Abstract
Brachiaria spp. have been grown in a variety of cropping systems and are often terminated with herbicides, which may cause nitrogen (N) loss from the soil-plant system. In this study ammonia (NH3-N) loss by shoots and N balance in a soil-plant system were determined after desiccation of palisade grass (Brachiaria brizantha (Hochst. ex A. Rich) Stapf, cv. Marandu), signalgrass (Brachiaria decumbens Stapf), humidicola (Brachiaria humidicola (Rendle) Schweick) and Congo grass (Brachiaria ruziziensis Germain et Evrard). The grasses were grown in pots filled with an Oxisol in a greenhouse. Sixty days after planting, the plants were desiccated with glyphosate. Analyses were performed on plant and soil at desiccation and then at 7, 14, 21 and 28 days after desiccation in order to assess NH3-N losses by shoots and to estimate the N balance in the system. Total nitrogen (Total-N) concentration in shoots and roots of brachiarias decreased after desiccation, thereby reducing the amount of N in plants of the four brachiaria species. However, as most of the N lost by plants was released into the soil, N losses from the soil-plant system were small compared with the total N in the system: 1.2, 0.5, 0.4 and 1.4% for palisade grass, signalgrass, humidicola and Congo grass, respectively. N losses as NH3 from the soil-plant system after desiccation with glyphosate varied among brachiaria species, ranging from 0.8 to 2.0 g m−2 kg−1, and accounted for 30–80% of total loss.
INTRODUCTION
Brachiarias are widely grown in no-tillage systems because they have high adaptability to various soil types as well as high dry matter yield and quality (Timossi et al. Citation2006). Brachiaria species, especially palisade grass (Brachiaria brizantha (Hochst. ex A. Rich) Stapf) and Congo grass (Brachiaria ruziziensis Germain et Evrard), have been extensively used in Crop-Livestock Integration systems, grown as cover crops during fall/winter. Their main role is to provide soil cover for no-tillage systems but may also be used as forage for livestock in the winter (Borghi and Crusciol Citation2007). This association also increases soil protection and nutrient cycling. In some cases, cash crop yields are increased (Borghi and Crusciol Citation2007). In spite of its low yields, signalgrass (Brachiaria decumbens Stapf) is used in most Brazilian pastures because it is tolerant to low fertility, and humidicula (Brachiaria humidicola (Rendle) Schweick) has been shown to modify soil nitrogen (N) dynamics (Subbarao et al. Citation2009).
Herbicides are widely used to terminate cover crops in crop rotations under no-tillage. The main herbicide used in cover crop desiccation is glyphosate (Rodrigues and Almeida Citation2005), and recent studies have shown that glyphosate may increase both root exudation of N compounds (Damin et al. Citation2010) and N loss by shoots of signalgrass (Damin et al. Citation2008), which may affect N management in cropping systems. Glyphosate blocks the enzyme 5-Enolpyruvylshikimate-3-phosphate synthase (EPSPS) (Duke and Hoagland Citation1985). As a consequence, phenylalanine ammonia-lyase (PAL) activity is increased, and plant ammonium (NH4+-N) concentrations rise to toxic levels (Hoagland et al. Citation1979; Duke and Hoagland Citation1985), resulting in root exudation of N compounds (Kremer et al. Citation2005) and in NH3-N emissions (Mohr et al. Citation1998; Schjoerring et al. Citation1998). N emission depends on the species, stage of development, soil N availability and stomatal conductance, among other factors (Parton et al. Citation1988; Schjoerring et al. Citation1998). According to Mohr et al. (Citation1998), ammonia (NH3-N) losses were highest (12% of the total N in shoots) when alfalfa (Medicago sativa, L.) residues were killed with ghyphosate and left on the soil surface. Plant senescence is one of the plant developmental stages with the highest NH3-N emission potential. In cut leaves, NH3-N is increased as protein are degraded during senescence (Marstorp Citation1995), and the amount of ammonia volatilized is proportional to the leaf N concentrations and temperature (Whitehead et al. Citation1988; Whitehead and Lockyer Citation1989).
Therefore, senescence induction through the application of herbicides such as glyphosate can increase NH3-N emissions by the shoots (Damin et al. Citation2008). Since chemical desiccation of brachiarias can involve significant N losses, it is important to investigate the extent of such losses in different species used in cropping systems. Thus, the aim of this study was to evaluate NH3-N loss by shoots and N balance in the soil-plant system after the desiccation of palisade grass, signalgrass, humidicola and Congo grass, species widely used in tropical humid areas as forage crops and as cover crops.
MATERIALS AND METHODS
The experiment was conducted in a greenhouse at São Paulo State University in Botucatu, State of São Paulo, Brazil. During the evaluation period, the mean highest and lowest temperature were 34.3°C and 20.8°C, respectively, and the average minimum and maximum relative humidity were 51.2% and 83.1%, respectively. Plants were grown in 12.6 dm3 polyvinyl chloride (PVC) pots (0.2 m in diameter and 0.4 m in depth) with sealed bottoms, filled with 12.6 kg of a Rhodic Hapludox (FAO Citation2006) with 539, 151 and 311 g kg−1 of clay, silt and sand, respectively. After liming, soil chemical analyses (Raij et al. Citation2001) showed pH (CaCl2) 5.3; 25 g kg‒1 of organic matter, 8.0 mg kg‒1 of P (resin), 0.8 mmolc kg‒1 of K, 30 mmolc kg‒1 of Ca, 20 mmolc kg‒1 of Mg, 81 mmolc kg‒1 of cation exchange capacity (CEC) and 62% of base saturation. Total-N concentration was 1036 mg kg‒1 (Bremner and Mulvaney Citation1982). The soil was then fertilized with 100 mg kg‒1 of N as ammonium nitrate, 150 mg kg‒1 of P as superphosphate and 12 mg kg‒1 of K as potassium chloride.
Treatments were four brachiaria species and five sampling times plus a control without plants. Twelve pre-germinated seeds were planted and then thinned to three seedlings per pot after 7 d. A top dressing of 50 mg dm‒3 of N (630 mg pot–1) as urea was applied at tillering (21 d after planting). Throughout the experiment, soil moisture was monitored by daily weighing the pots, and adjusted to 70% of the water-holding capacity, even after desiccation. Palisade grass (Brachiaria brizantha, cv. Marandu), signalgrass (Brachiaria decumbens), humidicola (Brachiaria humidicola) and Congo grass (Brachiaria ruziziensis) were grown for 60 d and desiccated with glyphosate at 1440 g ha‒1 a.i. (active ingredient) (2.0 kg ha‒1 Roundup WG® (wettable granules)). The herbicide was applied with a CO2-pressurized backpack sprayer and a spray volume of 150 L ha‒1.
Evaluations were made in the morning following desiccation (0) and then at 7, 14, 21 and 28 days after desiccation (DAD). The shoots were cut close to the soil surface, washed in distilled water, placed in paper bags and dried in a fan-assisted oven at 65°C to constant mass. The same cleaning and drying procedure was adopted for the roots once they were separated from the soil. The samples were weighed, ground and analyzed for N concentration. Total-N and NH4+-N were determined by sulfuric digestion and extraction with potassium chloride (KCl), respectively, and steam distillation (Malavolta et al. Citation1997). Soil samples were air-dried, sieved through a 2-mm sieve, and Total-N was determined in triplicate, via sulfuric digestion and subsequent distillation (Bremner and Mulvaney Citation1982).
The emission of NH3-N was determined using semi-open static chambers (Nömmik Citation1973), with adaptations. The chambers were made of transparent sheets of PVC and two foam pads. The bottom pad collected volatilized NH3-N, while the upper pad prevented contamination with atmospheric ammonia. The chambers were placed over the pots immediately after desiccation and replaced on a weekly basis. The bottom pads were soaked with 45 mL of a 0.17-mol L‒1 phosphoric acid and 50 mL L‒1 of glycerol solution before being placed inside the collectors. The amount of NH3-N lost by the shoots was obtained indirectly by subtracting NH3-N volatilized in the control pots from that in the planted pots.
The sampling (collection and replacement) of the pads was carried out at 1, 3, 5, 7, 10, 14, 17, 20, 24 and 28 DAD. At each sampling, the bottom pads were placed in plastic bags and stored in a refrigerator at 5°C for subsequent NH3-N extraction, which was always done the day after sampling. NH3-N was extracted by rinsing the bottom pad with 400 mL of a 0.0025-M sulfuric acid (H2SO4) solution, and a 50-mL subsample was taken for distillation.
The N balance in the soil-plant system was estimated based on the results of the first (0 DAD) and last (28 DAD) evaluation of the plants and soil, as well as NH3-N losses. All figures used in the N balance calculations were modified so that the results are presented in g pot–1. The amount of N accumulated in plants was obtained by the sum of accumulated N in both roots and shoots for each of the stages. The total N accumulated in soil was obtained by multiplying the Total-N concentration by the amount of soil in each pot. The N balance in plant and soil were calculated by subtracting the accumulated N at 28 DAD from the accumulated N at 0 DAD. The total N in the system at 0 and 28 DAD was calculated by adding the accumulated N in plant and soil for each stage. The N balance in the soil-plant system was obtained by subtracting the amount of N at desiccation from the amount of N at 28 DAD. N losses were normalized over dry matter yields, i.e., they were calculated considering the surface area of the pots and the yield of each grass. Some N loss may have occurred in the form of nitrogen oxides (nitrogen dioxide (NO2), nitrous oxide (N2O) and nitric oxide (NO)) or elemental nitrogen (N2) from both the soil and shoots. This loss was considered as unaccounted-for loss.
The experimental design was a split-plot in complete randomized blocks, with four replicates. The results were submitted to analysis of variance (ANOVA). Regressions were fit to responses through time, and species and N balance means were compared using the Student’s unpaired t test (P > 0.05).
RESULTS
The highest average shoot dry matter yield was observed for Congo grass (55.2 g), followed by signalgrass (43.8 g), palisade grass (38.1 g) and humidicola (23.5 g). However, shoot dry matter was not affected by the time after desiccation. The root dry matter of humidicola was also low compared with the other species (). Root dry matter of humidicola and signalgrass were not affected by time, but decreased linearly by 34.7 and 37.9% for palisade grass and Congo grass, respectively.
Figure 1 Root dry matter of palisade grass (Brachiaria brizantha (Hochst. ex A. Rich) Stapf) (□), signalgrass (Brachiaria decumbens Stapf) (▲), humidicola (Brachiaria humidicola (Rendle) Schweick) (○) and Congo grass (Brachiaria ruziziensis Germain et Evrard) (●) as affected by time after desiccation with glyphosate. ns = non significant, * = significant (F test, P > 0.05) and ** = significant (F test, P > 0.01). Vertical bar indicates least significant difference (LSD) comparing species (P > 0.05).
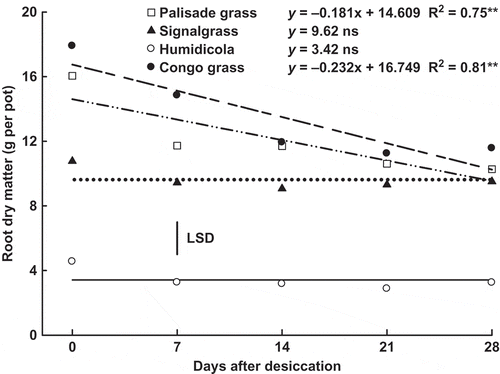
The highest Total-N concentrations for both shoots and roots were found in humidicola. The other species had lower N concentrations, with no significant difference among them (). Although the N concentration in shoots was different among species, its variation through time was similar, decreasing linearly to 16.8 g kg‒1 at 28 DAD (). The N concentration in roots followed a similar pattern, decreasing after desiccation ().
Figure 2 Total nitrogen (Total-N) concentration in (a) shoot and (b) roots of brachiaria grasses as affected by time after desiccation with glyphosate (mean of four species: palisade grass (Brachiaria brizantha (Hochst. ex A. Rich) Stapf), signalgrass (Brachiaria decumbens Stapf), humidicola (Brachiaria humidicola (Rendle) Schweick) and Congo grass (Brachiaria ruziziensis Germain et Evrard)). ** = significant (F test, P > 0.01).
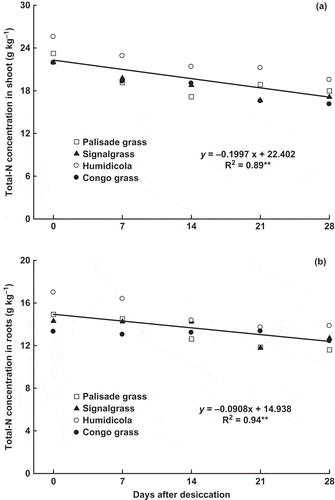
The amount of N in the shoots of palisade grass, signalgrass and Congo grass linearly decreased in time (); for humidicola the decrease was nominal but not significant. Hence, N was lost and/or remobilized from the shoots of these species after desiccation. Despite the decrease in root N concentrations in all species after glyphosate application (), this was not enough to decrease total N amounts in signalgrass and humidicola (). However, significant reductions in the amount of N present in the roots of palisade grass and Congo grass were observed (), resulting in a linear increase of N concentration in soil after desiccation ().
Figure 3 Nitrogen (N) accumulated in (a) shoot and (b) roots in palisade grass (Brachiaria brizantha (Hochst. ex A. Rich) Stapf) (□), signalgrass (Brachiaria decumbens Stapf) (▲), humidicola (Brachiaria humidicola (Rendle) Schweick) (○) and Congo grass (Brachiaria ruziziensis Germain et Evrard) (●) as affected by time after desiccation with glyphosate. ns = non significant, * = significant (F test, P > 0.05) and ** = significant (F test, P > 0.01). Vertical bar indicates least significant difference (LSD) comparing species (P > 0.05).
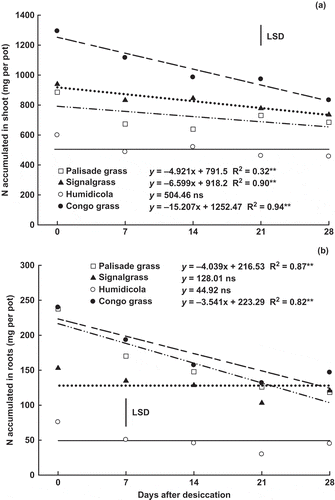
Figure 4 Total nitrogen (Total-N) concentration in soil as affected by time after brachiaria grasses desiccation with glyphosate (mean of four species: palisade grass (Brachiaria brizantha (Hochst. ex A. Rich) Stapf), signalgrass (Brachiaria decumbens Stapf), humidicola (Brachiaria humidicola (Rendle) Schweick) and Congo grass (Brachiaria ruziziensis Germain et Evrard)). ** = significant (F test, P > 0.01).
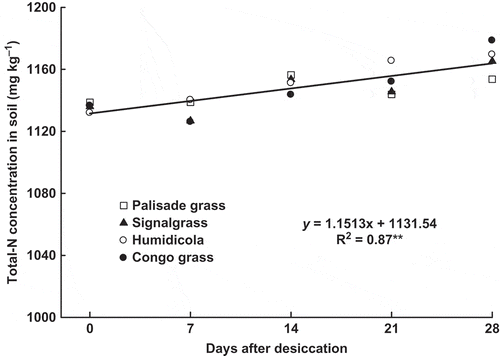
Shoot NH4+-N concentrations were similar for all species, with a quadratic response over time, peaking around 17 DAD (). Considering plant senescence and the mode of action of glyphosate, a rise in NH4+‒N concentration is likely, and it is directly related to NH3-N losses by the shoot (Hoagland et al. Citation1979; Duke and Hoagland Citation1985; Whitehead et al. Citation1988; Damin et al. Citation2008).
Figure 5 Ammonium (NH4+) concentration in brachiaria grasses shoot as affected by time after desiccation with glyphosate (mean of four species: palisade grass (Brachiaria brizantha (Hochst. ex A. Rich) Stapf), signalgrass (Brachiaria decumbens Stapf), humidicola (Brachiaria humidicola (Rendle) Schweick) and Congo grass (Brachiaria ruziziensis Germain et Evrard)). ** = significant (F test, P > 0.01).
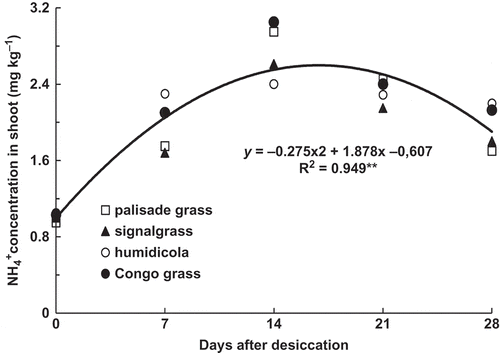
The daily NH3-N losses from the shoots of signalgrass and humidicola increased linearly, while for palisade grass and Congo grass the response was quadratic, with maximum at 21 and 17 DAD, respectively (). The largest accumulated losses of NH3-N were found in Congo grass, reaching 78 mg per pot. Palisade grass, humidicola and signalgrass showed lower losses: 54, 48 and 33 mg per pot, respectively (). It should be noted, however, that 28 d after desiccation humidicola and signalgrass showed increased daily NH3-N losses ().
Figure 6 Temporal variation of ammonia (NH3-N) emission by shoot of palisade grass (Brachiaria brizantha (Hochst. ex A. Rich) Stapf) (□), signalgrass (Brachiaria decumbens Stapf) (▲), humidicola (Brachiaria humidicola (Rendle) Schweick) (○) and Congo grass(Brachiaria ruziziensis Germain et Evrard) (●) as affected by time after desiccation with glyphosate. ns = non significant, ** = significant (F test, P > 0.01). Vertical bar indicates least signficant difference (LSD) comparing species (P > 0.05).
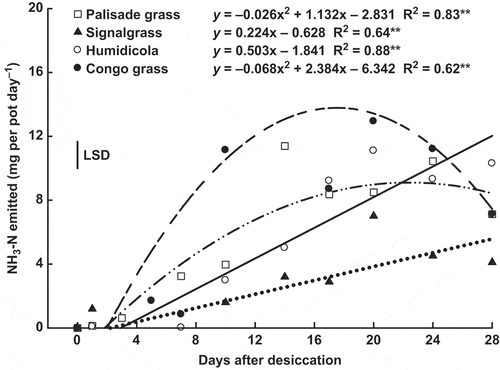
Figure 7 Accumulated emission of ammonia (NH3-N) by shoot of palisade grass (Brachiaria brizantha (Hochst. ex A. Rich) Stapf) (□), signalgrass (Brachiaria decumbens Stapf) (▲), humidicola (Brachiaria humidicola (Rendle) Schweick) (○) and Congo grass (Brachiaria ruziziensis Germain et Evrard) (●) as affected by time after desiccation with glyphosate. * = significant (F test, P > 0.05) and ** = significant (F test, P > 0.01). Vertical bar indicates least signficant difference (LSD) comparing species (P > 0.05).
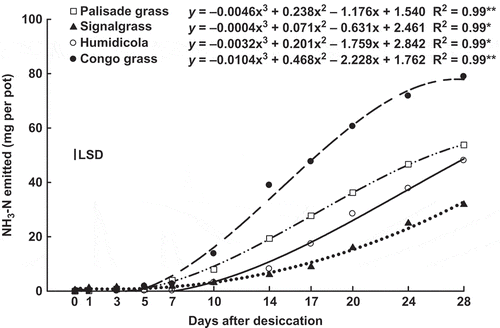
Overall, the plant N balance between 0 and 28 DAD was negative (), indicating that the plants lost N during this period. The largest losses occurred for Congo grass and palisade grass. Such high losses were observed in the roots for both the species and for the shoots of Congo grass. In fact, Congo grass was the species contributing most to N increase in soil (). When the other species were grown, a positive soil N balance was also observed (), probably due to exudation of N compounds by the roots, root detachment and accumulated N mineralization.
Table 1 Nitrogen (N) budget in roots, shoot and entire plant after palisade grass (Brachiaria brizantha (Hochst. ex A. Rich) Stapf), signalgrass (Brachiaria decumbens Stapf), humidicola (Brachiaria humidicola (Rendle) Schweick) and Congo grass (Brachiaria ruziziensis Germain et Evrard) desiccation with glyphosate
Table 2 Nitrogen (N) budget in soil after palisade grass (Brachiaria brizantha (Hochst. ex A. Rich) Stapf), signalgrass (Brachiaria decumbens Stapf), humidicola (Brachiaria humidicola (Rendle) Schweick) and Congo grass (Brachiaria ruziziensis Germain et Evrard) desiccation with glyphosate
The N balance in the soil-plant system was negative for all species (). Losses for palisade grass and Congo grass correspond to 1.2 and 1.4% of the total N in the soil-plant system at 0 DAD, respectively. N lost from the soil-plant system as NH3-N corresponded to 30, 43, 80 and 35% of the total loss for palisade grass, signalgrass, humidicola and Congo grass, respectively. It is interesting to note that the least significant difference (LSD) for values obtained for total N loss are high, a result of high variability.
Table 3 Nitrogen (N) budget in soil-plant system after palisade grass (Brachiaria brizantha (Hochst. ex A. Rich) Stapf), signalgrass (Brachiaria decumbens Stapf), humidicola (Brachiaria humidicola (Rendle) Schweick) and Congo grass (Brachiaria ruziziensis Germain et Evrard) desiccation with glyphosate
DISCUSSION
In this study the soil was fertile and there was adequate water supply. Hence, considering that palisade grass, signalgrass and humidicola yields were shown to be similar, but higher than Congo grass (Botrel et al. Citation1999), it can be inferred that when these forage crops are grown under favorable conditions and N availability is not limiting, Congo grass is more efficient in producing shoot dry matter. Dry matter yield and the rate of root decay are directly related to the nutrient cycling potential of cover crops. The decrease of root dry matter for palisade grass and Congo grass after desiccation () may have occurred either because of their higher susceptibility to the deleterious effects of the herbicide or simply because they had higher root dry matter and their roots were more prone to decay. The application of glyphosate to signalgrass grown in nutrient solution increased root loss by detachment (Damin et al. Citation2010).
The adequate N concentration in shoots of brachiaria plants cut 10 cm aboveground ranges from 12 to 20 g kg‒1 (Raij et al. Citation1996), which was observed in this study (), showing that N availability did not limit plant growth. Thus, the highest N concentrations found in the shoots and roots of humidicola probably resulted from its lower dry matter yield and the consequent N concentration in its tissues.
During senescence, translocation and redistribution of nutrients in plants can occur (Marschner Citation1995), which explains the decreased N concentrations in brachiaria shoots (). However, there was also a decrease in the root N concentration (), showing that N was lost from plants. Such loss may have either occurred via the shoot as gaseous oxides, N and N2, during nitrate reduction in the chloroplasts, or as NH3-N (Farquhar et al. Citation1980; Mohr et al. Citation1998; Schjoerring and Mattsson Citation2001) or by increased root exudation (Damin et al. Citation2010) due to ghlyphosate application (Kremer et al. Citation2005). Glyphosate application increased soil N and reduced plant N in signalgrass, showing that the herbicide either increased N exudation or root detachment (Damin et al. Citation2008).
The amount of N in brachiaria roots () followed the same pattern as in root dry matter (). Hence, the decreased N in roots of palisade grass and Congo grass was rather a consequence of root dry matter decrease than of a decrease in N concentration. However, differences among species as to N exudation from roots cannot be ruled out, since the amount and composition of exudates depends on plant species (Marschner Citation1995; Holland et al. Citation1996). The decreased N amounts in brachiaria roots is possibly related to the soil Total-N concentration (). Regardless of species, soil N increased over time after desiccation, which may have occurred due to nitrogenous compound exudation, root detachment and decay of senescent roots, all intensified by the use of glyphosate (Kremer et al. Citation2005; Damin et al. Citation2010).
As brachiaria shoot dry matter did not decrease after glyphosate application, the decrease in shoot N observed for palisade grass, signalgrass and Congo grass () resulted from a decrease in N concentrations. Plant natural senescence and ghyphosate application increase NH4+-N concentration in plants (Hoagland et al. Citation1979; Duke and Hoagland Citation1985), which promote NH3-N emission through the leaves (Mohr et al. Citation1998) and eventually N loss by shoots, which is higher the higher the tissue N concentration (Whitehead and Lockyer Citation1989) and the temperature (Whitehead et al. Citation1988). In the present experiment, NH4+-N concentration in the shoots increased up to the 17th day after desiccation (), which resulted in higher NH3-N loss during this period. Palisade grass and Congo grass showed the highest NH3-N losses at 21 and 17 DAD respectively, coinciding with the period of highest NH4+-N accumulation (). It has been shown that the start of ammonia volatilization from leaves is linearly related to the tissue protein content (Marstorp Citation1995), but in our experiment the plants had similar N concentrations () and NH3-N volatilization was not similar, showing that protein content is not the sole factor affecting this process.
In current practice the cash crop is usually planted 14–21 d after cover crops desiccation and the residues are not incorporated. This would be an indication that N losses would be likely higher in the field, but it has to be considered that all this happens during the rainy season, and at least some of the N would be returned to the soil, washed from the straw by rains (Rosolem et al. Citation2010).
Although an increase in NH4+-N concentration could lead to greater NH3-N losses by the shoot, they are dependent on many other factors including the species and carbon (C) assimilation pathway (Wendler et al. Citation1990). Another factor that is most likely associated with the NH3-N emission is the amount of N accumulated in plants. In this study, the cumulative NH3-N loss by brachiaria shoots () was correlated with the amount of N accumulated (). Damin et al. (Citation2008) attributed N loss via the shoots of signalgrass (28%) to NH3-N leaf emission, which in this experiment ranged from 3.4 to 9.5% of that accumulated in shoots at 0 DAD ().
As a consequence of the decrease in N amounts in shoots and roots, the N balance for the plant was negative (). Most N losses occurred in Congo grass, possibly as a result of the greater N accumulation in both shoots () and roots (). However, the relative N losses did not follow this pattern, since the relative smallest loss occurred in signalgrass (27%), while palisade grass, humidicola and Congo grass lost 36, 39 and 39% of their N, respectively.
Not all N lost by the plants was lost from the soil-plant system. Part of N is released by roots and/or mineralized in the soil, contributing to N cycling in the cropping system. Congo grass contributed the most to soil N increase, probably because, along with palisade grass, it presented the highest root dry matter (). Congo grass also accumulated more N in its roots, releasing it faster into the soil (). This characteristic is especially important when cover crops are grown in rotational cropping systems as they leave N available for the succeeding crop.
Using the N balance for plant and soil ( and ), it is possible to estimate the proportion of N loss by plants released and/or mineralized in the soil and remaining in the soil-plant system. Signalgrass and humidicola, despite minor decreases in root dry matter () and plant N (), left 74 and 75% of their lost N available in the soil. For palisade grass the percentage was 54% and for Congo grass 64%, indicating greater N loss by shoots.
The N balance in the soil-plant system () was negative for all species showing a small N loss after glyphosate application. These losses may have occurred via both the soil and the shoots. Since all precautions were taken, N losses either by leaching or NH3-N volatilization from the soil can be disregarded. However, some losses may have occurred as nitrogen oxides or N2, during the denitrification/nitrification processes and from the shoots via nitrate reductase in chloroplasts. N losses as N2O are usually low in tropical pastures (Melillo et al. Citation2001). In our experiment, losses as NH3-N accounted for 30–80% of the N total loss from the soil-plant system. It is important to highlight that total N losses from the soil-plant system at 28 DAD ranged from 0.5 to 1.4% of the total N in the system, and amounted to a maximum of 11.6% of the fertilizer applied. When N losses were normalized over dry matter yields, total N losses from the system ranged from 1.8 to 4.7 g m−2 kg−1 (), but N losses as NH3 amounted to from 0.8 to 2.0 g m−2 kg−1. Considering a dry matter yield of 6 t ha−1 the total losses would range from 10.8 to 28.2 kg ha−1 and NH3-N losses would range from 4.8 to 12.0 kg ha−1. The highest relative unaccounted-for losses were observed in palisade grass and Congo grass. These species reached the maximum NH3-N daily loss within the evaluation period () and N was probably lost in ways not quantified in this study. It is important to highlight that a simple extrapolation of the N rate applied to the pots would give 600 kg ha−1, close to the maximum N rates recommended for pastures with brachiarias in São Paulo State (Werner et al. Citation1996).
Table 4 Estimated nitrogen (N) losses from the soil-plant system 28 days after palisade grass (Brachiaria brizantha (Hochst. ex A. Rich) Stapf), signalgrass (Brachiaria decumbens Stapf), humidicola (Brachiaria humidicola (Rendle) Schweick) and Congo grass (Brachiaria ruziziensis Germain et Evrard) desiccation with glyphosate, under greenhouse conditions, with average maximum temperature of 34.3°C and average minimum temperature of 20.8°C. Averaged over four replicates. Applied N rate equivalent to 60 g m−2
Palisade grass and Congo grass presented high N losses after desiccation (), but contributed the most to N increase in the soil. Considering the importance of dry matter yield (for blanketing) and N cycling in the system, Congo grass would be better than humidicola, which showed lower N losses from the system. Signalgrass also presented interesting results, accumulating the same N amount as palisade grass at 28 DAD, with less than half of the loss in the same period. In the soil-plant system, the greatest losses occurred for palisade grass and Congo grass, but it should be noted that N losses from the soil-plant system as NH3 after desiccation with glyphosate were not high for all brachiaria species. Considering the observed N losses, chemical termination of any of these species would be acceptable.
Hence, even though a N rate as high as the equivalent to 600 kg ha−1 was applied, the amount of N loss is acceptable. However, further investigation under field conditions, with lower N rates, and considering the amount of biological N fixation, would be interesting to support the results of the present experiment.
ACKNOWLEDGMENTS
Thanks to FAPESP (State of São Paulo Research Foundation) for funding the present project.
REFERENCES
- Borghi E, Crusciol CAC 2007: Corn yield, spacing and intercropping modalities with Brachiaria brizantha in no-tillage system. Pesq. Agropec. Bras., 42, 163–171.
- Botrel MA, Alvim MJ, Xavier DF 1999: Evaluation of forage grasses for the South region of Minas Gerais. Pesq. Agropec. Bras., 34, 683–689.
- Bremner JM, Mulvaney CS 1982: Nitrogen‒total. In Methods of Soil Analysis 2nd edn, Ed. Page AL, Miller RH, Keeney DR, pp. 595–624. American Society of Agronomy, Madison, WI.
- Damin V, Franco HCJ, Moraes MF, Franco A, Trivelin PCO 2008: Nitrogen loss in Brachiaria decumbens after application of glyphosate or glufosinate-ammonium. Sci. Agric., 65, 402–407.
- Damin V, Trivelin PCO, Carvalho SJP, Moraes MF, Barbosa TG 2010: Herbicide application increases nitrogen (15N) exudation and root detachment of Brachiaria decumbens Stapf. Plant Soil, 334, 511–519.
- Duke SO, Hoagland RE 1985: Effects of glyphosate on metabolism of phenolic compounds. In The Herbicide Glyphosate, Ed. Grossbard E, Atkinson D, pp. 75–91. Butterworths, London.
- FAO – Food and Agriculture Organization of the United Nations 2006: World Soil Resources Report. FAO, Rome (World Reference Base for Soil Resources, 103).
- Farquhar GD, Firth PM, Wetselaar R, Weir B 1980: On the gaseous exchange of ammonia between leaves and the environmental: Determination of the ammonia compensation point. Plant Physiol., 66, 710–714.
- Hoagland RE, Duke SO, Elmore CD 1979: Effects of glyphosate on metabolism of phenolic compounds. III. Phenylalanine ammonia-lyase activity, free amino acids, soluble protein and hydroxiphenolic compounds in axes of dark-grown soybeans. Physiol. Plant, 46, 357–366.
- Holland JN, Cheng W, Crossley DAJ 1996: Herbivore induced changes in plant carbon allocation assestment of below-ground C fluxes using carbon-14. Oecologia, 107, 87–94.
- Kremer RJ, Means NE, Kim S 2005: Glyphosate affects soybean root exudation and rhizosphere microorganisms. Int. J. Environ. Anal. Chem., 85, 1165–1174.
- Malavolta E, Vitti GC, Oliveira AS 1997: Evaluation of the Plant Nutritional Status: Principles and Applications 2nd edn, Associação Brasileira para Pesquisa da Potassa e do Fosfato, Piracicaba.
- Marschner H 1995: Mineral Nutrition of Higher Plants, Academic Press, London.
- Marstorp H 1995: Influence of protein degradation and protein content in cut Lolium multiflorum leaves on the delay in ammonia volatilisation. Swed. J. Agric. Res., 25, 179–183.
- Melillo JM, Steudler PA, Feigl BJ, Neill C, Garcia D, Piccolo MC, Cerri CC, Tian H 2001: Nitrous oxide emissions from forests and pasture of various ages in the Brazilian Amazon. J. Geophys. Res., 106, 179–188.
- Mohr RM, Janzen HH, Entz MH 1998: Nitrogen dynamics under greenhouse conditions as influenced by method of alfalfa termination. 1. Volatile N losses. Can. J. Soil Sci., 78, 253–259.
- Nömmik H 1973: The effect of pellet size on the ammonia loss from urea applied to forest. Plant Soil, 39, 309–318.
- Parton WJ, Morgan JA, Altenhofen JM, Harper LA 1988: Ammonia volatilization from spring wheat plants. Agron. J., 80, 419–425.
- Raij BV, Andrade JC, Cantarella H, Quaggio JA 2001: Chemical Analysis for Determination of Tropical Soils Fertility, Instituto Agronômico, Campinas.
- Raij BV, Cantarella H, Quaggio JÁ, Furlani AMC 1996: Liming and Fertilizer Recommendations for São Paulo State 2nd edn, Instituto Agronômico/Fundação IAC, Campinas.
- Rodrigues BN, Almeida FS 2005: Herbicide Handbook, 5th edn, IAPAR, Londrina.
- Rosolem CA, Werle R, Garcia RA 2010: Nitrogen washing from C3 and C4 cover grasses residues by rain. R. Bras. Ci. Solo., 34, 1899–1905.
- Schjoerring JK, Husted S, Mattson M 1998: Physiological parameters controlling plant-atmosferic ammonia exchange. Atmos. Environ., 32, 491–498.
- Schjoerring JK, Mattsson M 2001: Quantification of ammonia exchange between agricultural cropland and the atmosphere: Measurements over two complete growth cycles of oilseed rape, wheat, barley and pea. Plant Soil, 228, 105–115.
- Subbarao GV, Nakahara K, Hurtado MP et al. 2009: Evidence for biological nitrification inhibition in Brachiaria pastures. PNAS, 106, 17302–17307.
- Timossi PC, Durigan JC, Leite GJ 2006: Efficacy of glyphosate in cover crops. Planta Daninha, 24, 475–480.
- Wendler C, Barniske EM, Wild A 1990: Effect of phosphinothricin (glufosinate) on photosynthesis and photorespiration of C3 and C3 plants. Photosynth. Res., 24, 55–61.
- Werner JC, Paulino VT, Cantarella H, Andrade NO, Quaggio JA 1996: Forrageiras. In Recomendações de adubação e calagem para o Estado de São Paulo, Ed. Raij B, Cantarella H. Quaggio JA, Furlani MAC, pp. 263–27. Instituto Agronômico, Campinas, SP.
- Whitehead DC, Lockyer DR 1989: Decomposing grass herbage as a source of ammonia in the atmosphere. Atmos. Environ., 23, 1867–1869.
- Whitehead DC, Lockyer DR, Raistrick N 1988: The volatilization of ammonia from Perennial Ryegrass during decomposition, drying and induced senescence. Ann. Bot., 61, 567–571.