Abstract
There are variations in soil respiration across vegetation types; however, it is unclear which factors are mainly responsible for the variations. A field experiment was conducted in 2008 and 2009 in a semiarid region of China to investigate the daytime and monthly variation of soil respiration across vegetation types and to determine the factors controlling the variation. An automated portable soil carbon dioxide (CO2) flux measurement system was used to measure the soil respiration in shrubland, grassland, fallow land, and cropland during the growing periods. The results showed that the relative daytime variation amplitude of soil respiration in the fallow land and cropland was as small as that of shrubland and grassland during July, but greater than that of shrubland and grassland during August and October. A hysteresis effect for the relationship between the daytime soil respiration and daytime soil temperature was observed for all four vegetation types. There was also a hysteresis effect for the relationship between the daytime soil respiration and daytime air temperature for the grassland. Over the study period, the monthly soil respiration rates of the fallow land and cropland were statistically comparable and significantly lower than those of the shrubland and grassland, with the exception of August, during which the monthly soil respiration of the cropland was as great as that of shrubland and grassland. The factors responsible for the monthly soil respiration variation across the vegetation types differed from month to month. In general, the soil temperature and soil water content were mainly responsible in August and September; however, the root biomass predominated in July and October. The results are valuable for accurately estimating regional carbon fluxes by considering the temporal variability of the soil respiration variation across vegetation types in the Loess Plateau of China.
1. INTRODUCTION
Soil respiration is the main carbon (C) efflux from terrestrial ecosystems into the atmosphere (Raich and Schlesinger Citation1992). Thus, information about soil respiration is very crucial in understanding the terrestrial C cycling and feedback with regard to climate change. However, quantifying soil respiration at a regional scale usually fails because soil respiration exhibits a high spatial variability within a site and over the landscape (Martin and Bolstad Citation2009). In general, soil respiration varies directly with certain abiotic and biotic factors, including soil temperature, soil moisture, belowground biomass, primary production and leaf area index (Buchmann Citation2000; Rustad et al. Citation2000; Flanagan and Johnson Citation2005; Smith Citation2005; Tanaka and Hashimoto Citation2006; Han et al. Citation2007a; Li et al., Citation2008). Because vegetation types have the potential to change these abiotic and biotic factors (Williams et al. Citation2003; Fu et al. Citation2009; Hu et al. Citation2008), it is expected that there are differences in soil respiration across vegetation types (Lessard et al. Citation1994; Wagai et al. Citation1998; Carlisle et al. Citation2006; Kellman et al. Citation2007; Zhang et al. Citation2007). However, which factors are mainly responsible for the differences in soil respiration across vegetation types remains unclear. Based on a study conducted in a managed northern Wisconsin forest landscape, Euskirchen et al. (Citation2003) argued that the average litter depth, an indicator of the aboveground productivity, was a better predictor of soil respiration among the dominant patch types than the average temperature within the studied landscape. The findings in southern Wisconsin of Wagai et al. (Citation1998) explained the observation that the soil respiration of a prairie plot was greater than that of a corn plot by considering the significant differences in the fine root biomass, and attributed the difference in the soil respiration between till and no-till corn plots to the soil temperature. Tufekcioglu et al. (Citation2001) conducted their study in Iowa and reported that perennial grass plots demonstrated higher soil respiration than did crops, and attributed this result to the greater fine root biomass and higher soil moisture in the perennial grass plots. Clearly, different systems yielded different main responsible factors for the soil respiration variation across vegetation types (Raich and Tufekcioglu Citation2000).
Additional complications in predicting the main factors responsible for the differences in soil respiration across vegetation types are introduced by coupling the spatial and temporal variations of soil respiration (Mo et al. Citation2005; Khomik et al. Citation2006; Kosugi et al. Citation2007; Panosso et al. Citation2009), which indicates that the controlling factors of spatial variation may be different from time to time rather than being consistent over the year. For example, Ohashi and Gyokusen (Citation2007) investigated the temporal change in the spatial variability of soil respiration in a Japanese cedar forest and found that the factors affecting the spatial variability were the relief index (a physiognomy parameter calculated as the sum of altitude differences between a subplot and eight points located 20 m away from the subplot in eight different directions) in autumn, the soil hardness of the A layer in winter, the soil hardness at a depth of 50 cm in spring and the altitude and relief index in summer. For cases of a system with different vegetation types, the temporal changes of factors affecting the spatial variability would be more difficult to predict because the plant phenology regulates the impact of the vegetation type on the soil respiration (Grogan and Chapin Citation1999). Baldocchi et al. (Citation2006) examined the biophysical regulators affecting the spatial-temporal variation of soil respiration in an oak (Quercus douglasii, Fagaceae)-grass (including Brachypodium distachyon, Hypochaeris glabra, Bromus madritensis, and Cynosurus echinatus) savanna. These authors reported that the soil respiration under trees was always larger than the soil respiration measured in the open grassland throughout the dry period after the grass had died; in contrast, the respiration from the grassland exceeded that from the forest understory during the wet season (winter) when greater grass growth occurred and the trees were leafless and dormant.
The Loess Plateau of China is characterized by a dense landscape of eroding canyons. To remedy the degradation caused by soil erosion, the conversion of croplands to shrublands or grasslands, or the reversion of the area to the natural vegetation, has been emphasized by the Chinese government. These efforts have resulted in a common fragmented landform consisting of mosaic vegetation types; thus, a valid estimation of soil respiration in this region depends on an understanding of the soil respiration differences among the typical vegetation types and the controlling factors contributing to these differences. In this study, we investigated the variability of soil respiration under shrubland, grassland, fallow land and cropland during the growing season in the northern region of the Loess Plateau of China. Our objectives were as follows: (1) to investigate the responses of the daytime and monthly soil respiration to the vegetation types and (2) to identify the main factors regulating the variation in soil respiration across the vegetation types on a monthly scale. This study aims to provide relevant information on the small-scale spatial variations in soil respiration and the factors affecting the soil respiration rates under different vegetation types in this region of mosaic vegetation types.
2. MATERIALS AND METHODS
2.1. Site description
The study was conducted at the Shenmu Erosion and Environment Research Station of the Institute of Soil and Water Conservation, Chinese Academy of Sciences, in the Liudaogou catchment in Shenmu County, Shaanxi, situated on the Northern Loess Plateau (38°46′–38°51′ N, 110°21′–110°23′ E). The climate in this area is categorized as semiarid, with a mean annual precipitation of approximately 437 mm, most of which falls in intense storms between June and September. The mean annual air temperature is approximately 8°C. The soil is Calcaric Regosol (Food and Agriculture Organization-United Nations Educational, Scientific, and Cultural Organization (FAO-UNESCO), 1974), with a high fragility and low fertility.
2.2. Experimental design
The study included the following four vegetation types: (1) Korshinsk Peashrub (shrubland), (2) a leguminous herb (grassland), (3) fallow for natural vegetation recovery (fallow land), and (4) cropland with millet (cropland). All four vegetation types were on a southern hillslope of 12°; the hillslope had been millet (Setaria italica) cropland for decades. The selected physical and chemical properties of this hillslope (0–10 cm soil layer) in 2004 were 15.6% clay, 44% silt, 40.4% sand, soil organic carbon (SOC) of 1.27 g kg–1, and total nitrogen (N) of 0.19 g kg–1. To remedy the serious problem of erosion, most of the cropland was converted into shrubland, grassland and fallow land in 2004, with approximate areas of 300 m2 each. The shrubs and grasses were planted with row spacings of 70 and 50 cm, respectively. The shrubs were not subjected to any harvest or fertilization after planting, whereas the grass was pruned annually in late June to stimulate its growth according to the local management. The grass and fallow lands have not received fertilization since 2004. The remaining cropland received annual tillage to a depth of 20 cm in May, and then millet was sown. The aboveground biomass of millet was harvested in late September or early October, depending on the maturity time. During the growing season, the cropland was manually weeded monthly and received an annual application of 120 and 60 kg ha–1 N and phosphorus pentoxide (P2O5), respectively, as recommended by the local agricultural service.
2.3. Soil respiration measurements
For the soil respiration measurements, three polyvinyl chloride collars (20 cm in diameter and 12 cm in height) were randomly inserted 2–3 cm into the soil within each land use area. All of the soil collars were left on site for the entire study period. Soil respiration was measured using an automated portable soil carbon dioxide (CO2) flux system (LI-8100, LI-COR, USA), and both daytime and monthly soil respiration measurements were performed. For the daytime soil respiration study, measurements were obtained every 1.5 h (between 06:00 and 20:00 h) on 2 d in July, August and October of 2008. For the monthly soil respiration study, the measurements were obtained approximately every 2 d from July to October of 2009. To present the daily mean soil respiration, all of the measurements for the monthly soil respiration study were obtained between 09:00 and 11:00 h, as suggested by earlier studies (Kessavallou et al. Citation1998; M. Xu and Qi 2001). Living plants inside the soil collars were removed by hand at least 1 d before the soil respiration measurement (W. Xu and Wan Citation2008). The soil respiration chamber was equipped with thermocouples to measure the soil surface air temperature while the chamber was closed. The 12 measurement locations were divided into three measurement groups: each group included four vegetation types, and the measurement was completed within 20 min to minimize the influence of soil temperature changes over the sampling period. Simultaneous with the soil respiration measurements, the soil temperature and soil moisture were measured at a depth of 6 cm using thermometers and a time domain reflectometry sensor (TDR), respectively.
2.4. Biotic factor measurements
The root biomass and leaf area index (LAI) around the PVC collars were measured in 2009. Root biomass to a 30-cm depth was sampled using a core sampler with a diameter of 10 cm, from July to October. The soil cores were sampled in 10-cm increments. The roots were extracted manually under running water, and the biomass samples were oven-dried at 70°C to a constant mass and weighed. Both the aboveground and root biomass measurements were replicated twice for each PVC collar during each sampling period. Ten branches of Korshinsk Peashrub, 20 branches of leguminous herb, 10 branches of Artemisia scoparia Waldst. et Kit. (dominant species of the fallow land), and 10 millet individuals were randomly selected monthly around the PVC collars for the shrubland, grassland, fallow land and cropland, respectively. The green leaf areas of the selected materials were obtained using a photography method and processed with Image-J 1.36b software (National Institute of Health, USA). To facilitate the scaling from the leaf to the canopy level, the selected materials were oven-dried at 70°C for the determination of the dry mass. The LAI can then be expressed in m2 m–2 by combining the aboveground biomass.
2.5. Data analysis
The statistical analyses were performed using SPSS 13.0 (SPSS Inc., Chicago, IL, USA), and statistical comparisons were performed using one-way analysis of variance (ANOVA) to determine the effects of the vegetation types on the monthly soil respiration, soil temperature, soil water content, root biomass and LAI. Stepwise multiple linear analyses were used to examine the relationships of the soil respiration with the soil water content (SW), soil temperature (ST), root biomass (RB), and LAI across the vegetation types for each month. The relative daytime variation amplitude of soil respiration, defined as the difference between daytime maximum and minimum value divided by the daytime mean value, was calculated to investigate the responses of daytime fluctuation extent to vegetation types. To examine the effects of the vegetation types on the relationship between the daytime soil respiration and temperature, the deviations of the soil respiration, air temperature and soil temperature from their respective daytime averages were calculated as suggested by Parkin and Kaspar (Citation2003):
3. RESULTS
3.1. Heterogeneous phenology-stage evolution
The perennial shrubs and grasses normally produced green leaves beginning in April and underwent a period of longer, slow vegetative growth during May and June because of the limited rainfall and relatively lower temperature. The plant growth accelerated in July with the increasing temperature and rainfall and reached a peak growth period in August when the rainfall was ample (). In September, the senescence of older leaves and the production of new leaves occurred concurrently; the new leaf growth generally lasted through late October. Additionally, the extent of the late leaf germination for the shrubs was more pronounced than for the grasses. For the fallow land, the Artemisia scoparia Waldst. et Kit. emerged in July and reached a peak growth period in mid-August. September was the fruiting stage, and the leaves quickly senesced in October. The millet in the cropland experienced a relatively longer seedling stage in July, vigorous tillering and anthesis in August, and maturation in September; the millet was harvested in early October.
3.2. Daytime soil respiration
Different daytime variations in soil respiration were found for the different vegetation types (). In July, the daytime soil respiration fluctuated slightly but remained relatively constant from 10:00 to 17:00 for the four vegetation types. Differences in the relative daytime variation amplitude were not substantial among the four vegetation types in July. In August and October, however, a distinct peak of daytime soil respiration was observed for the fallow land and cropland. The relative amplitudes of the daytime variation in the soil respiration from the fallow land and cropland were approximately 2.1 and 2.5 times greater than those of the shrubland and grassland in August and October. The relationship between the daytime percent deviations in the soil respiration and temperature is shown in . The soil respiration decreased in response to cooling but did not follow the curve when it increased in response to warming. The cooling curve normally fell bellow the warming curve to form a hysteresis loop. The apparent hysteresis effect for the relationship between the daytime soil respiration and daytime soil temperature was observed for all four of the vegetation types. There was also a hysteresis effect for the relationship between the daytime soil respiration and daytime air temperature for the grassland. In contrast, no significant hysteresis cycle between the daytime soil respiration and daytime air temperature was observed for the shrub land, fallow land and cropland.
Figure 2 Daytime changes of the soil respiration from 06:00 h to 20:00 h for shrubland, grassland, fallow land and cropland. Each data point represents the mean value of three measurement locations for each vegetation type.
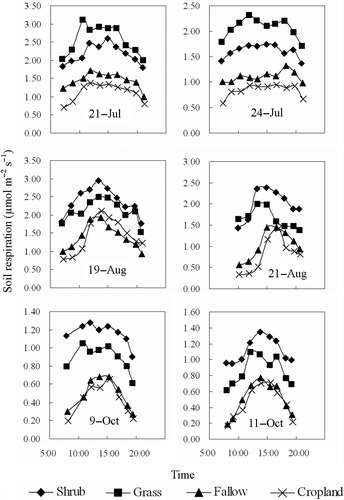
Figure 3 Relationships between the daytime soil respiration and daytime temperatures. SR (soil respiration), Ta (air temperature), and Ts (soil temperature) deviation are the percent deviation of SR, Ta, Ts from their daytime average value, respectively. Each data point was obtained from the mean value of three measurement locations for each vegetation type of the six measurement days.
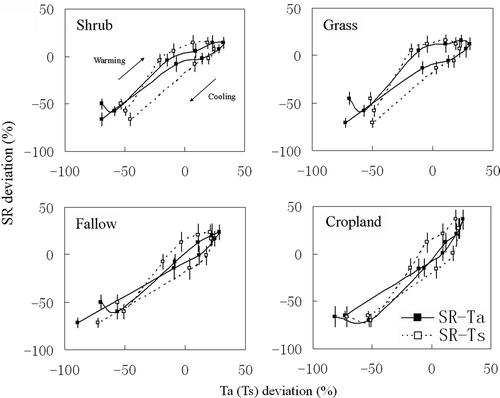
3.3. Monthly soil respiration and controlling factors
The soil respiration trend for each vegetation type over the study period is shown in . For the four vegetation types, the soil respiration was greater during July and August and declined to a lower level in September and October. shows the effects of vegetation types on the monthly soil respiration. There were no significant differences in the monthly soil respiration for the shrubland and grassland between July and September. In October, however, the shrubland exhibited significantly greater soil respiration than the grassland. Over the study period, the monthly soil respiration of the fallow land and cropland were statistically comparable and significantly lower than the shrubland and grassland, with the exception of August during which the monthly soil respiration of the cropland was as great as that of the shrubland and grassland. Overall, the cumulative daytime soil respiration over the study period of the cropland was significantly greater than that of the fallow land, and statistically as high as that of the shrubland and grassland ().
Figure 4 Soil respiration, soil temperature and soil water content for the shrubland, grassland, fallow land and cropland. Each data point represents the mean value of three measurement locations for each vegetation type.
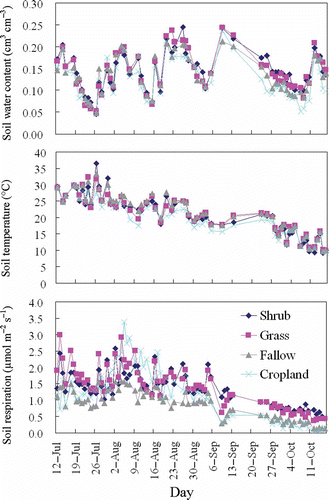
Figure 5 Effects of the vegetation types on soil respiration (SR, µmol m–2 s–1), soil water content (SW, 0.1 cm3 cm–3), soil temperature (ST, 10°C), root biomass (RB, 10 g m–2), and leaf area index (LAI, m2 m–2) for each month. Values followed by different lower-case letters within columns are significantly different at P < 0.05.
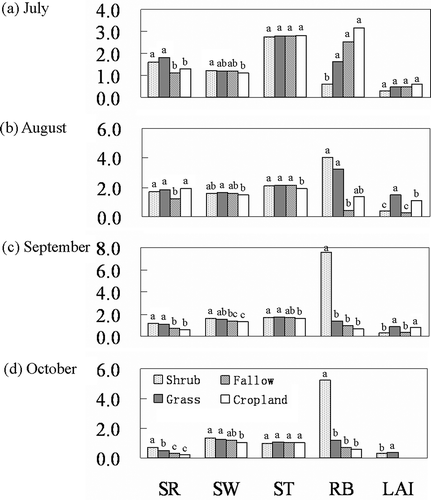
Figure 6 Cumulative daytime soil respiration over the study period of 123 d for the shrubland, grassland, fallow land and cropland. Values followed by different lower-case letters are significantly different at P < 0.05.

The effects of the vegetation types on the soil water content, soil temperature, root biomass and LAI for each month are demonstrated in . In general, the soil water content in the shrubland was significantly higher than in the cropland but statistically comparable with that of the grassland and fallow land during the study period. Significant differences in the soil temperature among the vegetation types were observed in August and September, for which the cropland had the lowest values. The root biomass and LAI varied from month to month. The root biomass in the shrubland was the lowest in July but significantly higher during September and October. In August, however, the root biomass values in the shrubland, grassland and cropland were statistically comparable. There was no significant difference in the LAI among the vegetation types during July. In August and September, the LAI values from the shrub and fallow areas were comparable and significantly lower than those of the grassland and cropland.
A multiple stepwise regression analysis was conducted for the relationship of the monthly soil respiration across the vegetation types with the corresponding biotic and abiotic factors (). In July, the root biomass alone explained 33.4% of the variation of the soil respiration across the vegetation types; in August, the soil respiration correlated negatively with the soil temperature but positively with the LAI. The soil temperature and LAI can explain 49.6 and 22.4% of the changes in the soil respiration across the vegetation types, respectively. For September, 42.8% of the variation of soil respiration can be explained by soil water content; for October, the root biomass alone explained 55.6% of the soil respiration variation across the land uses.
Table 1 Results of multiple stepwise regression analysis (n = 12, three monthly averages of each vegetation type) for the relationships of soil respiration (SR) with soil water content (SW), soil temperature (ST), root biomass (RB), and leaf area index (LAI) for each month.
4. DISCUSSION
4.1. Daytime soil respiration variation and vegetation type
The fact that the differences in the relative daytime variation amplitude of soil respiration among the vegetation types varied over the study period could be explained by considering the variation of photosynthesis activity because photosynthesis can modulate soil respiration on a diurnal scale (Högberg et al. Citation2001; Tang et al. Citation2005). During July, the longer sunlight duration resulted in the smaller differences in the daytime air temperature () and, consequently, yielded the smaller daytime variation in the soil respiration for the four vegetation types. During August and October, however, the greater differences in the daytime air temperature and shorter sunlight duration () were responsible for the greater relative amplitude of the daytime soil respiration variation.
The vegetation type influenced the relationship between the daytime soil respiration and daytime temperature (). Previous studies reported that soil respiration was strongly correlated with the soil temperature (Cao et al. Citation2004; Mo et al. Citation2005; Ding et al. Citation2006; Jia et al. Citation2006; Han et al. Citation2007b; Pavelka et al. Citation2007; Chang et al. Citation2008; Zhang et al. Citation2009). Our results showed that there was an apparent hysteresis effect in the relationship between the daytime soil respiration and soil temperature for the four vegetation types, which has also been observed in other forest or grass ecosystems (Bahn et al. Citation2008; Ruehr et al. Citation2010). Although previous research demonstrated that the diurnal soil respiration could have a stronger temporal coincidence with the air temperature (Parkin and Kaspar Citation2003), our results showed that such temporal coincidence did not exist in the grassland. The hysteresis effects discussed above indicated that using a shallow soil temperature to estimate soil respiration at short time scales in this study site involves risks.
4.2. Monthly soil respiration and vegetation type
Soil respiration from the shrubland and grassland was comparable and higher than that of the fallow land and cropland during most months of the study period. However, we observed that the soil respiration from the cropland was statistically comparable with that of the shrubland and grassland during August. This finding is inconsistent with a study conducted in another semiarid region by Frank et al. (Citation2006), who reported that the soil respiration of grassland was consistently greater than that of cropland. The inconsistent findings are likely caused by the following reasons. First, the grassland in the study of Frank et al. (Citation2006) was native prairie and had a significantly larger soil organic matter content to a soil depth of 0.3 m compared to the cropland. The grassland in the present study was converted from cropland 5 yr prior, and a previous study of the same site showed that the recent establishment of grassland did not significantly increase the soil organic matter (Fu et al. Citation2010). Therefore, the soil respiration from the grassland in the present study was not as great as that observed in Frank et al. (Citation2006). Second, Frank et al. (Citation2006) measured soil respiration approximately every 21 d, and this relatively low frequency could cause one to overlook a high respiration period that may occur in croplands. A study by Wagai et al. (Citation2008) lends support to the second explanation because these authors measured soil respiration approximately weekly, showing that soil respiration under tilled corn was larger than that of prairie land on two consecutive measurement days. Data from the present and the previous study further suggest that consecutive measurements are vital to quantify the differences of soil respiration across vegetation types validly, especially when the type of vegetation displays a vigorous tillering stage.
4.3. Factors that regulate the monthly soil respiration across vegetation types
The soil respiration components include autotrophic respiration (generally referred to as root respiration) and heterotrophic respiration (soil microorganisms utilizing pre-formed C compounds) (Baggs Citation2006). Various factors regulate the spatial variation of soil respiration across vegetation types by altering different components of soil respiration. Earlier findings showed that perennial plants significantly increased the CO2 efflux compared with crop plants (Larionova et al. Citation1998; Wagai et al. Citation1998; Tufekcioglu et al. Citation2001; Guner et al. Citation2010) because of their enhancement of soil heterotrophic respiration by larger input fresh plant debris (Schlesinger and Andrews Citation2000; Lohila et al. Citation2003; Chiti et al. Citation2011). Our previous study at the same site showed that the soil organic C in shrubland was statistically comparable to that of cropland but was significantly greater than that of fallow land in 2007 (Fu et al. Citation2010). The variation pattern of soil organic C among the vegetation types was similar to that of cumulative daytime soil respiration throughout the experimental period, but different from the monthly soil respiration ( and ). Therefore, the explanation of the soil organic matter in the soil respiration variation among vegetation types is likely to be valid on larger time scale (e.g., yearly). On a smaller time scale (e.g., monthly), the other readily changeable factors, such as the soil moisture, soil temperature, LAI and root biomass, would be expected to be more effective.
The soil moisture and temperature can influence soil respiration directly by altering the activities of plant roots and soil microbes, and indirectly by altering the plant biomass production and substrate supply (Wan et al. Citation2007). The vegetation type had significant effects on the monthly soil water content during the study period, a result that is consistent with the findings of Hu et al. (Citation2008), who also reported that there was a significant spatial variability in the surface soil moisture among different vegetation types in the same study area. Although the soil respiration was highly sensitive to the soil moisture in semiarid regions (Kaye and Hart Citation1998; Conant et al. Citation2000, Citation2004), we found that the soil moisture was the main reason for soil respiration variation across the vegetation types only during September (). Even though there was evidence showing that the soil moisture status is the critical factor that determines the spatial variation in the soil respiration on a site-to-site scale (Reichstein et al. Citation2003; Chen et al. Citation2010), our results supported the argument that the soil moisture may be the dominant determinant of the temporary and spatial variations of the soil respiration at individual sites but may be insufficient to characterize the soil respiration spatially among sites (Epron et al. Citation2004; Hibbard et al. Citation2005). The soil temperature was significantly different among the vegetation types during August and September and was mainly responsible for the soil respiration variations across the vegetation types in August (). However, the finding that the soil respiration negatively correlated with the soil temperature was in contrast to the previous inter-site seasonal variation studies (Scott-Denton et al. Citation2003; Dornbush and Raich Citation2006; Gaumont-Guay et al. Citation2006). Although the LAI exerted some influence on the soil respiration in August, the biotic factors mostly regulated it in July and October through root biomass.
The result that different months displayed different main responsible factors suggests that weighting of influence factors fluctuates with time. Such fluctuation has been well displayed within certain vegetation types for a longer time scale. For example, Larionova et al. (Citation1998) found that the effect of soil moisture on the soil respiration during the growing period of grass (a mixture of Festuca pratensis (Huds), Phleum pratense (L.) and Trifolium pratense (L.)) and buckwheat (Fagopyrum esculentum Moench) was greater than that of the soil temperature, whereas the soil temperature was the dominant factor during the cold seasons. Across vegetation types, Grogan and Chapin (Citation1999) reported that the vegetation type had little impact during the winter but was the principal controlling factor in summer. Although Grogan and Chapin (Citation1999) indicated that seasonality was the reason for the weighting fluctuation of soil respiration influence factors, we would like to attribute it to the heterogeneous phenology-stage evolution as well, because the biological processes of leaf emergence and senescence (biological switches) would impose considerable impacts on the weights of the soil respiration components. For example, a study in an oak-grass savanna reported that during the dry summer, heterotrophic respiration was dominant in a senesced grassland area, whereas the autotrophic respiration by roots and the feeding on root exudates by microbes was dominant under trees (Baldocchi et al. Citation2006). When a cropland with a short growth period and vigorous tillering stage was involved, the effects of biological switches would be pronounced. In a maize-wheat rotation system in a humid region of China, the cropland demonstrated the similar seasonal variations in soil respiration as forest and grassland, but its maximum soil respiration rate occurred approximately 30 d earlier (Wang et al. Citation2008). In the present study using a millet crop, the cropland presented a distinct peak stage of soil respiration during the vigorous tillering stage of August (). The biological switch is likely to be the reason for the fact that the soil respiration of cropland was as high as that of grassland and shrubland in this month ().
5. CONCLUSIONS
The Loess Plateau of China is characterized by a dense landscape of eroding canyons and a highly mosaic vegetation. In this study, we investigated the soil respiration of four dominant vegetation types at both daily and monthly intervals. Compared with cropland, the perennial shrubland and grassland are prone to decrease in the amplitude of the daytime soil respiration variation. The soil respiration rate in the cropland was as high as those of the shrubland and grassland during August, which meant that the cumulative soil respirations of these three vegetation types were also statistically comparable. Heterogeneous phenology-stage evolution resulted in different main controlling factors for the soil respiration variation across the vegetation types on a month-to-month basis. The soil temperature and soil water content were mainly responsible in August and September, whereas the root biomass predominated in July and October. These findings suggest that abiotic factors (such as the soil temperature and soil water content) are insufficient to assess the spatio-temporal variability of soil respiration in the estimation of the regional C fluxes in the study region, and that biotic factors (such as the root biomass and LAI) should also be considered.
ACKNOWLEDGMENTS
We gratefully acknowledge the field assistance of Wujun Zeng, Junliang Zhou, Xiaoxu Jia, Xuezhang Li, Wei Hu, Fengpeng Han, Hongbei Gao, Chuanqin Huang and Danfeng Li, and the technological support (LI-8100) of Xuanbin Xu. This work was funded by the National Key Basic Research Special Foundation Project (2010CB833501), the National Natural Science Foundation of China (41101203, 31070559) and the Foundations of State Key Laboratory of Soil Erosion and Dryland Farming on the Loess Plateau (10501-279).
REFERENCES
- Baggs EM 2006: Partitioning the components of soil respiration: A research challenge. Plant Soil, 284, 1–5.
- Bahn M, Rodeghiero M, Anderson-Dunn M et al. 2008: Soil respiration in European grasslands in relation to climate and assimilate supply. Ecosystems, 11, 1352–1367.
- Baldocchi D, Tang J, Xu L 2006: How switches and lags in biophysical regulators affect spatial-temporal variation of soil respiration in an oak-grass savanna. J. Geophys. Res., 111, G02008, doi: 10.1029/2005JG000063
- Buchmann N 2000: Biotic and abiotic factors controlling soil respiration rates in Picea abies stands. Soil Biol. Biochem., 32, 1625–1635.
- Cao G, Tang Y, Mo W, Wang Y, Li Y, Zhao X 2004: Grazing intensity alters soil respiration in an alpine meadow on the Tibetan plateau. Soil Biol. Biochem., 36, 237–243.
- Carlisle EA, Steenwerth KL, Smart DR, 2006: Effects of land use on soil respiration: Conversion of oak woodlands to vineyards. J. Environ. Qual., 35, 1396–1404.
- Chang S, Tseng K, Hsia Y, Wang C, Wu J 2008: Soil respiration in a subtropical montane cloud forest in Taiwan. Agric. Forest Meteorol., 148, 788–798.
- Chen Q, Wang Q, Han X, Wan S, Li L, 2010: Temporal and spatial variability and controls of soil respiration in a temperate steppe in northern China. Global Biogeochem. Cycles, 24, GB2010, doi: 10.1029/2009GB003538
- Chiti T, Neubert REM, Janssens IA, Yuste JC, Sirignano C, Certini G 2011: Radiocarbon based assessment of soil organic matter contribution to soil respiration in a pine stand of the Campine region, Belgium. Plant Soil, 344, 273–282.
- Conant RT, Dalla-Betta P, Klopatek CC, Klopatek JM 2004: Controls on soil respiration in semiarid soils. Soil Biol. Biochem., 36, 945–951.
- Conant RT, Klopatek JM, Klopatek CC 2000: Environmental factors controlling soil respiration in three semiarid ecosystems. Soil Sci. Soc. Am. J., 64, 383–390.
- Ding W, Cai Y, Cai Z, Zheng X 2006: Diel pattern of soil respiration in N-amended soil under maize cultivation. Atmos. Environ., 40, 3294–3305.
- Dornbush ME, Raich JW 2006: Soil temperature, not aboveground plant productivity, best predicts intra-annual variations of soil respiration in Central Iowa grasslands. Ecosystems, 9, 909–920.
- Epron D, Nouvellon Y, Roupsard O et al. 2004. Spatial and temporal variations of soil respiration in a Eucalyptus plantation in Congo. Forest Ecol. Manage., 202, 149–160.
- Euskirchen ES, Chen J, Gustafson EJ, Ma S 2003: Soil respiration at dominant patch types within a managed northern Wisconsin landscape. Ecosystems, 6, 595–607.
- Flanagan LB, Johnson BG 2005: Interacting effects of temperature, soil moisture and plant biomass production on ecosystem respiration in a northern temperate grassland. Agric. Forest Meteorol., 130, 237–253.
- FAO–UNESCO 1974: Soil Map of the World, Vol. 1–4. United Nations Educational, Scientific and Cultural Organization, Paris.
- Frank AB, Liebig MA, Tanaka DL 2006: Management effects on soil CO2 efflux in northern semiarid grassland and cropland. Soil Till. Res., 89, 78–85.
- Fu X, Shao M, Wei X, Horton R 2009: Effects of two perennials, fallow and millet on distribution of phosphorous in soil and biomass on sloping loess land, China. Catena, 77, 200–206.
- Fu X, Shao M, Wei X, Horton R 2010: Soil organic carbon and total nitrogen as affected by vegetation types in Northern Loess Plateau of China. Geoderma, 155, 31–35.
- Gaumont-Guay D, Black TA, Griffis TJ, Barr AG, Morgenstern K, Jassal RS, Nesic Z 2006: Influence of temperature and drought on seasonal and interannual variations of soil, bole and ecosystem respiration in a boreal aspen stand. Agric. Forest Meteorol., 140, 203–219.
- Grogan P, Chapin FS 1999: Arctic soil respiration: Effects of climate and vegetation depend on season. Ecosystems, 2, 451–459.
- Guner S, Tufekcioglu A, Gulenay S, Kucuk M 2010: Land-use type and slope position effects on soil respiration in black locust plantations in Artvin, Turkey. African J. Agric. Res., 5, 719–724.
- Han G, Zhou G, Xu Z, Yang Y, Liu J, Shi K 2007a: Biotic and abiotic factors controlling the spatial and temporal variation of soil respiration in an agricultural ecosystem. Soil Biol. Biochem., 39, 418–425.
- Han G, Zhou G, Xu Z, Yang Y, Liu J, Shi K 2007b: Soil temperature and biotic factors drive the seasonal variation of soil respiration in a maize (Zea mays L.) agricultural ecosystem. Plant Soil, 291, 15–26.
- Hibbard KA, Law BE, Reichstein M, Sulzman J 2005: An analysis of soil respiration across northern hemisphere temperate ecosystems. Biogeochemistry, 73, 29–70.
- Högberg P, Nordgren A, Buchmann N, Taylor AFS, Ekblad A, Högberg MN, Nyberg G, Ottosson-Löfvenius M, Read DJ 2001: Large-scale forest girdling shows that current photosynthesis drives soil respiration. Nature, 411, 789–791.
- Hu W, Shao M, Wang Q, Reichardt K 2008: Soil water content temporal-spatial variability of the surface layer of a Loess Plateau hillside in China. Sci. Agric., 65, 277–289.
- Jia B, Zhou G, Wang Y, Wang F, Wang X 2006: Effects of temperature and soil water-content on soil respiration of grazed and ungrazed Leymus chinensis steppes, Inner Mongolia. J. Arid Environ., 67, 60–76.
- Kaye JP, Hart SC 1998: Restoration and canopy-type effects on soil respiration in a ponderosa pine–bunchgrass ecosystem. Soil Sci. Soc. Am. J., 62, 1062–1072.
- Kellman L, Beltrami H, Risk D 2007: Changes in seasonal soil respiration with pasture conversion to forest in Atlantic Canada. Biogeochemistry, 82, 101–109.
- Kessavallou A, Mosier AR, Doran JW, Drijber RA, Lyon DJ, Heinemeyer O 1998: Fluxes of carbon dioxide, nitrous oxide, and methane in grass sod and winter-wheat fallow tillage management. J. Environ. Qual., 27, 1094–1104.
- Khomik M, Arain MA, McCaughey JH 2006: Temporal and spatial variability of soil respiration in a boreal mixedwood forest. Agric. Forest Meteorol., 140, 244–256.
- Kosugi Y, Mitani T, Itoh M, Noguchi S, Tani M, Matsuo N, Takanashi S, Ohkubo S, Nik AR 2007: Spatial and temporal variation in soil respiration in a southeast Asian tropical rainforest. Agric. Forest Meteorol., 147, 35–47.
- Larionova AA, Yermolayev AM, Blagodatsky SA, Rozanova LN, Yevdokimov IV, Orlinsky DB 1998: Soil respiration and carbon balance of gray forest soils as affected by land use. Biol. Fertil. Soils, 27, 251–257.
- Lessard R, Rochette P, Topp E, Pattey E, Desjardins RL, Beaumont G 1994: Methane and carbon dioxide fluxes from poorly drained adjacent cultivated and forest sites. Can. J. Soil Sci., 74, 139–146.
- Li H, Yan J, Yue X, Wang M 2008: Significance of soil temperature and moisture for soil respiration in a Chinese mountain area. Agric. Forest Meteorol., 148, 490–503.
- Lohila A, Aurela M, Regina K, Laurila1 T 2003: Soil and total ecosystem respiration in agricultural fields: Effect of soil and crop type. Plant Soil, 251, 303–317.
- Martin JG, Bolstad PV 2009: Variations of soil respiration at three spatial scales: Components within measurements, intra-site variation and patterns on the landscape. Soil Biol. Biochem., 41, 530–543.
- Mo W, Lee M, Uchida M, Inatomi M, Saigusa N, Mariko S, Koizumi H 2005: Seasonal and annual variations in soil respiration in a cool-temperate deciduous broad-leaved forest in Japan. Agric. Forest Meteorol., 134, 81–94.
- Ohashi M, Gyokusen K 2007: Temporal change in spatial variability of soil respiration on a slope of Japanese cedar (Cryptomeria Japonica, D. Don) forest. Soil Biol. Biochem., 39, 1130–1138.
- Panosso AR, Marques Jr J, Pereira GT, La Scala Jr N 2009: Spatial and temporal variability of soil CO2 emission in a sugarcane area under green and slash-and-burn managements. Soil Tillage Res., 105, 275–282.
- Parkin TB, Kaspar TC 2003: Temperature controls on diurnal carbon dioxide flux: Implications for estimating soil carbon loss. Soil Sci. Soc. Am. J., 67, 1763–1772.
- Pavelka M, Acosta M, Marek MV, Kutsch W, Janous D 2007: Dependence of the Q10 values on the depth of the soil temperature measuring point. Plant Soil, 292, 171–179.
- Raich JW, Schlesinger WH 1992: The global carbon dioxide flux in soil respiration and its relationship to vegetation and climate. Tellus, 44B, 81–99.
- Raich JW, Tufekcioglu A 2000: Vegetation and soil respiration: Correlation and controls. Biogeochemistry, 48, 71–90.
- Reichstein M, Rey A, Freibauer A et al. 2003: Modeling temporal and large-scale spatial variability of soil respiration from soil water availability, temperature and vegetation productivity indices. Global Biogeochem. Cycles, 17, 15.1–15.15.
- Ruehr NK, Knohl A, Buchmann N 2010: Environmental variables controlling soil respiration on diurnal, seasonal and annual time-scales in a mixed mountain forest in Switzerland. Biogeochemistry, 98, 153–170.
- Rustad L, Huntington TG, Boone RD 2000: Controls on soil respiration: Implications for climate change. Biogeochemistry, 48, 1–6.
- Schlesinger WH, Andrews JA 2000: Soil respiration and the global carbon cycle. Biogeochemistry, 48, 7–20.
- Scott-Denton LE, Sparks KL, Monson RK 2003: Spatial and temporal controls of soil respiration rate in a high-elevation, subalpine forest. Soil Biol. Biochem., 35, 525–534.
- Smith VR 2005: Moisture, carbon and inorganic nutrient controls of soil respiration at a sub-Antarctic island. Soil Biol. Biochem., 37, 81–91.
- Tanaka K, Hashimoto S 2006: Plant canopy effects on soil thermal and hydrological properties and soil respiration. Ecol. Model., 196, 32–44.
- Tang J, Baldocchi DD, Xu L 2005: Tree photosynthesis modulates soil respiration on a diurnal time scale. Global Change Biol., 11, 1298–1304.
- Tufekcioglu A, Raich JW, Isenhart TM, Schultz RC 2001: Soil respiration within riparian buffers and adjacent crop fields. Plant Soil, 229, 117–124.
- Wagai R, Brye KR, Gower ST, Norman JM, Bundy LG 1998: Land use and environmental factors influencing soil surface CO2 flux and microbial biomass in natural and managed ecosystems in southern Wisconsin. Soil Biol. Biochem., 30, 1501–1509.
- Wan S, Norby RJ, Ledford J, Weltzin F 2007: Responses of soil respiration to elevated CO2, air warming, and changing soil water availability in a model old-field grassland. Global Change Biol., 13, 2411–2424.
- Wang X, Zhu B, Gao M, Wang Y, Zheng X 2008: Seasonal variations in soil respiration and temperature sensitivity under three land-use types in hilly areas of the Sichuan Basin. Aus. J. Soil Res., 46, 727–734.
- Williams AG, Ternan JL, Fitzjohn C, de Alba S, Perez-Gonzalez A 2003: Soil moisture variability and land use in a seasonally arid environment. Hydrol. Process., 17, 225–235.
- Xu M, Qi Y 2007: Soil-surface CO2 efflux and its spatial and temporal variations in a young ponderosa pine plantation in northern California. Global Change Biol., 7, 667–677.
- Xu W, Wan S 2008: Water- and plant-mediated responses of soil respiration to topography, fire, and nitrogen fertilization in a semiarid grassland in northern China. Soil Biol. Biochem., 40, 679–687.
- Zhang L, Chen Y, Li W, Zhao R 2007: Seasonal variation of soil respiration under different land use/land cover in arid region. Sci. China (D), 50 (supp. 1), 76–85.
- Zhang L, Chen Y, Li W, Zhao R 2009: Abiotic regulators of soil respiration in desert ecosystems. Environ. Geol., 57, 1855–1864.