2 months of soil incubation, while the straw feedstock lost more than 50% of its initial C content. Knoblauch et al. (Citation2011) showed that only 4–9% of rice husk biochar was mineralized to CO2 within 3 yr of soil incubation, compared to 31–100% of the rice husk mineralized to CO2. The present study indicated that the C loss as CO2 in both BC350 and BC500 biochars only accounts for less than 4% of the initial C content within 28 d of soil incubation, while the raw straw lost 23–37% of its initial C content as CO2 emission (). Moreover, BC500 derived at higher pyrolysis temperature had lower DOC than BC350 (), resulting in less CO2 emission than BC350 (). Similar results for the biochar temperature effect were obtained in other works. For example, Pereira et al. (Citation2011) found that low-temperature (400°C) biochar induced a relatively higher CO2 efflux than did high-temperature (500°C) biochar.
Note that what was detected in our experiment was the net result of CH4 oxidation and CH4 production. Wide variations in CH4 emission from soils with biochar amendment have been reported in the literature (Kim et al. Citation2011). Some studies indicated that biochar incorporation into soil reduced CH4 emission since biochar could improve soil aeration and decease the anaerobic condition in soils, which could decrease CH4 production (inhibition methanogens activity), and/or biochar could increase CH4 oxidation (promoting methanotroph activity) or enhance the adsorption of CH4 in soil based on the high porosity and the large surface area (Lehmann et al. Citation2011; Scheer et al. Citation2011). However, other studies argued that incorporation of biochar into soil could increase CH4 emissions, probably due to the improved soil water holding capability, high DOC content, pH and metals which may potentially inhibit CH4 oxidation and/or promote CH4 production (Zhang et al. Citation2010; Knoblauch et al. Citation2011). In this study, there was significant positive correlation between CH4 emission and pH for all five soils (). Return of straw and BC350 reduced soil pH (), resulting in decline of CH4 emission, compared to the control soil (). However, the BC 500 had high pH (), which increased soil alkalinity (), especially in the acidic YT and CS soils, enhancing CH4 production (Mer and Roger Citation2001). For the FQ soil, there was significant negative correlation between the CH4 emission and DOC (), the same conclusion as reported by Ström et al. (Citation2003). In our experiment, 70% WHC and such a short-term incubation (28 d) was probably not the best condition for CH4 production, but it may have improved CH4 oxidation. The net result showed that the DOC was mineralized in terms of CO2. As a result, the negative correlation between CH4 production and DOC was observed for the FQ soil.
The cumulative N2O emissions from CS and FQ soils were higher than those of the other three soils (), probably due to the high NO3–-N and DOC in these two soils (), which may favor soil denitrification, generating more N2O. Impressively, BC500 addition to all five soils significantly (p < 0.05) reduced N2O emissions by 62–98%, which supported the idea that biochar returned to soil was a great effective measure for straw management to mitigate N2O emissions from cropland (B.P. Singh et al. Citation2010; Bruun et al. Citation2011). Soil pH, DOC, and NO3–N contents were key factors influencing N2O emission in the biochar-amended soils (Weier and Gilliam Citation1986; Granli and Bøckman Citation1995). Application of biochar, especially BC500, into soil significantly reduced N2O emission, in accordance with other reports (B.P. Singh et al. Citation2010; Castaldi et al. Citation2011). It can probably be attributed to increase of soil pH induced by biochar (), which promoted N2O convert to N2 (Clough et al. Citation2010). In addition, biochar had high surface area and pore volume () which might adsorb N2O in soil air and water (Bagreev et al. Citation2001), and metal oxides on or near biochar surfaces may catalyze the reduction of N2O to N2 (Lehmann and Joseph Citation2009). BC350 returned to YT, SY and CS soils would inhibit N2O emissions, while the increase in AS and FQ soil can probably be attributed to pH, DOC or NO3–-N change (). For example, there was a significant negative correlation between N2O emission and pH for AS and FQ soils (). Moreover, there was a significant negative correlation between N2O emission and NO3–-N for FQ soil () probably due to high NO3–-N content that could inhibit N2O production and/or stimulate N2O reduction (Ellis et al. Citation1996; Luo et al. Citation1996). In addition, the N2O production gene and N2O consuming gene change was probably one potential cause (C. Wang et al. Citation2013).
The biochar type and application rate can also affect the GHGs emissions. Spokas et al. (Citation2009) reported sawdust biochar amendment reduced CO2 and N2O production and inhibited ambient CH4 oxidation for all application rates from 2% to 60%. O’Toole et al. (Citation2013) observed wheat (Triticum sp.) straw biochar application to soil at 5% and 10% increased CO2 emission, while the emission decreased at 1%, compared to the control soil. Van Zwieten et al. (Citation2010) indicated a biosolid biochar application rate of 5% caused less CO2 and N2O emission than control soil. Our work showed a similar result, i.e., CO2 and N2O emissions were reduced at a 5% rate of rice straw biochar application.
As discussed above, effects of straw and biochar on GHGs emissions were highly variable with the amendments, gas types and soil types. However, when all three greenhouse gases were normalized on a CO2 basis, the overall GWP from the five soils followed the order of CS > YT > SY ≈ FQ > AS, and that for all treatments decreased as straw > BC350 > BC500 ().
CONCLUSIONS
The present study demonstrated the different GHG emission characteristics with two crop residue management practices: raw rice straw and its derived biochar application. The amendment of BC500, which was produced at 500°C, a relatively higher temperature, significantly reduced soil CO2 and N2O emissions in all five soils, though it increased CH4 emission. However, the GWP of soil with BC500 application was much lower than that of straw and even lower than that of BC350 which was produced at 350°C, a relatively low temperature. The GWP for all treatments followed the order of straw > BC350 > BC500, and that from the five soils decreased as CS > YT > SY ≈ FQ > AS. Comparing the two crop residue management practices, converting straw into biochar followed by its application to soil showed an effective method for global warming mitigation, and the effectiveness increased with biochar production temperature. It should be pointed out that the effects observed in this study were only obtained in the laboratory experiments with one straw and its biochar amendment during a short time of 28 d incubation. The long-term effects and field-scale trials with other straws and their derived biochar amendments need to be assessed.
ACKNOWLEDGMENTS
This work was supported in part by the National Natural Science Foundation of China (No. 21077072, 21107070), the Shanghai Pujiang Talent Project (11PJ1404600), The University Innovation Project (AE1600004), and the Colleges and Universities Excellent Young Talents Fund Project of Anhui Province (No. 2012SQRL149).
REFERENCES
- Bagreev A, Bashkova S, Locke DC, Bandosz TJ 2001: Sewage sludge derived materials as efficient adsorbent for removal of hydrogen sulfide. Environ. Sci. Technol., 35, 1537–1543.
- Bruun EW, Ambus P, Egsgaard H, Nielsen HH 2012: Effects of slow and fast pyrolysis biochar on soil C and N turnover dynamics. Soil Biol. Biochem., 46, 73–79.
- Bruun EW, Stöver DM, Ambus P, Nielsen HH 2011: Application of biochar to soil and N2O emissions: Potential effects of blending fast-pyrolysis biochar with anaerobically digested slurry. Eur. J. Soil Sci., 62, 581–589.
- Cao XD, Harris W 2010: Properties of dairy-manure-derived biochar pertinent to its potential use in remediation. Bioresour. Technol., 101, 5222–5228.
- Castaldi S, Riondino M, Baronti S, Espostio FR, Marzaioli R, Vaccari F 2011: Impact of biochar application to a Mediterranean wheat crop on soil microbial activity and greenhouse gas fluxes. Chemosphere, 85, 1464–1471.
- Cheng Y, Cai ZC, Chang S C, Wang J, Zhang JB 2012: Wheat straw and its biochar have contrasting effects on inorganic N retention and N2O production in a cultivated black chernozem. Biol. Fertil. Soils, 48, 941–946.
- Clough TJ, Bertram JE, Ray JL, Condron LM, O’Callaghan M, Sherlock RR, Wells NS 2010: Unweathered wood biochar impact on nitrous oxide emissions from a bovine-urine-amended pasture soil. Soil Sci. Soc. Am. J., 74, 852–860.
- Ellis S, Dendooven L, Goulding KWT 1996: Quantitative assessment of soil nitrate disappearance and N2O evolution during denitrification: Nitrate disappearance during denitrification. Soil Biol. Biochem., 28, 589–595.
- Fowles M 2007: Black carbon sequestration as an alternative to bioenergy. Biomass Bioenerg., 31, 426–432.
- Gong W, Yan XY, Wang JY, Hu TX, Gong YB 2009: Long-term manuring and fertilization effect on soil organic carbon pools under a wheat-maize cropping system in north China plain. Plant Soil, 149, 318–324.
- Gong ZT, Zhang GL, Chen ZC 2006: Pedogenesis and soil taxonomy, China Science Press, Beijing, China, pp. 480–487 ( in Chinese).
- Granli T, Bøckman OC 1995: Nitrous oxide (N2O) emissions from soils in warm climates. Nutr. Cycl. Agroecosyst., 42, 159–163.
- IPCC (Intergovernmental Panel on Climate Change) 2007: Agriculture. In Climate change 2007: Mitigation, contribution of working group III to the fourth assessment report of the Intergovernmental Panel on Climate Change, Eds. Metz B, Davison OR, Bosch PR, pp. 498–540. Cambridge University Press, Cambridge, UK.
- Jones DL, Murphy DV, Khalid M, Ahmad W, Edwards-Jones G, Deluca TH 2011: Short-term biochar-induced increase in soil CO2 release is both biotically and abiotically mediated. Soil Biol. Biochem., 43, 1723–1731.
- Keiluweit M, Nico PS, Johnson MG, Kleber M 2010: Dynamic molecular structure of plant biomass-derived black carbon (biochar). Environ. Sci. Technol., 44, 1247–1253.
- Kim YS, Makoto K, Takakai F, Shibata H, Satomura T, Takakai K, Hatano R, Koike T 2011: Greenhouse gas emissions after a prescribed fire in white birch-dwarf bamboo stands in northern Japan, focusing on the role of charcoal. Eur. J. Forest Res., 130, 1031–1044.
- Knoblauch C, Maarifat AA, Pfeiffer EM, Haefele SM 2011: Degradability of black carbon and its impact on trace gas fluxes and carbon turnover in paddy soils. Soil Biol. Biochem., 43, 1768–1778.
- Lehmann J, Gaunt J, Rondon 2006: Bio-char sequestration in terrestrial ecosystems – A review. Mitig. Adapt. Strat. Glob. Change, 11, 403–427.
- Lehmann, J, Joseph S, 2009: Biochar for Environmental Management: Science and Technology. Earthscan Press, London, UK.
- Lehmann J, Rillig M, Thies J, Masiello CA, Hockaday WC, Crowley D 2011: Biochar effects on soil biota – A review. Soil Biol. Biochem., 43, 1812–1836.
- Liu H, Jiang GM, Zhuang HY, Wang KJ 2008: Distribution, utilization structure and potential of biomass resources in rural China: With special reference of crop residues. Renew. Sust. Emerg. Rev., 12, 1402–1418.
- Liu YX, Yang M, Wu YM, Wang HL, Chen YX, Wu WX 2011: Reducing CH4 and CO2 emissions from waterlogged paddy soil with biochar. J. Soils Sediments, 11, 930–939.
- Lu F, Wang XK, Han B, Ouyang ZY, Duan XN, Zheng H 2010: Net mitigation potential of straw return to Chinese cropland: Estimation with a full greenhouse gas budget model. Ecol. Appl., 20, 634–647.
- Luo J, White RE, Roger Ball P, Tillman RW 1996: Measuring denitrification activity in soils under pasture: Optimizing conditions for the short-term denitrification enzyme assay and effect of soil storage on denitrification activity. Soil Biol. Biochem., 28, 409–417.
- Mer JL, Roger P 2001: Production, oxidation, emission and consumption of methane by soils: A review. Eur. J. Soil Biol., 37, 25–50.
- Mørkved PT, Dörsch P, Bakken LR, 2007: The N2O product ratio of nitrification and its dependence on long-term changes in soil pH. Soil Biol. Biochem., 39, 2048–2057.
- Nelissen V, Rütting T, Huygens D, Huygens D, Staelens J, Ruysschaert G, Boechx P 2012: Maize biochars accelerate short-term soil nitrogen dynamics in a loamy sand soil. Soil Biol. Biochem., 55, 20–27.
- Novak JM, Busscher WJ, Watts DW, Laird DA, Ahmedna MA, Niandou MAS 2010: Short-term CO2 mineralization after additions of biochar and switchgrass to a typic Kandiudult. Geoderma, 154, 281–288.
- O’Toole A, Knoth de Zarruk K, Steffens M, Rasse DP 2013: Characterization, stability, and plant effects of kin-produced wheat straw biochar. J. Environ. Qual., 42, 429–436.
- Pathak H, Singh R, Bhatia A, Jain N 2006: Recycling of rice straw to improve wheat yield and soil fertility and reduce atmospheric pollution. Paddy Water Environ., 4, 111–117.
- Pereira RC, Kaal J, Arbestain MC, Lorenzo RP, Aitkenhead W, Hedley M, Macías F, Hindmarsh J, Maciá-Agulló JA, 2011: Contribution to characterization of biochar to estimate the labile fraction of carbon. Org. Geochem., 42, 1331–1342.
- Scheer C, Grace PR, Rowlings DW, Kimber S, van Zwieten L, 2011: Effect of biochar amendment on the soil-atmosphere exchange of greenhouse gases from an intensive subtropical pasture in northern New South Wales, Australia. Plant Soil, 345, 47–58.
- Shneour EA, 1966: Oxidation of graphitic carbon in certain soils. Science, 151, 991–992.
- Singh BP, Hatton BJ, Singh B, Cowie AL, Kathuria A 2010: Influence of biochars on nitrous oxide emission and nitrogen leaching from two contrasting soils. J. Environ. Qual., 39, 1224–1235.
- Singh Y, Singh B, Ladha JK, Khind CS, Gupta RK, Meelu OP, Pasuquin E 2004: Long-term effects of organic input on yield and soil fertility in the rice-wheat rotation. Soil Sci. Soc. Am. J., 68, 845–853.
- Smith JL, Collins HP, Bailey VL 2010: The effect of young biochar on soil respiration. Soil Biol. Biochem., 42, 2345–2347.
- Spokas KA, Ksokinen WC, Baker JM, Reicosky DC 2009: Impacts of woodchip biochar additions on greenhouse gas production and sorption/degradation of two herbicides in a Minnesota soil. Chemosphere, 77, 574–581.
- Spokas KA, Reicosky DC 2009: Impacts of sixteen different biochars on soil greenhouse gas production. Ann. Environ. Sci., 3, 179–193.
- Ström L, Ekberg A, Mastepanov M, Christensen TR 2003: The effect of vascular plants on carbon turnover and methane emissions from a tundra wetland. Global Change Biol., 9, 1185–1192.
- USDA Soil Survey Staff, 1994: Keys to soil taxonomy, 6th ed., US Department of Agriculture, Soil Conservation Service, Lincoln, NE, USA.
- van Zwieten L, Kimber S, Morris S, Downie A, Berger E, Rust J, Scheer C 2010: Influence of biochars on flux of N2O and CO2 from Ferrosol. Aust. J. Soil Res., 48, 555–568.
- Wang C, Lu HH, Dong D, Deng H, Strong PJ, Wang HL, Wu WX 2013: Insight into the effects of biochar on manure composting: Evidence supporting the relationship between N2O emission and denitrifying community. Environ. Sci. Technol., 47, 7341–7349, doi: 10.1021/es305293h
- Wang JY, Zhang M, Xiong ZQ, Liu PL, Pan GX 2011: Effects of biochar addition on N2O and CO2 emissions from two paddy soils. Biol. Ferti. Soils, 47, 887–896.
- Weier KL, Gilliam JW 1986: Effect of acidity on denitrification and nitrous oxide evolution from Atlantic coastal plain soils. Soil Sci. Soc. Am. J., 50, 1202–1205.
- Yan XY, Ohara T, Akimoto H 2006: Bottom-up estimate of biomass burning in mainland China. Atmos. Environ., 40, 5262–5273.
- Yanai Y, Toyota K, Okazaki M 2007: Effect of charcoal addition on N2O emissions from soil resulting from rewetting air-dried soil in short-term laboratory experiments. Soil Sci. Plant Nutr., 53, 181–188.
- Zhang AF, Cui LQ, Pan GX, Li LQ, Hussain Q, Zhang XH, Zheng JW, Crowley D 2010: Effect of biochar amendment on yield and methane and nitrous oxide emssions from a rice paddy from Tai Lake plain, China. Agr. Ecosyst. Environ., 139, 469–475.
- Zou JW, Huang Y, Jiang JY, Zheng XH, Sass RL 2005: A 3-years field measurement of methane and nitrous oxide emissions from rice paddies in China: Effects of water regime, crop residues, and fertilizer application. Global Biochem. Cycles, 19, GB2021, doi: 10.1029/2004GB002401