Abstract
The pattern of soil respiration and its responsible factors are still unclear in subalpine ecosystems. In this study, we used a static chamber system to measure soil respiration in a primary Abies fabri (Masters) Craib forest, a secondary A. fabri forest and a clear-cut land on Gongga Mountain, east of Tibetan Plateau in China. Our results showed there were substantial diurnal and temporal variations in these three subalpine ecosystems. The diurnal coefficient of variation (CV) of soil respiration was lowest in primary forest (20.5%), and highest in clear-cut land (40.4%). Diurnal patterns of soil respiration were generally associated with soil temperature at 5 cm for all the three systems. The temporal CV was lowest in secondary forest (55.6%), and highest in clear-cut land (82.8%). Soil temperature at 5 cm depth was the major factor controlling 55–61% of temporal variation of soil respiration. The temperature sensitivity (Q10 value) was higher in clear-cut land (Q10 = 6.7) than that in primary forest (3.7) and secondary forest (3.0), indicating that soil respiration in clear-cut land was more sensitive to temperature change. Annual soil respiration was 3330.2 g carbon dioxide (CO2) m−2 year−1, 2358.9 g CO2 m−2 year−1 and 4162.8 g CO2 m−2 year−1 for primary forest, secondary forest and clear-cut land, respectively. Our findings provided valuable information for understanding the effect of forest ecosystem change and land management on soil respiration in high-elevation subalpine ecosystems.
1. INTRODUCTION
Worldwide concerns over increasing atmospheric carbon dioxide (CO2) concentrations and its possible effects on the global climate have prompted extensive research on the global carbon (C) cycle. Soils hold about 1500 Pg of organic C in terrestrial ecosystems . Soil respiration (RS), which follows gross primary production (GPP) as the second largest C flux in the global terrestrial C cycle (Raich and Schlesinger Citation1992), is a critical ecosystem process that regulates C cycle in the earth system. It is estimated that a global flux of CO2 emission through RS accounts for 68–75 Pg CO2-C year−1 (Mosier Citation1998); thus, even a small change in the RS rate would have a profound impact on the atmospheric CO2 concentration (Rustad et al. Citation2000; Chen et al. Citation2010). Therefore, detailed information on RS is critical for understanding ecosystem-atmosphere C exchange. Although globally important, RS is not well characterized spatially or seasonally for most ecosystems (Raich and Tufekcioglu Citation2000; Hu et al. Citation2001; Takahashi et al. Citation2011).
Over the past few decades, high-altitude soil has attracted more attention in the debate on the potential impact of environmental change on the global C cycle. The Tibetan Plateau (> 2.5 million km2) is the youngest and highest plateau in the world. There is increasing interest in its C cycle because of the region’s unique climate and its apparently sensitive responses to climate change (Cao et al. Citation2004). It is estimated that soils on the Tibetan Plateau contain large amounts of soil organic matter (SOM), and the magnitude of warming on the Tibetan Plateau is projected to be larger relative to many other regions (Jiang et al. Citation2009; Xu et al. Citation2010). Therefore, CO2 emission from soils in this region could be more pronounced than in other ecosystems. RS was studied over many ecosystems, including temperate forest (Ruehr and Buchmann Citation2010), boreal forest (Laganière et al. Citation2012), cropland (Huang et al. Citation2012), plantation (Wu et al. Citation2011), etc. However, there are few data on RS and its controlling factors on the plateau, especially for subalpine ecosystems on the plateau.
Gongga Mountain is an eco-environmentally vulnerable region, located at the east side of the Tibetan Plateau. Land use/cover changes happen regularly in this region due to frequent anthropogenic (e.g., forest harvest or clear-cut of forest) and natural (e.g., debris flow, landslide) disturbances. Most of the land originally covered with primary forests in this region has been degraded by human activities during the past several centuries (Sun et al. Citation2012). In extreme cases, the land has become very degraded, with barely any vegetation cover. Some destroyed primary forests (anthropogenically or naturally driven) may recover to secondary forests in several years if there is no further disturbance, while some were converted to agricultural land or other land systems (e.g. road, building land). Forest soil is an important source or sink of CO2 in atmosphere, and this “source/sink” role is generally associated with changes in forest type, forest age and forest management (Jackson et al. Citation2000b; Jandl et al. Citation2007). However, little information can be found about the effect of forest ecosystem change and land management on RS in Tibetan Plateau, which will limit our understanding on RS in this region.
Hence, in this study, we conducted research in three adjacent subalpine systems on Gongga Mountain, including a primary forest, a secondary forest and clear-cut land. The main objectives of this study were as follows: (1) to quantify diurnal and temporal RS and their variability in primary forest, secondary forest and clear-cut land, (2) to examine the factors affecting variation of RS in the three subalpine systems and (3) to examine the effect of forest ecosystem change and land management on RS on Tibetan Plateau.
2. MATERIALS AND METHODS
2.1. Site description
Gongga Mountain (29o20′–30o30′ N, 101o30′–102o15′ E), located in the eastern fringe of the Tibetan Plateau, is a region characterized by varied topography, geology and climate (a). It is the highest of the Hengduan Mountains, elevation 7556 m above sea level (asl). Our study was conducted in Hailuogou Glacier and Forest National Park, on the eastern slope of Gongga Mountain, between 3000 and 3100 m asl (b). The Hailuogou Glacier, which is a typical monsoonal temperate glacier, is located in this region (more information is given by He et al. Citation2008 and Luo et al. Citation2013). The zonal vegetation is Abies fabri (Masters) Craib forest, which is the main component of the vertical vegetation spectrum on the eastern slope of Gongga Mountain. The zonal soil is classified as Alfisols based on United States Department of Agriculture (USDA) Soil Taxonomy, which contains a high level of humus, nitrogen (N) and rocks, with a high porosity. The regional climate is dominated by the southeastern Pacific monsoon. The mean annual air temperature is 5.3°C, the minimum mean air temperature is –4.3°C in January, and the maximum mean air temperature is 11.9°C in July. The total annual precipitation is about 1900 mm, most of which occurs from June to September. The length of the growing season is approximately 7 months, from early April to middle October. Snowfall usually occurs from mid-November to early March. On shady slopes, the depth of snow cover could reach to about 15 cm, and remain for a long time. On sunny slopes, snow retention time is usually 2 or 3 d. Daily air temperature and rainfall during the study period (2008.6–2009.5) are presented in .
Figure 1 (a) Location of Gongga Mountain, (b) map of eastern slope of Gongga Mountain and (c) location of study sites.
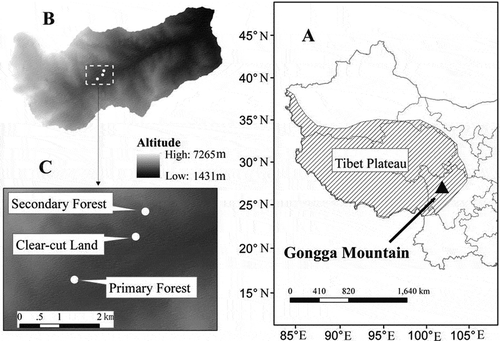
Figure 2 Mean monthly air temperature (°C) and mean monthly precipitation (mm) during the study period. Data were collected at the Meteorological station of Gongga Mountain, China.
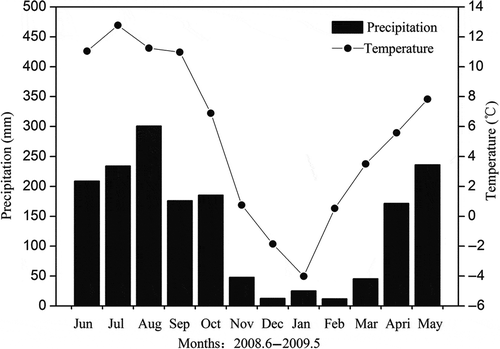
This study covered the following three subalpine systems: primary forest (Site 1), secondary forest (Site 2), and clear-cut land (Site 3) (c). Site 1 is a mature stand of A. fabri (> 200 years). Dominant understory species are Betula utilis D. Don, Acer maximowiczii Pax and Sorbus pohuashanensis (Hance) Hedl, etc. Mean tree density in Site 1 was about 211 trees ha−1. Mean diameter at breast height (DBH) and tree height were 50.8 cm and 31.9 m, respectively. Soil at Site 1 is classified as Alfisols, which has a high sand content and strong permeability, with a thick organic horizon (about 28 cm on average). The soil texture is forest loamy sand.
Strong debris flows, which could destroy original soil and vegetation, occur frequently on the eastern slope of Gongga Mountain. Secondary forest succession can take place on slash land after debris flow in this region, with a chronosequence of development from herbosa (Leontopodium longifolium Ling, Cacalia davidii (Franch.) Hand-Mazz., Fragaria orientalis Losina-Losinsk, etc.) to the climax community dominated by A. fabri. Site 2 is a secondary succession forest developed from the slash land after debris flow and regenerated naturally for about 60 years since 1948. It had a closed canopy dominated by A. fabri, Populus purdomii Rehder, A. maximowiczii and B. utilis, with dense shrubs in the undergrowth during the study period. Mean tree density was about 1708 trees ha−1. Mean DBH and height were 19.6 cm and 15.3 m, respectively. Soil at Site 2 is classified as Entisols originating from debris-flow slash. The soil texture is forest sandy loam, with higher sand content and a thinner organic horizon (about 7 cm on average) compared with Site 1.
In past decades, many forests were destroyed for the construction of tourist facilities (roads, hotels, cableway, etc.) or converted to farmland in Gongga Mountain. Site 3 is located in the meteorological observation field of the Alpine Ecosystem Observation and Experiment Station of Gongga Mountain. It used to be a secondary A. fabri forest similar to Site 2, but it was clear cut (removal of trees and shrubs) and reserved for about 10 years since 1998. Soil in Site 3 was well reserved, so pioneer plants occupied the land quickly after the clear cutting. The main species now are herb plants including Astragalus adsurgens Pall., Anaphalis aureopunctata Lingelsh. et Borza and Epilobium hirsutum Linn., etc. Taller plants may be unfavourable for meteorological observation, so they would receive a harvest in a year, littering the aboveground parts on the ground surface. The ecosystem in Site 3 remained stable in general, for the harvest only inclued a small portion of the taller plants, and didn’t disturb (or only slightly disturbed) the soil in Site 3. So, in this paper, we defined Site 3 as a clear-cut land formed in 1998, and tried to examine the clear-cut effect of RS. The soil at Site 3 was also Entisols, similar to the soil at Site 2.
2.2. Soil respiration, soil temperature and soil moisture measurements
Three 10 m × 10 m plots were randomly located within each site. RS was measured using a soil chamber connected to a portable infrared gas analyzer (LI-6400-09, Li-Cor, Inc., Lincoln, NE, USA). To minimize soil surface disturbances, the chamber was mounted on polyvinyl chloride (PVC) soil collars inserted into the soil to a depth of 2 cm. Three PVC collars were randomly placed in each plot 1 d before the measurements. The plants in the collars were cut at ground level and litter in the collars was removed. A measurement consisted of placing the chamber on the soil collar, scrubbing the CO2 to sub-ambient levels, and determining soil CO2 efflux over several 5-s periods. Data were recorded at 5-s intervals by the datalogger in the LI-Cor 6400 console. Each measurement usually took 1–3 min after placing the chamber on the collar. Concurrent measurements were made on the same day on each occasion at all three sites. The measurements of RS were conducted three times between 08:00 and 14:00 h at a frequency of approximately every 10 d from June 2008 to May 2009. A 24-hourly respiration was measured in July 2008 for each site.
During the experimental period, soil temperature (ST) was monitored concurrently with RS using a copper/constantan thermocouple penetration probe (LI-6400-09 TC, LI-Cor) inserted into the soil to a depth of 5 cm in the vicinity of the RS chamber collars. The soil moisture (SM) content in the topsoil layer (0–20 cm) was measured gravimetrically and simultaneously with RS (Jackson et al. Citation2000a).
2.3. Soil sampling and analysis
Three soil cores for chemical analyses, 20 cm in depth, were collected randomly from each plot on each month during the study period. The soil samples were sieved to remove all visible plant material and air-dried for measurements of soil C and N concentration and pH value. Soil organic carbon (SOC) was determined using wet combustion as described by Nelson and Sommers (Nelson and Sommers Citation1982), soil total nitrogen (total N) was measured by semimicro-Kjedahl method (Bremner Citation1996), soil pH was measured with a potentiometer pH meter. Mean values were used for the later investigation ().
Table 1 Main properties of topsoil in primary forest, secondary forest and clear-cut land on Gongga Mountain, China
2.4. Estimation of daily average soil respiration
Although daily average RS can be accurately calculated from continuous diurnal RS measurements, it is time-consuming work and unsuitable for long-term seasonal and between-year variation measurement (Shi et al. Citation2006). Our solution to these problems was to use daytime measurement during a specific period for daily estimation (Mielnick and Dugas Citation2000). We selected nine pieces of 24-hourly RS data, extracted from the measurements made from 1997 to 2007, to analyze the suitability of our selected daytime average RS to represent the daily RS. The daytime average was calculated from three measurements between 08:00 and 14:00, and the diurnal average was calculated from 12 measurements in a day. Although the data showed apparent diurnal variation in the growing season, especially for July, the daytime and diurnal averages were similar for the nine 24-h RS measurements at all three sites (), allowing us to use the average of RS measurements between 08:00 and 14:00 to provide a reasonable estimate of daily value in this study.
2.5. Statistical analysis
Based on the measured data, an exponential function was established to describe the relationship between soil respiration and soil temperature:
where RS is soil respiration, ST is soil temperature, and α and β are constant coefficients. The temperature sensitivity (Q10) of soil respiration was calculated as:
Linear regression was performed to examine the relationship between RS and SM. In order to explore the concordant effects of ST and SM on RS, the following model was used:
where a, b, and c are constants.
To quantify diurnal and seasonal variability in RS, the coefficient of variation (CV) was calculated as:
The effects of land ecosystems and sampling dates on RS were tested using a two-way analysis of variance (ANOVA). All statistical analyses were conducted using SAS 9.0.
3. RESULTS
3.1. Soil properties
Site differences in topsoil properties were shown in . ST at 5 cm did not differ significantly among the three sites, but the annual average ST was lowest in primary forest (ST = 5.5°C), due to its dense shrub coverage, and highest in clear-cut land (ST = 8.9°C), owing to its lower aboveground shade. SM differed significantly among the three sites, with the highest value in primary forest (SM = 23.9%) and lowest value in secondary forest (SM = 15.8%). Both SOC and total N content in the top 20 cm of soil were recorded at the maximum in primary forest and minimum in clear-cut land. In contrast, the highest values of pH and bulk density could be detected in clear-cut land, while the lowest values could be found in primary forest (see details in ).
3.2. Diurnal variation of soil respiration
In the Gongga Mountain area, July is the typical month of the growing seasons when both the temperature and precipitation are high. Twenty-four-hourly RS and ST at 5 cm depth for the three sites in July 2008 are shown in . The diurnal CV of RS was 20.5%, 36.0% and 40.4% for primary forest, secondary forest and clear-cut land, respectively.
Figure 4 Diurnal variation of soil respiration and soil temperature at 5 cm depth for primary forest, secondary forest and clear-cut land on Gongga Mountain, China.
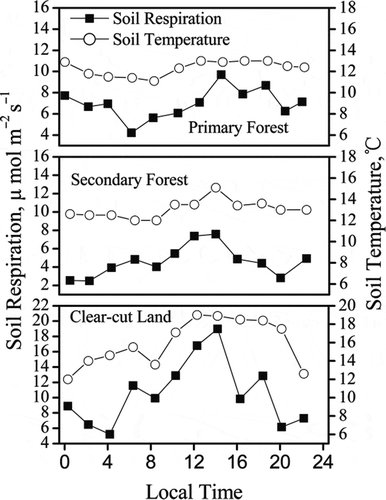
The maximum value of diurnal RS was observed between 14:00 and 16:00, and the minimum value was found between 02:00 and 06:00 for all three sites, although the diurnal variation patterns weren’t similar among the three sites. Diurnal variations of RS were generally associated with variations of ST at 5 cm depth for all three sites. The coefficient of correlation between 24 h RS and ST was 0.74, 0.68 and 0.64 for primary forest, secondary forest and clear-cut land, respectively.
3.3. Temporal variation of soil respiration, soil temperature and soil moisture
By assuming that daytime average RS (08:00–14:00) was a reasonable estimate of the daily average, we calculated the monthly values of RS as mean RS on the 3 observation days each month. As is shown in , similar temporal variations in RS were observed for all three subalpine ecosystems. RS was higher in the growing season than than in the non-growing season. The lowest monthly RS was 0.67 μ mol m−2 s−1 occurring at the secondary forest in February. By contrast, monthly RS rate peaked in July or August in the three sites, with the maximum rate of 8.5 μ mol m−2 s−1 for clear-cut land in August. The cumulative RS in July and August accounted for 34.4%, 33.6% and 45.8% of the total annual emissions in primary forest, secondary forest and clear-cut land, respectively. In contrast, the cumulative RS in the coldest months, December, January and February, accounted for only 12.2%, 11.6% and 9.3% of the total annual emissions in primary forest, secondary forest and clear-cut land, respectively. The temporal coefficient of variation (CV) of RS was 57.0%, 55.6% and 82.8% for primary forest, secondary forest and clear-cut land, respectively.
Figure 5 Temporal dynamics of soil respiration, soil temperature and soil moisture for primary forest, secondary forest and clear-cut land on Gongga Mountain, China.
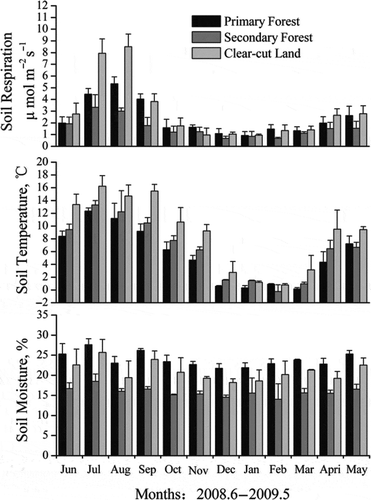
The pattern of temporal variation for ST from each site was similar to that of RS (). The temporal CV value was 43.3%, 46.2% and 56.0% for primary forest, secondary forest and clear-cut land, respectively. The temporal variation of SM at the depth of 0–20 cm fluctuated relative gently compared to that of RS and ST (). The CV value was 9.4%, 9.2% and 15.8% for primary forest, secondary forest and clear-cut land, respectively.
3.4. Annual soil respiration of three subalpine ecosystems
Significant differences in RS were observed when the data set was analyzed using a two-way ANOVA (). The total annual RS increased significantly with the change from forest land to managed land. Annual RS was significantly larger for clear-cut land (4162.8 g CO2 m−2 year−1, p < 0.05) than that in primary forest (3330.2 g CO2 m−2 year−1, p < 0.05) and secondary forest (2358.9 g CO2 m−2 year−1, p < 0.05). And, also, annual RS in primary forest was significantly higher than that in secondary forest.
Table 2 Two-way analysis of variance (ANOVA) of soil respiration by site and measuring date
ANOVA also revealed a significant measuring date effect on RS (). The result confirmed the substantial temporal variation in RS.
3.5. Temporal soil respiration related to soil temperature and soil moisture
The temporal RS experienced a positive exponential correlation with ST for all sites (), with the highest R2 coefficient in primary forest and the lowest in clear-cut land (). The Q10 value against ST at 5 cm was 3.7, 3.0 and 6.7 for primary forest, secondary forest and clear-cut land, respectively, indicating the different response of RS to ST among the different subalpine ecosystems. Notably, the Q10 value was significantly higher in clear-cut land than that in primary forest and secondary forest.
Figure 6 Relationship between soil respiration and soil temperature at 5 cm depth for primary forest, secondary forest and clear-cut land on Gongga Mountain, China.
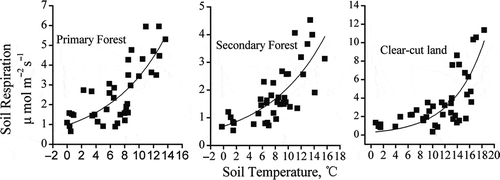
Table 3 Values of regression coefficient and R2 in models for relationships between soil respiration (RS) and soil temperature (ST), soil moisture (SM), and ST-SM
Significantly linear correlation of temporal RS with SM could be found in all three sites (). The SM-based model could explain 16–41% of temporal variation in RS for the three ecosystems (), but the correlation of temporal RS with SM was relatively weaker than that with ST. Furthermore, compared to ST-based model, the model integrating RS against ST and SM could explain another 13% temporal variation of RS in secondary forest, but did not increase R2 significantly in primary forest and clear-cut land ().
4. DISCUSSION
4.1. Diurnal variation of soil respiration
RS has been studied intensely across ecosystems in the world, while the findings on diurnal variation are sometimes controversial. Yan et al. (Citation2009) reported that there were no significantly differences in Rs between daytime and nighttime in Dinghushan area in China, although ST varied significantly through the day in different forest types and all seasons. Betson et al. (Citation2007) also found no diurnal patterns in RS rate in a boreal Picea abies L. Karst. forest in northern Sweden, despite substantial variations in climatic conditions and shoot photographic rates. However, Shi et al. (Citation2006) and Hirano (Citation2005) found there were substantial diurnal variations in a cropland ecosystem on the Tibetan Plateau and a temperate deciduous forest. However, few studies on diurnal variation for RS in subalpine ecosystems have been reported. In this study, we found district diurnal variations in three different subalpine systems on Gongga Mountain. Diurnal patterns of RS were generally associated with ST at 5 cm for all the three systems (primary forest: r = 0.74, secondary forest: r = 0.68, clear-cut land: r = 0.64). Diurnal variation was highest in clear-cut land and lowest in primary forest. Tree canopy and dense shrub in primary and secondary A. fabri forest can reduce sensitivity of ST to the diurnal changes in air temperature, while in clear-cut land, ST was more sensitive to air temperature fluctuation because of the lower aboveground shade. These facts may cause the greater diurnal variation of RS in clear-cut land than that in primary and secondary forest. What’s more, the differences in the content of SOC, the quality of SOM and biodiversity in different sites may also produce different diurnal patterns of RS (Wu et al. Citation2003).
4.2. Effects of soil temperature and soil moisture on soil respiration
Temporal variation of RS was obvious in all three sites in our study, which is consistent with other studies on RS in different ecosystems with distinguished temporal climatic characteristics (Irvine and Law Citation2002; Campos Citation2006; Iqbal et al. Citation2008; Liu et al. Citation2008). Temporal variation in RS was generally attributed to changes in ST (Shi et al. Citation2006) or SM (Davidson et al. Citation1998; Xu and Qi Citation2001) alone or together (Chen et al. Citation2010; Liu et al. Citation2011) at the ecosystem level.
Temperature can influence soil respiration mainly by affecting root activities, decomposition of plant litter and SOM and plant biomass production, leading to changes in C substrate availability for plant roots and soil microbes (Chen et al. Citation2010; Luo et al. Citation2012). The high exponential positive correlation of RS with ST reported in this study indicates that RS was strongly influenced by seasonal temperature change for all three subalpine ecosystems. Similar findings have been reported in numerous previous studies on different ecosystems (Raich and Schlesinger Citation1992; Wang et al. Citation2008; Yan et al. Citation2009). The magnitude of temporal variation in RS due to ST varied from 55% at clear-cut land to 61% at primary forest (). The differences in the temperature controls over RS may be caused by the changes in plant growth and soil microbial activities, which may also contribute to temporal variation in RS (Chen et al. Citation2010).
Q10 was used to describe ST dependence of SR. The Q10 value of RS varied from 3.0 to 6.7 across different sites, with an average of 4.5 which was higher than the global median value of 2.4 (Raich and Schlesinger Citation1992), indicating a general tendency towards higher Q10 in colder ecosystems than in warmer ecosystems. The higher Q10 value of RS in clear-cut land than that in forests (primary and secondary A. fabri forest) demonstrated that the deforestation may enhance the temperature sensitivity of RS. The Q10 value could be affected by many factors such as calculation model, ST, SM, substrate quality and microbial population (Davidson et al. Citation2006), while the mechanisms mediating the variability of Q10 value with land ecosystems are still unclear (Sheng et al. Citation2010). In this study, the difference of Q10 value between primary forest and secondary forest may result from the differences in the root respiration, SOC quality or microbial activities. The enhanced Q10 value in clear-cut land may primarily result from the human disturbance which could promote the decomposition of SOM and enhance the microbial activity, thus increasing the temperature sensitivity of RS.
Apart from ST, SM is also recognized as the main factor controlling the temporal variability of SR (Davidson et al. Citation1998; Saiz et al. Citation2006b). The relationship between SR and SM is complex, and both linear and nonlinear relationships have been reported (Saiz et al. Citation2006a; Yan et al. Citation2006; Chen et al. Citation2010). In this study, although there was a significant linear relationship between RS and SM, the relationship was not as strong as that between RS and ST. The combined use of ST and SM functions did not improve our capability to better explain SR compared with the model based on ST only, thus indicating that ST exerts a stronger control than SM on SR in our study. Similar results were reported in previous studies under various ecosystems (Iqbal et al. Citation2008; Liu et al. Citation2011). It was suggested that SM has an insignificant effect on upland RS, unless the area experiences frequent prolonged drought (Liu et al. Citation2011). In our study area, the atmospheric humidity and SM content were high because of the frequent precipitation (Luo et al. Citation2012). The temporal variation in SM for each site was small (CV from 9.2% to 15.8%). Thus, SM is less important in influencing temporal variation of RS, in contrast with ST.
4.3. Comparison of soil respiration among the three sites
Our results showed that annual RS in primary forest was 41.2% larger than that in secondary forest, and RS in clear-cut land was 25.0% and 76.5% larger than that in primary and secondary forest, respectively. RS is the sum of autotrophic respiration and heterotrophic respiration. As forest develops from young stand to old stand, root biomass, microbial biomass, SOC and forest floor mass tend to increase with this process, thus possibly leading a higher autotrophic respiration or heterotrophic respiration in old forest than that in young forest (Wang et al. Citation2010). In this study, we investigated SOC and total N in primary forest and secondary forest. As RS involves the process of converting organic C into inorganic C, the RS rate is ultimately determined by the supply of C substrate. Total N content could affect RS by affecting soil N availability for plant growth and root activities. Thus, the higher SOC and soil total N in primary forest may induce higher heterotrophic respiration and autotrophic respiration than secondary forest.
Forest management activities such as clear-cutting represent a major disturbance and change in soil C dynamics, thus the change of soil CO2 efflux following clear-cutting has received considerable attention (Xu and Qi Citation2001; Kim Citation2008). However, conflicting results have been reported for the effects of clear-cutting on RS, including increase (Laporte et al. Citation2003; Kim Citation2008), decrease (Ponder Citation2005) and no discernible effect (Davidson et al. Citation1998). In this study, we can detect a significantly positive effect of clear-cutting on RS 10 years after the forest was harvested. Our study showed clear-cut land had much lower SOC content than the other two subalpine ecosystems and, therefore, we expected that it had much less annual RS. However, in this study, clear-cut harvesting has a significant increase effect on RS. The reason may be that clear-cut land could provide a favorable environment, such as the increased ST and soil pH (), for microbial or root respiration and organic matter decomposition compared with mature and secondary forest. For example, the roots retained in soil and the aboveground parts of plants littered on the ground surface would provide organic matter for microbial respiration, and the significantly lower content of SOC in clear-cut land than that in primary or secondary forest indicated an accelerated turnover of soil C pool by increased ST after canopy removal (a). Mean soil pH was highest in clear-cut land (pH 6.8) than in primary forest (pH 4.1) and secondary forest (pH 6.5). Soil pH is an important variable affecting soil respiration because it is closely related to microbial biomass activity and nutrient availability. The increase of pH in clear-cut land may be due to the release of basic cations (potassium, calcium, magnesium, etc.) in connection with decomposition of slash and humus, and reduced cation uptake by trees (Bååth et al. Citation1995). As discussed above, the conditions for the decomposition of organic matter could improve in clear-cut land after canopy removal, thus possibly enhancing RS in clear-cut land compared with the other two subalpine ecosystems (b).
Figure 8 (a) Relationship between soil temperature (ST) and soil organic carbon (SOC); (b) relationship between annual soil respiration (RS) and SOC. CO2, carbon dioxide.
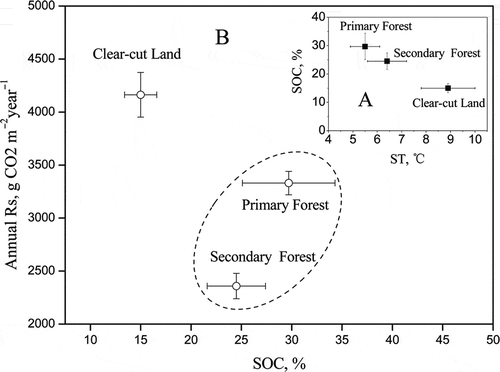
The annual RS from the three subalpine ecosystems ranged from 2359 to 4162 g CO2 m−2 year−1. The annual RS emission for coniferous forest reported here is comparable to that in the review of annual RS across vegetation types and biomes made by Bahn et al. (Citation2010), while higher than that in the review of global RS rates made by Raich and Schlesinger (Citation1992) when concerning ST. Several reasons could contribute to the difference between this study and the review made by Raich and Schlesinger. The method employed to measure RS in Raich’s article was mostly static chambers or the alkali absorption method. These methods are known to underestimate RS, particularly when effluxes are large (Rayment and Jarvis Citation2000), and as a result their extrapolated annual emission is also likely to be an underestimate. Besides, Hailuogou Glacier, a typical monsoonal temperate glacier, could modulate regional climate and hydrological regime, which may be the reason for the long growing season (approximately 7 months) for coniferous forest in our study area. It is reported that a longer growing season can enhance the productivity and respiration of ecosystems (Myneni et al. Citation1997; Euskirchen et al. Citation2006). The larger RS reported here may be partly related to the larger autotrophic respiration which is due to the long growing season in this region.
5. CONCLUSION
RS was investigated in three different subalpine ecosystems (primary A. fabri forest, secondary A. fabri forest and clear-cut land) on the Eastern Tibetan Plateau, China. Diurnal variations of RS were generally associated with variations of ST at 5 cm depth for all three subalpine ecosystems, with the maximum value at 14:00–16:00 and the minimum value at 02:00–06:00. RS showed similar temporal variation, being higher in the growing season and lower in the non-growing season. Significantly positive correlation can be detected between RS and ST as well as SM (p < 0.05), but ST was the main controlling factor of temporal variation of RS in this region. The Q10 value was higher in clear-cut land than that in primary and secondary forest, indicating that RS in clear-cut land was more sensitive to temperature change. Annual RS was highest in clear-cut land and lowest in secondary forest. Our findings provided valuable information for understanding the effect of forest ecosystem change and land management on soil respiration in high-elevation subalpine ecosystems.
ACKNOWLEDGMENTS
The present work received financial support from the Knowledge Innovation Project of the Chinese Academy of Science (Grant KZCX2-EW-309) and the National Natural Science Foundation of China (Grants 41272200 and 40871042).
REFERENCES
- Bååth E, Frostegård Å, Pennanen T, Fritze H 1995: Microbial community structure and pH response in relation to soil organic matter quality in wood-ash fertilized, clear-cut or burned coniferous forest soils. Soil Biol. Biochem., 27, 229–240.
- Bahn M, Reichstein M, Davidson EA et al. 2010: Soil respiration at mean annual temperature predicts annual total across vegetation types and biomes. Biogeosciences (Online), 7, 2147.
- Betson NR, Gottlicher SG, Hall M, Wallin G, Richter A, Hogberg P 2007: No diurnal variation in rate or carbon isotope composition of soil respiration in a boreal forest. Tree Physiol., 27, 749–756.
- Bremner DJ 1996: Nitrogen: total. In Method of Soil Analysis, Ed. Sparks DL et al., pp. 539–579. American Society of Agronomy, Madison, WI.
- Campos CA 2006: Response of soil surface CO2-C flux to land use changes in a tropical cloud forest (Mexico). For. Ecol. Manage., 234, 305–312.
- Cao GM, Tang YH, Mo WH, Wang YA, Li YN, Zhao XQ 2004: Grazing intensity alters soil respiration in an alpine meadow on the Tibetan plateau. Soil Biol. Biochem., 36, 237–243.
- Chen Q, Wang Q, Han X, Wan S, Li L 2010: Temporal and spatial variability and controls of soil respiration in a temperate steppe in northern China. Glob. Biogeochem. Cy., doi: 10.1029/2009gb003538.
- Davidson EA, Belk E, Boone RD 1998: Soil water content and temperature as independent or confounded factors controlling soil respiration in a temperate mixed hardwood forest. Glob. Change Biol., 4, 217–227.
- Davidson EA, Janssens IA, Luo YQ 2006: On the variability of respiration in terrestrial ecosystems: moving beyond Q(10). Glob. Change Biol., 12, 154–164.
- Euskirchen E, McGuire AD, Kicklighter DW et al. 2006: Importance of recent shifts in soil thermal dynamics on growing season length, productivity, and carbon sequestration in terrestrial high‐latitude ecosystems. Glob. Change Biol., 12, 731–750.
- He Y, Li Z, Yang X et al. 2008: Changes of the Hailuogou Glacier, Mt. Gongga, China, against the background of global warming in the last several decades. J. China Univ. Geosci., 19, 271–281.
- Hirano T 2005: Seasonal and diurnal variations in topsoil and subsoil respiration under snowpack in a temperate deciduous forest. Glob. Biogeochem. Cy., doi: 10.1029/2004gb002259
- Hu RG, Kusa K, Hatano R 2001: Soil respiration and methane flux in adjacent forest, grassland, and cornfield soils in Hokkaido, Japan. Soil Sci. Plant Nutr., 47, 621–627.
- Huang N, Niu Z, Zhan Y et al. 2012: Relationships between soil respiration and photosynthesis-related spectral vegetation indices in two cropland ecosystems. Agric. For. Meteorol., 160, 80–89.
- Iqbal J, Hu R, Du L et al. 2008: Differences in soil CO2 flux between different land use types in mid-subtropical China. Soil Biol. Biochem., 40, 2324–2333.
- Irvine J, Law BE 2002: Contrasting soil respiration in young and old-growth ponderosa pine forests. Glob. Change Biol., 8, 1183–1194.
- Jackson RB, Anderson LJ, Pockman WT 2000a: Measuring water avail ability and uptake in ecosystem studies. In Methods in Ecosystem Science, Ed. Sala OE et al., pp. 199–214. Springer, New York.
- Jackson RB, Schenk H, Jobbagy E et al. 2000b: Belowground consequences of vegetation change and their treatment in models. Ecol. Appl., 10, 470–483.
- Jandl R, Lindner M, Vesterdal L et al. 2007: How strongly can forest management influence soil carbon sequestration? Geoderma, 137, 253–268.
- Jiang F, Sun H, Lin B, Liu Q 2009: Dynamic changes of topsoil organic carbon in subalpine spruce plantation at different succession stages in western Sichuan Province (In Chinese). J. Appl. Ecol., 20, 2581.
- Kim C 2008: Soil CO(2) efflux in clear-cut and uncut red pine (Pinus densiflora S. et Z.) stands in Korea. For. Ecol. Manage., 255, 3318–3321.
- Laganière J, Paré D, Bergeron Y, Chen HY 2012: The effect of boreal forest composition on soil respiration is mediated through variations in soil temperature and C quality. Soil Biol. Biochem., doi: 10.1016/j.soilbio.2012.04.024
- Laporte MF, Duchesne LC, Morrison IK 2003: Effect of clearcutting, selection cutting, shelterwood cutting and microsites on soil surface CO2 efflux in a tolerant hardwood ecosystem of northern Ontario. For. Ecol. Manage., 174, 565–575.
- Liu J, Jiang P, Wang H et al. 2011: Seasonal soil CO2 efflux dynamics after land use change from a natural forest to Moso bamboo plantations in subtropical China. For. Ecol. Manage., 262, 1131–1137.
- Liu H, Zhao P, Lu P, Wang Y-S, Lin Y-B, Rao X-Q 2008: Greenhouse gas fluxes from soils of different land-use types in a hilly area of South China. Agric. Ecosyst. Environ., 124, 125–135.
- Luo J, Chen Y, Wu Y, Shi P, She J, Zhou P 2012: Temporal-spatial variation and controls of soil respiration in different primary succession stages on glacier forehead in Gongga Mountain, China. Plos One., 7, e42354.
- Luo J, Chen Y, Zhu W, Zhou P 2013: Modeling climate change effects on soil respiration in three different stages of primary succession in deglaciated region on Gongga Mountain, China. Scand. J. For. Res., 28, 363–372.
- Mielnick PC, Dugas WA 2000: Soil CO2 flux in a tallgrass prairie. Soil Biol. Biochem., 32, 221–228.
- Mosier AR 1998: Soil processes and global change. Biol. Fert. Soils., 27, 221–229.
- Myneni RB, Keeling C, Tucker C, Asrar G, Nemani R 1997: Increased plant growth in the northern high latitudes from 1981 to 1991. Nature, 386, 698–702.
- Nelson DW, Sommers LE 1982: Total carbon, organic carbon and organic matter. In Methods of Soil Analysis, Ed. Page AL, Miller RH Keeney DR, pp. 539–579. American Society of Agronomy, Madison, WI.
- Ponder F 2005: Effect of soil compaction and biomass removal on soil CO2 efflux in a Missouri forest. Commun. Soil Sci. Plant Anal. 36, 1301–1311.
- Raich JW, Schlesinger WH 1992: The global carbon dioxide flux in soil respiration and its relationship to vegetation and climate. Tellus Ser. B-Chem. Phys. Meteorol., 44, 81–99.
- Raich JW, Tufekcioglu A 2000: Vegetation and soil respiration: correlations and controls. Biogeochemistry, 48, 71–90.
- Rayment M, Jarvis P 2000: Temporal and spatial variation of soil CO2 efflux in a Canadian boreal forest. Soil Biol. Biochem., 32, 35–45.
- Ruehr NK, Buchmann N 2010: Soil respiration fluxes in a temperate mixed forest: seasonality and temperature sensitivities differ among microbial and root–rhizosphere respiration. Tree Physiol., 30, 165–176.
- Rustad LE, Huntington TG, Boone RD 2000: Controls on soil respiration: implications for climate change. Biogeochemistry, 48, 1–6.
- Saiz G, Byrne KA, Butterbach-Bahl K, Kiese R, Blujdeas V, Farrell EP 2006a: Stand age-related effects on soil respiration in a first rotation Sitka spruce chronosequence in central Ireland. Glob. Change Biol., 12, 1007–1020.
- Saiz G, Green C, Butterbach-Bahl K, Kiese R, Avitabile V, Farrell EP 2006b: Seasonal and spatial variability of soil respiration in four Sitka spruce stands. Plant Soil., 287, 161–176.
- Sheng H, Yang Y, Yang Z et al. 2010: The dynamic response of soil respiration to land-use changes in subtropical China. Glob. Change Biol., 16, 1107–1121.
- Shi P-L, Zhang X-Z, Zhong Z-M, Ouyang H 2006: Diurnal and seasonal variability of soil CO2 efflux in a cropland ecosystem on the Tibetan Plateau. Agric. For. Meteorol., 137, 220–233.
- Sun H, Zheng D, Yao T, Zhang Y 2012: Protection and Construction of the National Ecological Security Shelter Zone on Tibetan Plateau (in Chinese). Acta Geogra. Sin., 67, 3–12.
- Takahashi M, Hirai K, Limtong P et al. 2011: Topographic variation in heterotrophic and autotrophic soil respiration in a tropical seasonal forest in Thailand. Soil Sci. Plant Nutr., 57, 452–465.
- Wang X, Jiang Y, Jia B, Wang F, Zhou G 2010: Comparison of soil respiration among three temperate forests in Changbai Mountains, China. Can. J. For. Res. Rev. Canadienne De Recherche Forestiere, 40, 788–795.
- Wang X, Zhua B, Gao M, Wang Y, Zheng X 2008: Seasonal variations in soil respiration and temperature sensitivity under three land-use types in hilly areas of the Sichuan Basin. Aust. J. Soil Res., 46, 727–734.
- Wu J, Liu Z, Chen D, Huang G, Zhou L, Fu S 2011: Understory plants can make substantial contributions to soil respiration: evidence from two subtropical plantations. Soil Biol. Biochem., 43, 2355–2357.
- Wu J, Zhang X, Xu D 2003: The temporal variations of soil respiration under different land use in Liupan Mountain forest zone. Environ. Sci., 24, 23–32.
- Xu M, Qi Y 2001: Soil‐surface CO2 efflux and its spatial and temporal variations in a young ponderosa pine plantation in northern California. Glob. Change Biol., 7, 667–677.
- Xu Z, Wan C, Xiong P et al. 2010: Initial responses of soil CO2 efflux and C, N pools to experimental warming in two contrasting forest ecosystems, Eastern Tibetan Plateau, China. Plant Soil., 336, 183–195.
- Yan JH, Wang YP, Zhou GY, Zhang DQ 2006: Estimates of soil respiration and net primary production of three forests at different succession stages in South China. Glob. Change Biol., 12, 810–821.
- Yan JH, Zhang DQ, Zhou GY, Liu JX 2009: Soil respiration associated with forest succession in subtropical forests in Dinghushan Biosphere Reserve. Soil Biol. Biochem., 41, 991–999.