Abstract
Five cultivars of camelina species (Camelina sativa L.) were grown in the field at two levels of nitrogen (N) under the climate conditions of Hokkaido Island, Japan. N nutrition as well as the genetic difference between cultivars influenced biomass and grain production. Three cultivars responded positively to N application, but not the other two. Increase in grain yield was associated more with grain number than grain size. The plants accommodated the extra grains by increasing pod number. Irrespective of the N level in the soil, α linolenic acid concentration of the grain was very high at 31% of the total fatty acids. In cultivar Calena, lower leaves on the main stem were excised to find the effect of reduced source area on yield. Defoliation of lower leaves did not affect grain yield, indicating that these leaves do not contribute to source capacity of the plant during grain filling. However, removal of all leaves decreased grain yield to 70% relative to the control plant. In contrast to defoliation, shading of the whole panicle consisting of the pod shell and rachis was more effective in reducing grain yield. Shading of the panicle prior to the grain filling period at the levels of 33 and 6% irradiance relative to control decreased grain yield to 39 and 26%, respectively. These results suggested that the pod shell and rachis are more important source organs for photosynthetic carbon supply to grains than the upper leaves, and they can sustain grain filling in exigency. The presence of attributes like extended source area for grain filling and high α-linolenic acid content of leaves is an indicator for successful adaptation of Camelina in the stress-prone environment of Hokkaido Island.
INTRODUCTION
Camelina sativa L. has been cultivated in Europe for over 2000 years for oil and livestock fodder (Cherian Citation2012). Recently, demand for the crop has increased significantly because of its potential for non-food utilization of seed oil as biodiesel (Putnam et al. Citation1993). Also, Camelina sativa oil contains omega-3 acids with cholesterol-lowering properties in the human diet (Chapman et al. Citation1983; Zubr and Matthäus 2002). The plant is sturdy and survives with low input of agronomic features (Putnam et al. Citation1993). It sustains growth under adverse environmental conditions, such as low temperature, drought and suboptimal nutritional conditions. However, potential crop yield is seldom achieved because of improper management of the cultivation conditions. Both seed number and weight decrease when the right combination of genotype and environment is not attained in the field. This problem has adversely influenced grain yield, and, compared to about 4.0 t ha−1 grain yield of another oil seed crop, rapeseed (Brassica napus L.) (Wittkop et al. Citation2009), Camelina sativa has a maximum yield of 2.8 t ha−1 (Vollmann et al. Citation2007). In general, poor yield in oil crops is assumed to be partly due to the higher energy requirement for synthesis of oil and fatty acid in grains. But, subject to good agronomic management, seed size and high oil content can be achieved in the newly developed cultivars of Camelina sativa (Vollmann et al. Citation2007). Today, Camelina sativa is an under-exploited crop and there is no data available on analysis of factors limiting grain production based on the partitioning of assimilates between the source and sink organs. The subtending leaf is the source of photosynthate for fruit in rice (Oryza sativa) (Mohapatra et al. Citation2004), corn (Zea mays L.) (Sawada et al. Citation1995), and tomato (Solanum lycopersicum L.) (Tanaka and Fujita Citation1974). Visual symptoms such as small leaf area of the subtending leaf and pod shell of a dark-green color resembling leaves during the grain filling period as well as minuscule seed size suggest that the source and sink relationship is a crucial factor to determine grain production in Camelina sativa. In contrast, such investigations in cereals like rice (Mohapatra et al. Citation2004; Kariali and Mohapatra Citation2007), wheat (Triticum L.) (Passioura Citation2002) and corn (Sawada et al. Citation1995) have contributed immensely to knowledge of crop improvement.
Although Camelina sativa is commonly cultivated under low nitrogen (N) levels, it has been observed that a higher grain yield can be obtained by increasing the rate of N application (Agegnehu and Honermeler Citation1997, Crowley and Frohlich Citation1998; Crowley Citation1999; Urbaniak et al. Citation2008; Koncius and Karcauskiene Citation2010; Losak et al. Citation2010, Citation2011; Imbrea et al. Citation2011). Pan et al. (Citation2011) reported that Camelina sativa is very sensitive to both water and N deficiency; the regulative capacity of N supply on photosynthesis and growth is adversely influenced by low water status. This interactive effect of N and water has identified the photosynthetic limitations restricting grain production of Camelina sativa. Further, there is considerable variation in the agronomic features of Camelina sativa genotypes grown in the field; the genotypes vary in grain yield and seed quality as expressed in oil and protein contents (Vollmann et al. Citation2007). These differences indicate considerable variation in carbon (C) and N partitioning between the genotypes and widen scope for genetic manipulation of Camelina sativa for adaptation in stress-prone environments. However, as of today, the genetic difference of N utilization responsible for variation in source activity as well as carbon partitioning has not been assessed properly. Neither have we elucidated the nature of source organs contributing assimilate supply to reproductive sinks for grain growth. In our laboratory, N deficiency was found to affect sink capacity, such as pod number, limiting grain production of soybean (Glycine max) (Nobuyasu et al. Citation2003). Additionally, we identified the contribution of source leaves to grain filling in both high- and low-yielding rice cultivars with carbon radioisotopes (Mohapatra et al. Citation2004). In the present endeavour, we intend to examine whether Camelina sativa can be adapted to the stress-prone climate in Hokkaido, Japan, and to identify the nature of the source organ responsible for the supply of photosynthetic assimilates to developing grains of the crop under common cultivation practices.
MATERIALS AND METHODS
Experiment I: differential N application effects on five genotypes
Five genotypes of camelina (Camelina sativa L.), Celine, Calena, CGX-40, CA4X and CA9X-21, were grown in a field experiment in Hokuto, Hokkaido, Japan (41°84’65”N, 140°65’95”E, 10 m above sea level). Out of the five, Celine and Calena are used as commercial cultivars. Planting distance and plant density of the crops were 12 × 6 cm and 139 plants m–2 respectively. The period of growth was from May to July in 2011. The dates of planting, flowering and harvesting were May 28, July 3 and August 2, respectively. The maturity periods of these genotypes were approximately similar. Annual average temperature was 9.7°C and annual precipitation was 1152 mm. The soil of the experimental area was an alluvial soil. Fertilizers were applied basally at the rate of 30 or 130 kg N ha−1, 87.4 kg phosphorus (P) ha−1 and 166 kg potassium (K) ha−1. In addition, calcium carbonate equivalent to 1000 kg ha−1 was applied basally at the same time. The experimental design was a complete randomized block design with three replicates. At maturity, plant biomass, grain yield and yield components were measured.
Oil extraction, fatty acid and tocopherol composition
Seeds of cultivar Calena grown at two N levels were used for extraction of oil by cold press (KT100-400, Sun seiki Co. Ltd.). An aliquot of oil was employed for analysis of fatty acid composition. The refined solution was injected in the high-performance liquid chromatography (HPLC) and fatty acid concentration was determined by matching with authentic fatty acids of known concentration. Similarly, tocopherol composition was measured by the HPLC method.
Experiment II: defoliation and shading effects on plant biomass
In Experiment II, the cultivar Calena was grown from June to August in 2012, and the dates of planting, flowering and harvesting were June 4, July 16 and August 22, respectively. The method of cultivation was similar to that in Experiment I. With the exception of 60 kg N ha−1, the rate of basal application of other fertilizers was identical. The annual average temperature was 9.5°C and annual precipitation was 1153 mm. At the initiation of the pod filling stage on July 27, plants were divided into six groups and treatments for defoliation, depodding and shading were given as detailed below. Group 1: control plants with no treatments; Group 2: defoliation of all leaves; Group 3: defoliation of lower leaves which were produced on the main stem and equivalent to about 77% of whole plant leaf area; Group 4: Shading I – panicles of a plant were covered with two sheets of shade net which reduced irradiance by 94%, and transmittance was 6%; Group 5: Shading II – panicles of a plant were covered with a sheet of shade net which reduced irradiance by 67%, and transmittance was 33%. At maturity (on August 22), plant biomass, grain yield and yield components were measured.
RESULTS
Experiment 1: differential N application effects on five genotypes
No significant difference in grain yield was observed among camelina cultivars at the low N rate (30 kg N ha−1) (). At the high N rate (130 kg N ha−1), grain yield increased in CG3X-40, CA4X-4 and CA9X-21; the last one recorded the highest yield of 1.9 t ha−1. This difference was mainly due to the difference in grain number rather than grain size (data not shown). Cultivars Celine and Calena did not respond to high N treatment.
Figure 1 Grain yield in camelina (Camelina sativa L.) genotypes grown at different nitrogen (N) rates. Dark and light gray indicate 30 kg N ha−1 and 130 kg N ha−1, respectively. Bars in the graph indicate standard deviation (SD) in the different N levels. Camelina sativa genotypes are denoted as follows: (1) Celine, (2) Calena, (3) CG3X-40, (4) CA4X-4, (5) CA9X-21.
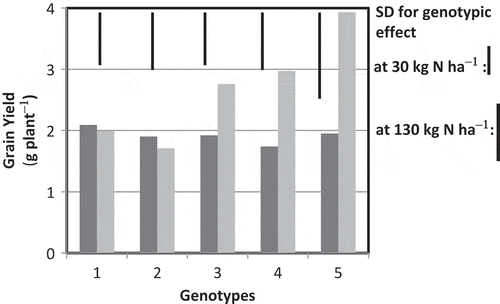
There was no significant difference in fatty acid composition in seed oil among plants grown at different N rates (). Among fatty acids, α-linolenic acid content was highest at about 31%, followed by α-linoleic acid at about 18%, irrespective of N rates. α-tocopherol, γ-tocopherol andδ-tocopherol contents were 19, 916 and 24 mg kg−1, respectively (data not shown).
Table 1 Content of fatty acids in oil of Camelina (Camelina sativa L.) seed (percent of fatty acid) as affected by nitrogen (N) rates (means ± standard error)
Experiment 2: defoliation and shading effects on plant biomass
Whole plant biomass decreased slightly with detachment of all leaves, while it was depressed significantly by shading of panicles and the magnitude of reduction was more severe with the heavier shading (). Grain yield was not affected by removal of lower leaves; however, it decreased to 70% relative to the control (1.6 t ha−1) by complete defoliation (). Complete defoliation reduced both grain number and weight significantly, but the effect was larger on the former than the latter. Grain number per plant declined because defoliation reduced pod number per plant more than it did grain number per pod (). Compared to defoliation treatments, shading of the panicle at the initiation of grain filling was more effective in reducing grain yield; the shading treatments reduced grain yield per plant by decreasing grain and pod numbers as well as 1000-grain weight (). The adverse effect of shading on grain yield increased with decrease in the level of irradiance. Depodding treatment by removal of all but three basal pods had no significant effect on 1000-grain weight (data not shown).
Figure 2 Plant biomass in a Camelina (Camelina sativa L.) genotype as affected by defoliation and shading. Treatment was applied at flowering and measurement was made at maturity.
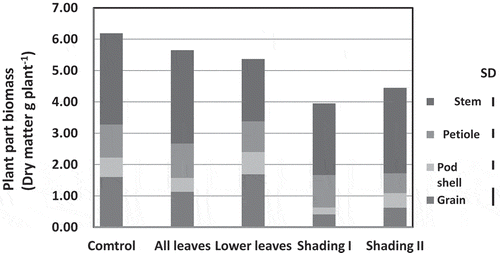
Table 2 Effect of defoliation and shading on grain yield and yield components in Camelina sativa L.
DISCUSSION
Could Camelina sativa adapt to the climate in Hokkaido?
In the present study, grain yield in Camelina sativa ranged from 1 to 2 t ha−1 in the temperate climate of Hokkaido, Japan, which is equivalent to that in European countries (Putnam et al. Citation1993; Vollmann et al. Citation1996, Citation2005). The fatty acid composition of seed oil of the grains did not exhibit any change compared to that reported earlier (Putnam et al. Citation1993; Zubr 2003; Gugel and Falk Citation2006). The concentration of α-linolenic acid was highest among the fatty acids accounting for 31% of the total. No other oil crop except linseed (46%) possesses such a high concentration of α-linolenic acid. It is possible that Camelina sativa acclimated successfully to the cold climate prevalent in Hokkaido Island by maintaining the high producing function of highly unsaturated fatty acids. It is reckoned that chilling-resistant plants have a greater proportion of unsaturated fatty acids (Taiz and Zeiger Citation2002); they acclimatize to low-temperature stress by activating fatty acid desaturase enzyme genes for increasing the concentration of trienoic fatty acids (Iba Citation2002), and α-linolenic acid is the most prominent among them. In our experiment, there was no difference in α-linolenic acid content in grain of Camelina sativa grown at different N rates (). Tocopherol composition was similar to previous findings showing significant high figure in γ-tocopherol (Zubr Citation2009).
This observation suggests that, irrespective of variation in an important edaphic factor, the plant has maintained the unique property of tolerance to low-temperature stress. We recommend wide cultivation of Camelina sativa in the stress-prone environment of Hokkaido, Japan.
Factors controlling grain production in Camelina sativa
In the present experiment, three out of the five cultivars used responded positively for grain yield to high N treatment, and the other two cultivars showed no response (). The increase in grain yield in the well-responding cultivars was mainly caused by an increase in grain number per plant (), but not by 1000-grain weight (). Increase in grain number per plant was more closely associated with pod number per plant than grain number per pod (). These results identified diversity in fertilizer management required for Camelina sativa in Hokkaido Island. N nutrition could have improved greater source activity for all five cultivars used, but it was not helpful in enhancing sink activity for higher pod number in two of them. Lack of response on the sink, at least in some cultivars, suggests that grain production in Camelina sativa could be limited by sink capacity.
There are several crops like field bean (Phaseolus vulgaris L.) (Tanaka and Fujita 1980), corn (Sawada et al. Citation1995), soybean (Yamada et al. Citation2011), rice (Mohapatra et al. Citation1993; Citation2004; Patel and Mohapatra Citation1996) in which grain production is limited more by sink capacity than source capacity during the grain filling period. In the present study, it was shown that grain production was not affected by the removal of lower leaves in Camelina sativa (), because they did not constitute the source area for the grains. Grain production was affected when either the upper leaves were excised or the pod wall and rachis were exposed to low irradiance. These results suggested that, similar to other grain crops mentioned above, an oil crop like Camelina sativa also possesses source capacity in excess for sink filling of developing grains.
Additionally, it was found that size of the sink determining grain yield of the plant can be manipulated by improving grain number per pod and not grain weight. We noticed that removal of some of the pods did not improve weight of the residual grains. Source activity at the stage of initiation of grain filling could be crucial for determination of sink capacity for grain number per pod of the plant because shading of the panicle at this time impaired development of inflorescence and pod formation in our experiment (data not shown). Further, our study identified rachis and pod shell as an additional source for photosynthetic assimilates for grain development in Camelina sativa. There are several examples like this in other crops, such as awn of barley (Hordeum vulgare L.) (Johnson et al. Citation1975), husk leaf of corn (Sawada et al. Citation1995) and culm and leaf sheath of rice (Yoshida Citation1981). However, in our experiment, defoliation treatment was not as effective in lowering grain filling compared to panicle shading. Thus, it is assumed that source activity of the latter is more important than that of the former. This discovery is unique to Camelina sativa and significantly differs from other crops where grains derive photosynthetic assimilates primarily from the leaves subtending the reproductive structures. Possession of an alternative source for C assimilates in exigency is an attribute essential for stress tolerance of Camelina sativa in a sub-optimal environment. Panicle photosynthesis can sustain grain filling in Camelina sativa when low temperature or frostbite damages leaf photosynthesis.
ACKNOWLEDGMENTS
The authors would like to thank the staff at Hokkaido Prefectural Donan Agricultural Experiment Station for technical assistance.
REFERENCES
- Agegnehu M, Honermeler B 1997: Effects of seeding rates and nitrogen fertilization on seed yield, seed quality and yield components of false flax (Camelina sativa Crtz). Bodenkultur., 48, 15–20.
- Chapman DJ, De-Felice J, Barber J 1983: Growth temperature effects on thylakoid membrane lipid and protein content of pea chloroplasts. Plant Phys.,72, 225–228.
- Cherian G 2012: Camelina sativa in poultry diets: opportunities and challenges. In: Biofuel Co-products as Livestock Feed “Opportunities and Challenges”, Ed. Makkar HPS, pp. 303–651. Food and Agriculture Organization of the United Nations (FAO), Rome. http://www.fao.org/docrep/016/i3009e/i3009e00.htm (December, 2013)
- Crowley JG 1999: Evaluation of Camelina sativa as an Alternative Oilseed Crop. Crops Research Centre, Oak Park, Carlow. Teagasc, Dublin.
- Crowley JG, Frohlich A 1998: Factors affecting the composition and use of Camelina. Irish Agriculture and Food Development Authority. http://www.teagasc,ie/research/reports/crops/4319/eopr-4319.htm.
- Gugel RK, Falk KC 2006: Agronomic and seed quality evaluation of Camelina sativa in western Canada. Can. J. Plant Sci., 86, 1047–1058.
- Iba K 2002: Acclimative response to temperature stress in higher plants: approaches of gene engineering for temperature tolerance. Annu. Rev. Plant Biol.., 53, 225–245.
- Imbrea F, Jurcoane S, Halmajan HV, Duda ML 2011: Camelina sativa: A new source of vegetal oils. Rom. Biotechnol. Lett.., 16, 6263–6270.
- Johnson RR, Willmer CM, Moss DN 1975: Role of awns in photosynthesis, respiration, and transpiration of barley spikes. Crop Sci.,15, 217–221.
- Kariali E, Mohapatra PK 2007: Hormonal regulation of tiller dynamics in differentially-tillering rice cultivars. Plant Growth Regul., 53, 215–223.
- Koncius D, Karcauskiene D 2010: The effect of nitrogen fertilizers, sowing time and seed rate on the productivity of Camelina sativa. Zemdirbyste., 97, 37–46.
- Losak T, Hlusek J, Martinec J, Vollmann J, Peterka J, Filipeik R, Varga L, Ducsay D, Martensson A 2011: Effect of combined nitrogen and sulphur fertilization on yield and qualitative parameters of Camelina sativa [L.]Crtz. (false flax). Acta Agriculturae Scandinavica, Sect.B-Soil & Plant Sci., 61, 313–321.
- Losak T, Vollmann J, Hlusek J, Peterka J, Filipeik R, Praskova L 2010: Influence of combined nitrogen and sulphur fertilization on false flax (Camelina sativa [L.] Crtz.) yield and quality. Acta Alimentaria., 39, 431–444.
- Mohapatra PK, Masamoto Y, Morita S et al. 2004: Partitioning of 13C-labelled photosynthate varies with growth stages and panicle size in high yielding rice. Funct. Plant Biol., 31, 1–9.
- Mohapatra PK, Patel R, Sahu SK 1993: Time of flowering affects grain quality and spikelet partitioning within the rice panicle. Aust. J. Plant Physiol., 20, 231–241.
- Nobuyasu H, Liu S, Adu-Gyamfi JJ, Mohapatra PK, Fujita K 2003: Variation in the export of 13C and 15N from soybean leaf: the effects of nitrogen application and sink removal. Plant and Soil., 253, 331–339.
- Pan X, Lada R, Caldwell CD, Falk KC 2011: Photosynthetic and growth responses of Camelina sativa (L.) Crantz to varying nitrogen and soil water status. Photosynthetica., 49, 316–320.
- Passioura JB 2002: Environmental biology and crop improvement. Funct. Plant Biol., 29, 537–546.
- Patel R, Mohapatra PK 1996: Assimilate partitioning within floret components of contrasting rice spikelets producing qualitatively different types of grains. Aust. J. Plant Physiol., 23, 85–92.
- Putnam DH, Budin JT, Field LA, Breene WM 1993: Camelina: A promising low-input oilseed. In New crops, Eds. Janick J, Simon JE, pp. 314–322. Wiley, New York.
- Sawada O, Ito J, Fujita K 1995: Characteristics of photosynthesis and translocation of 13C-labelled photosynthate in the husk leaves of sweet corn. Crop Sci., 35, 480–485.
- Taiz L, Zieger E 2002: Plant Physiology. 3rd Edn. Sinauer Associates Inc. Publishers, Massachusetts, USA.
- Tanaka A, Fujita K 1974: Nutrio-physiological studies on tomato plant IV. Source sink relationship and structure of source-sink unit. Soil Sci. Plant Nut., 20, 305–315.
- Tanaka A, Fujita K 1980: Growth, photosynthesis and yield components in relation to grain yield of the field bean. J. Fac. Agr. Hokkaido Univ., 59, 145–238.
- Urbaniak SD, Caldwell CD, Zheljazkov VD, Lada R, Luan L 2008: The effect of cultivar and applied nitrogen on the performance of Camelina sativa L. in the Maritime Provinces of Canada. Can. J. Plant Sci., 88, 111–119.
- Vollmann J, Damboeck A, Eckl A, Schrems H, Ruckenbauer P 1996: Improvement of Camelina sativa, an underexploited oilseed. In Progress in new crops, Ed. Janick J, pp. 357–362. ASHS Press, Alexandria, VA.
- Vollmann J, Grausgruber H, Stift G, Dryzhyruk V, Lelley T 2005: Genetic diversity in Camelina germplasm as revealed by seed quality characteristics and RAPD polymorphism. Plant Breed., 124, 446–453.
- Vollmann J, Moritz T, Kargl C, Baumgartner S, Wagentriskl H 2007: Agronomic evaluation of Camelina genotypes selected for seed quality characteristics. Ind. Crops Prod., 26, 270–277.
- Wittkop B, Snowdon RJ, Friedt W 2009: Status and perspectives of breeding for enhanced yield and quality of oilseed crops for Europe. Euphytica., 170, 131–140.
- Yamada K, Sasakura A, Nishiwaki K, Shemy EL-, Mohapatra A, Nguyen PK, Kurosak Kanai NT, Ito J, Fujita K 2011: Effect of terminal locations of pods on biomass production and 13C partitioning in a fascinated stem soybean Shakujyo. J. Zhejiang Univ.-Sci. B Biomed. & Biotechnol., 12, 72–82.
- Yoshida S 1981: Fundamentals of rice crop science. International Rice Research Institute, Phippines.
- Zubr J 2003: Qualitative variation of Camelina sativa seed from different locations. Ind. Crops Prod., 17, 161–169.
- Zubr J 2009: Unique dietary oil from Camelina sativa seed. AgroFood Ind. Hi-tech., 20, 42–46.
- Zubr J, Matthäus B 2002: Effects of growth conditions on fatty acids and tocopherols in Camelina sativa oil. Ind. Crops Prod., 15, 155–162.