Abstract
We evaluated the validity of Tessier’s method as applied to the extraction of manganese (Mn) and iron (Fe) oxides in Japanese Andisols and other soil types in Japan. Using the original Tessier’s extractant mixture, 0.04 mol L−1 hydroxylamine hydrochloride in 25% acetic acid (0.04 mol L–1 NH2OH-HCl in 25% HOAc), we found that substantial amounts of short-range-ordered Fe oxides were not extracted from allophanic Andisol samples and that considerable amounts of total Fe oxides were not extracted from all soil types. Relatively high extraction pH and large amounts of short-range-ordered Fe oxides in the Andisol samples might be responsible for incomplete extraction. Stoichiometric calculation indicated that the concentration of NH2OH-HCl might be insufficient for complete extraction of Fe oxides. The extracted amounts of Mn and Fe increased with increasing concentration of NH2OH-HCl in the extractant, and most of the Mn and Fe oxides in the soil samples, including samples with as much as 5.6% Fe, were extracted with 0.6 mol L–1 NH2OH–HCl in 25% HOAc. As judged from the simultaneous dissolution of aluminum (Al) and silicon (Si) minerals, extraction selectivity of Fe oxides with 0.6 mol L–1 NH2OH-HCl in 25% HOAc was comparable to that of the original Tessier’s method and better than that of a modified Community Bureau of Reference (BCR) sequential extraction procedure or a method using an extractant consisting of a mixture of oxalate and ascorbate, especially for Andisol samples.
INTRODUCTION
Because of the importance of manganese (Mn) and iron (Fe) oxides to the dynamics of heavy metals in the environment, many methods for extracting Mn and Fe oxides and occluded heavy metals have been developed. A common method is extraction with acidified 0.5 mol L–1 hydroxylamine hydrochloride (NH2OH-HCl), the second-step fraction in the modified Community Bureau of Reference (BCR) sequential extraction procedure (SEP) (Sahuquillo et al. Citation1999). However, Suda et al. (Citation2012) reported that this method was not suitable for Japanese Andisols, especially allophanic Andisols, which showed poor extraction selectivity and low rates of extraction of short-range-ordered Fe oxides such as ferrihydrite.
Dithionite-citrate has been used to extract both short-range ordered and well-crystalline Fe oxides in soil samples (McKeague and Day Citation1966). However, commercial dithionite could be contaminated with zinc; in addition, target metals would be precipitated as sulfide or sulfate (Gleyzes et al. Citation2002). Oxalate solutions and mixtures of oxalate solution and ascorbic acid have also been used for extracting heavy metals occluded in short-range ordered and total Fe oxides, respectively (Miller et al. Citation1986; Wilcke et al. Citation1998). One of the critical disadvantages of these oxalate-containing solutions is precipitation of some metals [cadmium (Cd) and lead (Pb)] as oxalate salts (Benitez and Dubois Citation1999).
Many researchers have used Tessier’s SEP (Tessier et al. Citation1979) for fractionation of heavy metals in soil samples. Tessier et al. (Citation1979) used “sediment” samples, not “soil” samples, but most researchers have used Tessier’s procedure for the analysis of heavy metals in soil samples without any significant modifications. In the original Tessier’s method, sediment samples with heavy metals bound to Mn and Fe oxides are extracted with 0.04 mol L–1 NH2OH-HCl in 25% acetic acid (HOAc) in boiling water for 6 h with occasional agitation (HH004-A extraction). Six hours was sufficient to extract all Fe oxides from the sediment samples in Tessier’s study. Therefore, the target phase of the Tessier’s method is generally considered to be heavy metals occluded in all Mn and Fe oxides, including short-range ordered to highly crystalline phases (e.g., Tack et al. Citation2006). However, on the basis of selective sequential extraction data and X-ray adsorption near-edge structure spectroscopy, La Force and Fendorf (Citation2000) pointed out that part of the crystalline Fe oxide was not extracted from sediments contaminated with mine waste. This result indicates that the extractability of Fe oxides with HH004-A extraction depends on the properties of the sediment or soil samples, especially their Fe oxide content and crystallinity.
Despite its widespread use, Tessier’s SEP has not been evaluated and sufficiently modified for various soil types. In Japanese agricultural fields, Andisols are among the most common soil types containing high amounts of Mn and Fe (Takeda et al. Citation2004). Mn oxides and short-range-ordered Fe oxides in Andisol samples are hardly extracted by 0.1 mol L–1 NH2OH-HCl (pH 2.0) and 0.5 mol L–1 NH2OH-HCl (pH 1.5), respectively (Suda et al. Citation2011, 2012). Inceptisols, classified as Brown Forest Soils in the Japanese soil classification system, are also widely distributed in Japan and generally contain abundant highly crystalline Fe oxides such as goethite. Fe oxides in these common Japanese soils might be incompletely extracted with HH004-A extraction, and better extractants might be needed for these soils.
Here we evaluate the original Tessier’s HH004-A extraction method as applied to samples of Japanese Andisols and other major soil types in Japan and improve the method to completely extract Mn and Fe oxides in these soils.
MATERIALS AND METHODS
Soil samples and their properties
Basic information about the three Entisols (E), three Inceptisols (I), five allophanic Andisols (AA), and three non-allophanic Andisols (NA) used in this study is compiled in . The soil samples were air-dried, passed through 2-mm mesh sieves, and stored at room temperature.
Table 1 Brief description of soil samples
Soil pH was measured in H2O or 1 mol L–1 potassium chloride (KCl) with a glass electrode pH meter. The ratio of soil sample to H2O or soil sample to 1 mol L–1 KCl was 1:2.5 (weight/volume). Total carbon content of the soil sample was measured by a dry combustion method (Sumigraph NC-22F, Shimadzu, Kyoto, Japan). Dithionite-citrate extractable Fe (Fed) and Mn (Mnd), and ammonium oxalate-extractable Al, Fe, Mn, and Si (Alo, Feo, Mno, and Sio) were extracted as described by Blakemore et al. (Citation1987). Then the extract was centrifuged and filtrated by a 0.2-µm membrane filter (Merck Millipore, Burlington, MA, USA). Detailed extraction procedures for Fed, Feo, and Mno are described in subsequent paragraphs. Pyrophosphate-extractable Al and Fe (Alp and Fep) were extracted and centrifuged as described by Blakemore et al. (Citation1987), and the supernatant was filtered through a 0.025-µm filter (Merck Millipore) to minimize contamination of Fe from microcrystalline minerals (Schuppli et al. Citation1983). The concentrations of these elements in the filtrate were determined by inductively coupled plasma optical emission spectrometry (ICP-OES) (Vista-Pro, Varian, Palo Alto, CA, USA). Clay content was determined by a pipette method (Gee and Bauder Citation1986).
Experiment 1: Effects of increasing NH2OH-HCl concentration in NH2OH-HCl-HOAc extractant on the rates of extraction of Mn and Fe oxides
Extraction with 0.04, 0.2, 0.4, and 0.6 mol L–1 NH2OH-HCl in 25% HOAc solution
The extractants used in this experiment are NH2OH-HCl in 25% HOAc (HH-A) solutions. To determine the optimal NH2OH-HCl concentration for extraction of Mn and Fe oxides in soil samples, we evaluated the effect of increasing NH2OH-HCl concentration in NH2OH-HCl-HOAc extractant. For this purpose, we used NH2OH-HCl concentrations of 0.04, 0.2, 0.4, and 0.6 mol L–1 (HH004-A, HH02-A, HH04-A, and HH06-A).
An air-dried soil sample (1 g) was placed in a 50-mL polyethylene centrifuge tube, and 20 mL of HH-A solution was added. The mixture was then heated in boiling water for 6 h with occasional agitation. After extraction, the tube was cooled to approximately room temperature with running water for 10 min. After centrifugation at 3500 rpm (1700 g) for 10 min, the supernatant was filtered through a 0.2-μm mesh membrane filter, and the filtrate was diluted with 1% nitric acid. The pH of the residual supernatant was measured with a glass electrode pH meter. Fe, Mn, Al, and Si concentrations in the diluted solution were determined by ICP-OES. All extractions and measurements were carried out in triplicate. The extraction with original Tessier’s extractant of HH004-A aims to extract both short-range-ordered and crystalline Mn and Fe oxides as well as metals occluded in these oxides.
Extraction of exchangeable and specifically adsorbed Mn and Fe
Exchangeable and specifically adsorbed Mn and Fe were extracted with a mixture of 1 mol L–1 acetic acid and ammonium acetate (pH 5.0) (hereafter “acetate” solution). Air-dried soil (0.8 g) was placed in a 50-mL polyethylene centrifuge tube, 16 mL of acetate solution was added, and the mixture was mechanically shaken at room temperature for 2 h. After centrifugation at 3500 rpm (1700 g) for 10 min, the supernatant was filtered through a 0.2-μm mesh membrane filter, and the filtrate was diluted with 1% nitric acid. Mn and Fe concentrations in the diluted solution were determined by ICP-OES. All extractions and measurements were carried out in duplicate. Experimental conditions were based on those of Salbu et al. (Citation1998).
Extraction of total Mn oxides
The amount of total Mn oxides was approximated using the value of oxalate-extractable Mn (Mno) according to Jarvis (Citation1984). An air-dried soil sample (0.4 g) was placed in a 50-mL polyethylene centrifuge tube, and 40 mL of oxalate solution (mixture of 0.2 mol L–1 oxalic acid and 0.2 mol L–1 ammonium oxalate solution, pH 3.0) was added. The mixture was then mechanically shaken at 25°C for 4 h in the dark, a drop of polymeric flocculants (Superfloc, Kemira Co., Ltd., Helsinki, Finland) was added to an aliquot (about 25 mL) of the extract, and the resulting mixture was manually shaken. After centrifugation at 3500 rpm (1700 g) for 10 min, the supernatant was filtered through a 0.2-μm mesh membrane filter, and the filtrate was diluted with 1% nitric acid (HNO3).
Oxalate is likely to extract Mn bound to organic matter in addition to oxide-forming Mn. Therefore, the amount of total Mn oxides should be estimated by the difference between the amount of oxalate-extractable Mn and the amount of organically bound Mn. Organically bound Mn is extracted with pyrophosphate (e.g., Iu et al. Citation1982) or hydrogen peroxide (e.g., Shuman Citation1979), but pyrophosphate extracts not only organically bound Mn but also oxide-forming Mn (Manh et al. Citation2010). Moreover, oxalate is produced by hydrogen peroxide treatment (Harada and Inoko Citation1977), resulting in dissolution of Mn oxides. Therefore, quantifying organically bound Mn is difficult. McBride (Citation1982), however, showed that strong binding of Mn to organic matter is unlikely at pH < 6.0. Because the soil samples that are rich in organic matter in this study (i.e., the non-allophanic Andisols) are either moderately or strongly acidic (pH 4.43 to 5.67), the amount of organically bound Mn extracted is negligible. Thus, we approximated the amount of total Mn oxides as the Mno value.
Extraction of short-range-ordered Fe oxides
Oxalate extraction was used to approximate the amounts of short-range-ordered Fe oxides according to the method described by Blakemore et al. (Citation1987). An air-dried soil sample (0.4 g) was placed in a 50-mL polyethylene centrifuge tube, and 40 mL of oxalate solution (0.2 mol L–1 oxalic acid and ammonium oxalate solution, pH 3.0) was added. The mixture was mechanically shaken at 25°C for 4 h in the dark, a drop of the polymeric flocculants was added to an aliquot (about 25 mL) of the extract, and the resulting mixture was manually shaken. After centrifugation at 3500 rpm (1700 g) for 10 min, the supernatant was filtered through a 0.2-μm mesh membrane filter, and the filtrate was diluted with 1% HNO3.
Extraction of total Fe oxides
Total Fe oxides were extracted with a dithionite-sodium citrate reagent. A ground, air-dried soil sample (0.4 g, < 212 μm) and dithionite powder (0.4 g) were placed in a 50-mL polyethylene centrifuge tube, 20 mL of 0.75 mol L–1 sodium citrate solution was added, and the mixture was mechanically shaken at 25°C for 16 h. Ultrapure water (20 mL) and a drop of the polymeric flocculants were added, and the resulting mixture was manually shaken. After centrifugation at 3500 rpm (1700 g) for 10 min, the supernatant was filtered through a 0.2-μm mesh membrane filter, and the filtrate was diluted with 1% nitric acid. Fe concentration in the diluted solution was measured by ICP-OES. All extractions and measurements were carried out in triplicate. The experimental conditions were based on those of Blakemore et al. (Citation1987). Both short-range-ordered and crystalline Fe oxides should be extracted under these conditions. Therefore, in this study, we consider dithionite-citrate extractable Fe as total Fe oxides.
Experiment 2: Comparison of extraction selectivity for Mn and Fe oxides for some major methods for extracting heavy metals occluded in Mn and Fe oxides
Extraction with 0.5 mol L–1 NH2OH-HCl (pH 1.5)
An air-dried soil sample (1 g) was placed in a 50-mL polyethylene centrifuge tube, 40 mL of 0.5 mol L–1 NH2OH-HCl (pH 1.5) solution was added, and the mixture was mechanically shaken at 25°C for 16 h. After centrifugation at 3500 rpm (1700 g) for 10 min, the supernatant was filtered through a 0.2-μm mesh membrane filter, and the filtrate was diluted with 1% nitric acid. The pH of the extract was measured with a glass electrode pH meter, and the Mn and Fe concentrations in the diluted solution were determined by ICP-OES. All extractions and measurements were carried out in triplicate. The experimental conditions were based on those used for the step 2 fraction (reducible fraction) in the modified BCR method (Sahuquillo et al. Citation1999). Hereafter, this NH2OH-HCl extractant is designated HHmBCR.
Extraction with a mixture of acid oxalate and ascorbic acid
The extraction reagent is acid oxalate (0.1 mol L–1 oxalic acid and 0.175 mol L–1 ammonium oxalate) plus 0.2 mol L–1 ascorbic acid (hereafter Oxa-Asc). An air-dried soil sample (1 g) was placed in a 50-mL polyethylene centrifuge tube, and 30 mL of Oxa-Asc was added. The mixture was heated in boiling water for 1 h with occasional agitation and then cooled to approximately room temperature with running water for 10 min. After centrifugation at 3500 rpm (1700 g) for 10 min, the supernatant was filtered through a 0.2-μm mesh membrane filter, and the filtrate was diluted with 1% nitric acid. The Mn and Fe concentrations in the diluted solution were determined by ICP-OES. All extractions and measurements were carried out in triplicate. The experimental conditions were based on those reported by Shuman (Citation1985), but slightly modified as described by Sadamoto et al. (Citation1994). The modifications involved changes to the soil:solution ratio (from 1:50 to 1:30) and the extraction time (from 30 min to 1 h). The Oxa-Asc extraction method aims to extract both short-range-ordered and crystalline Mn and Fe oxides as well as the metals occluded in these oxides.
Calculation of the rates of extraction of Mn oxides and short-range ordered Fe oxides
We obtained the rates of extraction of Mn and Fe oxides for the acetate, HH-A, HHmBCR, and Oxa-Asc extractants as follows:
where MnEx and FeEx are the amounts of Mn and Fe extracted by the HH-A, HHmBCR and Oxa-Asc extractants. Mns is the amount of exchangeable and specifically adsorbed Mn extracted by the acetate solution, and Mno and Feo are the amounts of Mn and Fe extracted by oxalate. Fed is the amount of Fe extracted by dithionite-citrate. Exchangeable and specifically adsorbed metals do not form oxides, and Mns was likely extracted by the HH-A, HHmBCR, and Oxa-Asc extractants. Therefore, Mns is subtracted from Mn extracted by the HH-A, HHmBCR, and Oxa-Asc extractants. Because the amounts of exchangeable and specifically adsorbed Fe (Fes) were far less than the amounts of Fe extracted by the HH-A, HHmBCR and Oxa-Asc extractants, as well as much less than Feo and Fed, we calculated the rate of extraction of Fe oxides by simply using the values of Fe extracted by the HHmBCR and Oxa-Asc extractants.
RESULTS
Soil properties
Selected properties of the soil samples are listed in . The pH (H2O) values of the soil samples ranged from acidic to neutral (pH 3.80 to 7.06). Total carbon (TC) ranged from 10.4 to 296 g kg–1, and clay contents varied from 76.5 to 430 g kg–1. The amounts of Fed ranged from 13.7 to 55.5 g kg–1, and the amounts of Feo varied from 2.12 to 24.0 g kg–1. Dithionite-citrate extractable Mn (Mnd) was slightly higher than Mno for each sample. Alp and Fep were abundant in the non-allophanic Andisol samples than other soil types.
Table 2 Selected properties of soil samples
Experiment 1: Effects of increasing NH2OH-HCl concentration in NH2OH-HCl-HOAc extractant on the extractability of Mn and Fe oxides
Extraction of exchangeable and specifically adsorbed Mn and Fe
The amount of exchangeable and specifically adsorbed Mn was 4.66 × 10‒2 g kg–1 on average, ranging from 2.73 × 10‒3 to 0.243 g kg–1. The amount of exchangeable and specifically adsorbed Fe ranged from 1.98 × 10‒3 to 0.136 g kg–1, with an average value of 3.53 × 10‒2 g kg–1.
Extracted amounts and rates of extraction of Mn and Fe for the HH004-A, HH02-A, HH04-A, and HH06-A extraction methods
The amounts of Mn extracted with HH-A (MnHHA) were comparable to or larger than those for oxalate extraction (). MnHHA and rates of extraction of Mn oxides increased slightly with increasing concentration of NH2OH-HCl (). The rates of extraction of Mn oxides for the HH004-A, HH02-A, HH04-A, and HH06-A extraction methods from all samples averaged 117%, 130%, 136%, and 150%, respectively ().
Table 3 Amounts of manganese (Mn) extracted with hydroxylamine hydrochloride (NH2OH-HCl) in acetic acid (HOAc) extractants
Table 4 Rates of extraction of manganese (Mn) oxides with hydroxylamine hydrochloride (NH2OH-HCl) in acetic acid (HOAc) extractants
The amounts of Fe extracted with the HH-A method (FeHHA) were highly dependent on the NH2OH-HCl concentration (). The amounts of Fe extracted with the HH004-A, HH02-A, HH04-A, and HH06-A methods ranged from 4.69 to 20.9 g kg–1, 9.21 to 31.3 g kg–1, 11.9 to 37.0 g kg–1 and 16.9 to 46.4 g kg–1, respectively. For non-Andisol samples (except E-1 and E-3), the amounts of Fe extracted with HH004-A extraction were generally greater than those for oxalate extraction but less than those for dithionite-citrate extraction. For Andisol samples, the amounts of Fe extracted with HH004-A extraction were less than those with both oxalate extraction and dithionite-citrate extraction. Rates of extraction of short-range-ordered Fe oxides from the Entisols, Inceptisols, allophanic Andisols and non-allophanic Andisol samples with HH004-A extraction averaged 133%, 158%, 74.4% and 80.0% (). Rates of extraction of total Fe oxides for the Entisols, Inceptisols, allophanic Andisols and non-allophanic Andisol samples averaged 48.2%, 28.9%, 42.6% and 52.1%. Rates of extraction of both short-range-ordered Fe oxides and total Fe oxides increased with increasing NH2OH-HCl concentration. When the NH2OH-HCl concentration was 0.6 mol L–1, the rates of extraction of total Fe oxides from the Entisols, Inceptisols, allophanic Andisols and non-allophanic Andisol samples averaged 133%, 100%, 112% and 134%.
Table 5 Amounts of iron (Fe) extracted with hydroxylamine-acetic acid (NH2OH-HOAc) extractants
Table 6 Rates of extraction of iron (Fe) oxides with hydroxylamine hydrochloride (NH2OH-HCl) in acetic acid (HOAc) extractants
Thus, the amounts of Fe extracted with HH06-A extraction were comparable to those with dithionite-citrate extraction. The pH values of the extracts decreased with increasing NH2OH-HCl concentration (). Because pH values decreased during heated extraction (data not shown), the pH values after extraction were lower than before extraction in some cases. The average pH values after extraction were much higher for the allophanic Andisol samples than for the other soil types, and those for the non-allophanic Andisol samples were slightly higher than those for the Entisols and Inceptisols samples.
Experiment 2: Comparison of extraction selectivity for Mn and Fe oxides for some major methods for extracting occluded heavy metals
Amounts of Mn and Fe extracted with HHmBCR and Oxa-Asc
The amounts of Mn extracted with HHmBCR were comparable to those extracted with oxalate (). Oxa-Asc extracted smaller amounts of Mn than oxalate did from allophanic Andisol samples.
Table 7 Extracted amounts and rates of extraction of iron (Fe) and manganese (Mn) with 0.5 mol L–1 hydroxylamine hydrochloride (NH2OH-HCl) (pH 1.5) and with acid oxalate-ascorbic acid solution
The amounts of Fe extracted from all samples (except E-2), especially Andisol samples, were smaller with HHmBCR than with oxalate. Oxa-Asc extracted much greater amounts of Fe from non-Andisol samples than oxalate did; for some samples, the amount of Fe extracted was comparable to Fed. However, the amounts of Fe extracted from allophanic Andisol samples were comparable to Feo and much less than Fed. Oxa-Asc extracted smaller amounts of Fe from allophanic Andisol samples than from other soil samples, despite an abundance of Feo and Fed in the allophanic Andisol samples.
Amounts of Al and Si extracted with HH004, HH06, HHmBCR, and Oxa-Asc
The amounts of Al extracted from the soil samples with the four extractants followed the order Oxa-Asc > HH06-A > HH004-A ≥ HHmBCR (). The amounts of Al extracted from the Andisol samples were greater than those extracted from the non-Andisol samples. The order for Si was Oxa-Asc > HH06-A > HH004-A = HHmBCR (). Greater amounts of Si were extracted from the allophanic Andisol samples than from the other soil samples.
Figure 2 Amount of aluminum (Al) extracted from soil samples with different extraction methods. HHmBCR, HH004-A, HH06-A, and Oxa-Asc denote extraction with 0.5 mol L-1 hydroxylamine hydrochloride (NH2OH-HCl) (pH 1.5), 0.04 mol L-1 NH2OH-HCl in 25% acetic acid (HOAc), 0.6 mol L-1 NH2OH-HCl in 25% HOAc, and a mixture of acid oxalate and ascorbic acid, respectively. Bars represent means ± standard deviation (SD) (n = 3).
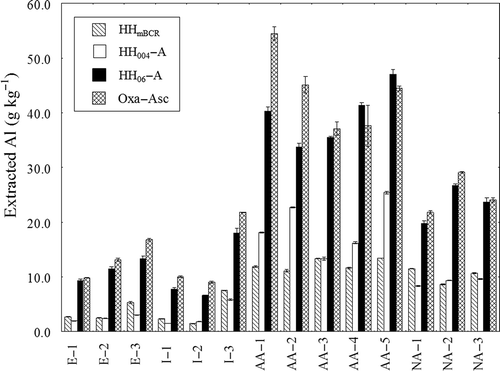
Figure 3 Amount of silicon (Si) extracted from soil samples with different extraction methods. HHmBCR, HH004-A, HH06-A, and Oxa-Asc denote extraction with 0.5 mol L-1 hydroxylamine hydrochloride (NH2OH-HCl) (pH 1.5), 0.04 mol L-1 NH2OH-HCl in 25% acetic acid (HOAc), 0.6 mol L-1 NH2OH-HCl in 25% HOAc, and a mixture of acid oxalate and ascorbic acid, respectively. Bars represent means ± standard deviation (SD) (n = 3).
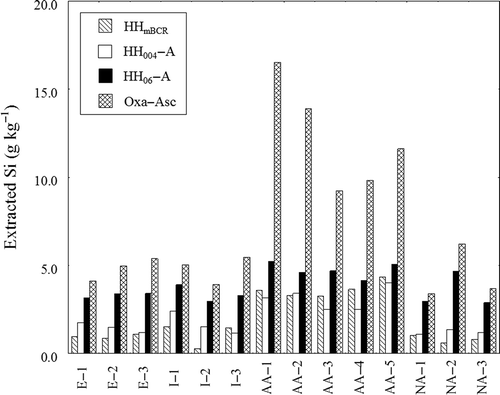
DISCUSSION
Relationships between extractability of Mn and Fe and NH2OH-HCl concentration
The amounts of Mn extracted with HH-A was comparable to or greater than those extracted with oxalate and gradually increased with increasing NH2OH-HCl concentration. Because Mn ions can be incorporated into crystalline Fe oxides (Ford et al. Citation1997), the small increase in the amounts of extracted Mn might be caused by the release of isomorphous-substituted Mn from the crystal lattice of these oxides. More Mn was extracted by HH06-A than even Mnd from most of the soil samples. This indicated that Mn was released from Fe oxides, such as coarse magnetite, which were relatively insoluble in dithionite-citrate. Most of the oxalate-extractable Mn is considered to form oxides. Therefore, we believe that the HH-A method extracted total Mn oxides from all soil samples used in this study.
For the non-Andisol samples, the amounts of Fe extracted with HH004-A were the same as or greater than those extracted with oxalate. Most of the oxalate-extractable Fe originates from short-range ordered Fe oxides (Parfitt and Childs Citation1988). Therefore, HH-A completely extracted short-range-ordered Fe oxides from the non-Andisol samples, assuming that short-range ordered Fe oxides were extracted prior to most of crystalline Fe oxides.
The smaller amounts of Fe were extracted from the Andisol samples with HH004-A than with oxalate ( and ). A substantial percentage of short-range ordered Fe oxide (allophanic samples: 13–40%, non-allophanic samples: 9–32%) was not extracted with HH004-A. Because volcanic ashes contain magnetite, and oxalate extracts part of the oxide, the amounts of short-range ordered Fe oxides in samples of slightly weathered (i.e. low Feo) volcanic ash soils might be overestimated (Shoji et al. Citation1987). However, in a preliminary experiment, we found that more Fe was extracted from synthesized magnetite (Kanto Chemical Co., Inc., Tokyo, Japan) with HH004-A than with oxalate. Therefore, we believe that, for our Andisol samples, some short-range ordered Fe oxides remained after extraction, with or without magnetite in the samples. La Force and Fendorf (Citation2000) reported that HH004-A extraction was insufficient for complete dissolution of crystalline Fe oxides in some polluted Fe-rich sediments. However, our study revealed that HH004-A extraction would not extract a relatively large percentage of short-range ordered Fe oxides from non-Fe-polluted allophanic Andisol samples. For non-allophanic Andisol samples, Feo and Fed may include a measurable amount of Fe extracted from Fe–humus complex, as indicated by the high content of Fep (). This additional amount of Fe possibly led to underestimation of the rates of extraction of short-range ordered and total Fe oxides of the non-allophanic Andisol samples.
Extraction of Fe oxides by NH2OH-HCl is highly dependent on extraction pH (Chao Citation1972). The pH values for the Andisol samples (allophanic samples: 2.43, non-allophanic samples: 2.15, on average) were higher than those for the non-Andisol samples (Entisols: 2.04, Inceptisols: 1.97, on average). Dissolution of abundant allophane-like minerals or amorphous Al oxides in allophanic Andisol samples might consume great amounts of protons during extraction. A decrease in proton concentration resulted in a decrease in the rates of both reduction and acid dissolution of Fe oxides. In addition, short-range ordered Fe oxides are more abundant in the Andisol samples than in the other soil samples. Therefore, larger amount of Fe oxides might result in a shortage of NH2OH-HCl as a reducing agent during extraction. Stoichiometrically, when proton consumption by reduction of Fe3+ is compensated by hydrolysis of NH2OH-HCl, 0.8 mmol of NH2OH-HCl (i.e., 20 mL of 0.04 mol L–1 solution) can reduce only 0.4 mmol (22.3 mg) of Fe3+. The same solution can reduce double the amounts (0.8 mmol, 44.7 mg) of Fe3+, assuming that all proton consumption is compensated by H+ from HOAc. The amount of NH2OH-HCl in the extractant was stoichiometrically sufficient to reduce all short-range-ordered Fe oxides. However, because 4.22 to 19.1 mg of Fe was extracted by 20 mL of 0.04 mol L–1 extractant, NH2OH-HCl concentration during extraction should be remarkably decreased. Stoichiometric calculation also indicates that Tessier’s condition (20 mL of 0.04 mol L‒1 NH2OH-HCl for 1 g of sample) cannot reduce all Fe oxides from relatively Fe-rich, non-polluted soil samples (e.g., AA-1 contains greater than 44.7 mg of Fed per gram of air-dried soil sample). Moreover, since hydroxylamine (NH2OH) itself reacts with O2 and is oxidized on heating, the net decrease in NH2OH-HCl during extraction would be greater than that calculated stoichiometrically. It might, however, be significantly important and not surprising that the original Tessier’s condition potentially has a severe shortage of NH2OH-HCl to extract Fe oxides from soil samples in Japan.
On the basis of the foregoing discussion, the concentration of NH2OH-HCl should be increased. Greater amounts of Fe were extracted with HH02-A, HH04-A, and HH06-A than with HH004-A (). Because NH2OH was oxidized on heating and HCl remained in solution, extraction pH decreased with increasing NH2OH-HCl concentration (). Therefore, this acceleration of Fe extraction was caused by the increase in both proton concentration and amount of reducing agent. On average, more Fe was extracted with HH02-A and HH04-A than with HH004-A, and less Fe was extracted with HH02-A and HH04-A than with dithionite-citrate. However, the amounts of Fe extracted with HH06-A were comparable to those extracted with dithionite-citrate. Therefore, most of the Fe oxides in the soil samples were extracted with HH06-A. The amount of Fe extracted with HH06-A was larger than that with dithionite-citrate. This indicates that HH06-A extracts even from hardly-soluble Fe phases, such as coarse particles of crystalline Fe oxides. Among our soil samples, AA-1 contained the largest amount of Fe oxides (). A dataset of 151 Andisol samples (24 soil profiles) studied by Kurobokudo Co-operative Research Group (Citation1986) indicates that about 85% of Andisol samples contained smaller amounts of Fe oxides than AA-1. Moreover, in general, Andisols (Andosols) contain much greater amounts of Fe than other soil types in Japan (Takeda et al. Citation2004). Hence, 0.6 mol L–1 NH2OH-HCl seems to be a sufficiently high concentration of NH2OH-HCl to extract almost all Fe oxides in most Japanese soils.
Lower pH is also favorable for suppressing re-adsorption of metals during extraction (Gómez-Ariza et al. Citation1999). However, lower pH may accelerate the extraction of non-target phases such as Al and Si minerals. Therefore, we discuss the extraction selectivity of the HH06-A method for Mn and Fe oxides in the next section.
Selectivities of HH004, HH06, HHmBCR, and Oxa-Asc extractions for Fe oxides
The amounts of Al and Si extracted with the four extractants followed the order Oxa-Asc > HH06-A > HH004-A ≥ HHmBCR for Al () and Oxa-Asc > HH06-A > HH004-A = HHmBCR for Si (). Oxa-Asc-extractable Al seems to include Al from the Al-humus complex, especially for the non-allophanic Andisol samples. For the other soil samples, much more Al was extracted with Oxa-Asc than with the other extractants, although Alp values were small. Hence, we concluded that Oxa-Asc is a more destructive extractant for the Al and Si minerals than the other extractants. Less Al and Si were extracted with HH06-A than with Oxa-Asc, and more Al and Si were extracted with HH06-A than with HH004 and HHmBCR.
Heavy metal concentrations in Mn and Fe oxides are much higher than those in bulk soils (McKenzie Citation1989; Sipos et al. Citation2011). Therefore, the concentrations of heavy metals in Al and Si minerals are probably much lower than those in Mn and Fe oxides. However, Al and Si minerals more or less contain heavy metals in their lattices (Kitagawa Citation1973; Churakov and Dähn Citation2012), and the amounts of the metals derived from these non-target phases would increase as their dissolution proceeds during extraction. Hence, extraction selectivity for heavy metals in Fe oxides over Al and Si minerals is important if extracted metals are defined as a “reducible” or “Mn and Fe oxide occluded” fraction. However, this point has not been studied in detail. Because it is difficult to determine the amounts of heavy metals extracted from Fe oxides or from Al and Si minerals, we used Al/Fe and Si/Fe ratios as indicators for extraction selectivity. Because the amounts of Mn oxides were much less than those of Fe oxides, and most of the Mn oxides were extracted with each extraction method, Mn is ignored in the following discussion.
As judged by the selectivities indicated by the Al/Fe and Si/Fe ratios, HH004-A and HH06-A were better extractants than HHmBCR and Oxa-Asc (). Because of its relatively strong acidity, HHmBCR extracts large amounts of Al. The results for the non-allophanic Andisol samples indicate that substantial amounts of Al were extracted from the Al-humus complex, although this extraction has been carried out before organically bound heavy metals are extracted in the modified BCR SEP. In contrast, HHmBCR extracted very small amounts of Fe from the Andisol samples, resulting in remarkably high Al/Fe ratios in these samples, being consistent with the results of Suda et al. (Citation2012). Oxa-Asc extracted large amounts of Fe except for the allophanic Andisol samples. Unexpectedly, Oxa-Asc extracted much smaller amounts of Fe than Fed from the allophanic Andisol samples. This result may be due to a lack of oxalate; however, more detailed studies might be needed. The Al/Fe and Si/Fe ratios for HH06-A extraction, as well as for HH004-A extraction, were lower than those for HHmBCR and Oxa-Asc extraction, especially for Andisol samples. Hence, we concluded that the selectivity of HH06-A extraction was comparable to that of Tessier’s HH004-A extraction and better than that of HHmBCR and Oxa-Asc extractions.
Table 8 Comparison of rates of extraction of iron (Fe) and manganese (Mn) oxides, and aluminum (Al)/Fe and silicon (Si)/Fe mole ratios, for four extraction methods
CONCLUSION
Tessier’s HH004-A extraction method might be unsuitable for allophanic Andisol samples because substantial amounts of short-range ordered Fe oxides are not extracted by this method. Higher extraction pH and relatively large amounts of short-range ordered Fe oxides might be responsible for incomplete extraction. For all soil samples in this study, a considerable amount of crystalline Fe oxides remained after HH004-A extraction. However, increasing the concentration of NH2OH-HCl increased the amounts of extracted Mn and Fe, and 0.6 mol L–1 of NH2OH-HCl was sufficient to extract almost all Mn and Fe oxides from the soil samples. The selectivity of the HH06-A method for Fe oxides over Al and Si minerals was comparable to or better than that of the original Tessier’s method (HH004-A). In contrast, the selectivities of the HHmBCR and Ox-Asc methods were much lower than those for the HH-A extractions (Tessier’s extraction, HH06-A extraction), especially when the HHmBCR method was applied to both allophanic and non-allophanic Andisol samples and when the Oxa-Asc method was applied to allophanic Andisol samples. Therefore, the HH06-A extraction method seems to be better than the HH004-A, HHmBCR, and Oxa-Asc extraction methods, especially for Japanese Andisol samples.
ACKNOWLEDGMENTS
We acknowledge Dr. S. Ono, Dr. T. Ito (Tohoku University), Dr. K. Kamewada, Dr. A. Aoki (Tochigi Prefecture Agricultural Experiment Station) and Dr. Y. Nagasaki (National Agricultural Research Center for the Western Region) for soil sampling. We also thank Dr. S. Wakabayashi (Agricultural Research Center) and Dr. J. Takahashi (University of Tsukuba) for providing some of the soil samples used in this study and for providing physicochemical data for some of the samples. We also thank Dr. Y. Maejima and Dr. I. Akahane (National Institute for Agro-Environmental Sciences) and Dr. K. Tamura (University of Tsukuba) for helpful comments. This work was supported by a Grant-in-Aid for Research Fellows of the Japan Society for the Promotion of Science (No. 23-444).
REFERENCES
- Benitez LN, Dubois JP 1999: Evaluation of ammonium oxalate for fractionating metallic trace elements in soils by sequential extraction. Int. J. Environ. Anal. Chem., 75, 261–273.
- Blakemore LC, Searle PL, Darly BK 1987: Extractable iron, aluminum and silicon. In Methods for Chemical Analysis of Soils, New Zealand Soil Bureau Scientific Report 10A, Ed. Blakemore L, pp. 71–76. Lower Hutt, DISR.
- Chao TT 1972: Selective dissolution of manganese oxides from soils and sediments with acidified hydroxylamine hydrochloride. Proc. Soil Sci. Soc. Am., 36, 764–768.
- Churakov SV, Dähn R 2012: Zinc adsorption on clays inferred from atomistic simulations and EXAFS spectroscopy. Environ. Sci. Tech., 46, 5713–5719.
- Ford RG, Bertsch PM, Farley KJ 1997: Changes in transition and heavy metal partitioning during hydrous iron oxide aging. Environ. Sci. Tech., 31, 2028–2033.
- Gee, GW, Bauder, JW 1986: Particle-size analysis. In Methods of Soil Analysis, Part 1, Physical and Mineralogical Methods, Ed. Klute A, pp. 383–411.American Society of Agronomy, Inc., Soil Science Society of America, Inc, Madison, WI.
- Gleyzes C, Tellier S, Astruc M 2002: Fractionation studies of trace elements in contaminated soils and sediments: A review of sequential extraction procedures. Trends Anal. Chem., 21, 451–467.
- Gómez-Ariza JL, Giráldez I, Sánchez-Rodas D, Morales E 1999: Optimization of a sequential extraction scheme for the characterization of heavy metal mobility in iron oxide rich sediments. Int. J. Environ. Anal. Chem., 75, 3–18.
- Harada Y, Inoko A 1977: The oxidation products formed from soil organic matter by hydrogen peroxide treatment. Soil Sci. Plant Nutr., 23, 513–521.
- Iu KL, Pulford ID, Duncan HJ 1982: Influence of soil waterlogging on subsequent plant growth and trace metal content. Plant Soil, 66, 423–427.
- Jarvis SC 1984: The forms of occurrence of manganese in some acidic soils. J. Soil Sci., 35, 421–429.
- Kitagawa Y 1973: Substitution of aluminum by iron in allophane. Clay Sci., 4, 151–154.
- Kurobokudo Co-operative Research Group 1986: Part II: Data base, In Ando Soils in Japan, Ed. Wada K, pp. 115–276. Kyushu University Press, Fukuoka.
- La Force MJ, Fendorf S 2000: Solid-phase iron characterization during common selective sequential extractions. Soil Sci. Soc. Am. J., 64, 1608–1615.
- Manh, PN, Ali Khan M, Jeon BH, Kim, JG, Lee G 2010: Stability of Fe- and Mn-(oxyhydr)oxides in common soil dispersion solutions. Water Air Soil Pollut., 217, 677–687.
- McBride MB 1982: Electron-spin resonance investigation of Mn2+ complexation in natural and synthetic organics. Soil Sci. Soc. Am. J., 46, 1137–1143.
- McKeague JA Day DH 1966: Dithionite- and oxalate-extractable Fe and Al as aids in differentiating various classes of soils. Can. J. Soil Sci., 46, 13–22.
- McKenzie RM 1989: Manganese oxides and hydroxides. In Minerals in Soil Environments, Eds. Dixon JB, Weed SB, pp. 439–465. Soil Science Society of America, Inc., Madison, WI.
- Miller WP, Martens DC, Zelazny LW 1986: Effect of sequence in extraction of trace metals from soils. Proc. Soil Sci. Soc. Am., 50, 598–601.
- Parfitt RL, Childs CW 1988: Estimation of forms of Fe and Al ‒ A review, and analysis of contracting soils by dissolution and Moessbauer methods. Aust. J. Soil Res., 26, 121–144.
- Sadamoto H, Iimura K, Honna T, Yamamoto S 1994: Examination of fractionation of heavy metals in soils. Jpn. J. Soc. Soil Sci. Plant Nutr., 65, 645–653.
- Sahuquillo A, Lopez-Sanchez JF, Rubio R, Rauret G, Thomas RP, Davidson CM, Ure AM 1999: Use of a certified reference material for extractable trace metals to assess sources of uncertainty in the BCR three-stage sequential extraction procedure. Anal. Chim. Acta., 382, 317–327.
- Salbu B, Krekling T, Oughton D.H 1998: Characterisation of radioactive particles in the environment. Analyst, 123, 843–849.
- Schuppli PA, Ross GJ, McKeague JA 1983: The effective removal of suspended materials from pyrophosphate extracts of soils from tropical and temperature regions. Soil Sci. Soc. Am. J., 47, 1026–1032.
- Shoji S, Itou T, Saigusa M 1987: Andisol-Entisol transition problem. Pedologist, 31, 171–175.
- Shuman LM, 1979: Zinc, manganese, and copper in soil fractions. Soil Sci., 127, 10–17.
- Shuman LM, 1985: Fractionation method for soil microelements. Soil. Sci., 140, 11–22.
- Sipos P, Nemeth T, May Z, Szalai Z 2011: Accumulation of trace elements in Fe-rich nodules in a neutral-slightly alkaline floodplain soil. Carpathian. J. Earth Environ. Sci., 6, 13–22.
- Soil Survey Staff 1998. Keys to Soil Taxonomy, 8th ed, National Resources Conservation Service, Washington, DC.
- Suda A, Makino T, Higashi T 2011: Applicability of selective dissolution of manganese oxide by acidified 0.1 M NH2OH-HCl in Japanese soils. Geoderma, 163, 291–295.
- Suda A, Makino T, Higashi T 2012: Extractability of manganese and iron oxides in typical Japanese soils by 0.5 mol L–1 hydroxylamine hydrochloride (pH 1.5). Soil Sci. Plant Nutr., 58, 684–695.
- Tack FMG, Van Ranst E, Lievens C, Vandenberghe RE 2006: Soil solution Cd, Cu and Zn concentrations as affected by short-time drying or wetting: The role of hydrous oxides of Fe and Mn. Geoderma, 137, 83–89.
- Takeda A, Kimura K, Yamasaki S 2004: Analysis of 57 elements in Japanese soils, with special reference to soil group and agricultural use. Geoderma, 119, 291–307.
- Tessier A, Campbell PGC, Bisson M 1979: Sequential extraction procedure for the speciation of particulate trace metals. Anal. Chem., 51, 844–851.
- The Japanese Society of Pedology 2003: Unified Soil Classification System of Japan, 2nd Approximation, The Fourth Committee for Soil Classification and Nomenclature, Hakuyu-sya, Tokyo (in Japanese).
- Wilcke W, Kretzschmar S, Bundt M, Saborío G, Zech W 1998: Aluminum and heavy metal partitioning in a horizons of soils in Costa Rican coffee plantations. Soil. Sci., 163, 463–471.