Abstract
Measuring the phosphorus (P) solubility in animal manure compost (AMC) is important to estimate both the risk of P loss from agricultural land and the P availability for crops in agriculture that use AMC as fertilizer. Water-extractable phosphorus (WEP) has most often been used to estimate P solubility in AMC. A single water extraction with a high ratio of compost to water is usually used to determine WEP, but in many cases, this may not evaluate the maximum WEP. In this study, we extracted the P included in AMC by using a continuous extraction method with a large amount of water, and tested this approach for 16 AMCs: four cattle manure composts, five swine manure composts, five layer (chicken) manure composts, and two broiler (chicken) litter composts. The P dissolution patterns were fitted to kinetic models, and the maximum WEP (WEPmax) was determined by the coefficient of the non-linear regression equation. The WEPmax values corresponded to the sum of H2O- and sodium bicarbonate (NaHCO3)-extractable P measured using the modified Hedley sequential extraction method. The result also suggests that the maximum amount of WEP from AMC can be estimated rapidly using a sequential extraction (the Hedley method) that has been widely used to characterize P in manure or AMC. The maximum water-extractable magnesium (Mg) (WEMgmax) was significantly positively correlated with WEPmax (r = 0.854, P < 0.01). This suggests that WEPmax in the AMCs is affected by water-soluble magnesium compounds.
INTRODUCTION
Animal manure and its compost (AMC) contain large amounts of nitrogen (N) and phosphorus (P) that can be recycled and used as fertilizer in agricultural fields. The ratios of nitrogen to phosphorus (N/P) in these materials are usually lower than those of commercial fertilizers that have been blended to meet the nutrient demand of crops. The difference in the N/P ratio between AMC and crop requirements results in P accumulation in the soil when AMC is supplied in sufficient quantity to meet the N needs of crops over several years (Eghball and Power Citation1999). The accumulation of P in agricultural fields can increase the risk of non-point-source P losses and accelerate eutrophication of lakes and streams. To solve this problem, it is necessary to be able to predict P discharge from soils fertilized with manure or AMC and to establish a P-based AMC application system (Sharpley and Moyer Citation2000; Gérard-Marchant et al. Citation2005). In such a system, the AMC application rate is chosen so as to supply the P required by crops, and N fertilizer is added because the amount of available N supplied from the AMC is insufficient to maintain crop yield. To use this system, it is necessary to accurately evaluate the amounts of available P in manure and AMC.
P availability in manure and AMC, and the risk of P loss from agricultural lands amended with manures or their composts, have been evaluated based on the water-extractable P (WEP). For instance, Sharpley and Moyer (Citation2000) showed that the amount of P that leached from manures and their composts during simulated rainfall events was significantly correlated with their WEP. However, the maximum amount of WEP cannot be measured by the conventional extraction method, which is typically based on a single water extraction with a high ratio of manure or AMC to water. Gérard-Marchant et al. (2005) developed first- and second-order P-release kinetics models for manure and AMC. In some manures and AMCs, the maximum amounts of WEP described by their kinetics models were higher than the WEP measured using the single-extraction method. Toor et al. (Citation2006) reported that the WEP of dairy cattle manures increased as the manure to water ratio decreased from 1:200 to 1:400 [weight/volume (w/v)].
The sequential chemical fractionation method developed by Frossard et al. (Citation1994) has been applied to characterize P in organic wastes. Frossard et al. (Citation1994) modified the method developed for soil P characterization by Hedley et al. (Citation1982). In Hedley’s original method, the soil P is sequentially extracted with an anion exchange resin, 0.5 M sodium bicarbonate (NaHCO3), 0.1 M sodium hydroxide (NaOH), and 1.0 M hydrochloric acid (HCl). In the modified method, the material (manure or AMC) is extracted with water instead of the resin during the first step. This water-extractable phosphorus (H2O-P) represents the most soluble P fraction, and is thought to be the fraction most readily absorbed by crops. Sodium bicarbonate-extractable phosphorus (NaHCO3-P) has moderate solubility. The other P fractions (the NaOH- and HCl-extractable P) have low solubility (Hedley et al. Citation1982; Frossard et al. Citation1994). This method is widely used to characterize the P in organic wastes such as manure or compost (Frossard et al. Citation1994; Dou et al. Citation2000; Sharpley and Moyer Citation2000; He et al. Citation2003; Turner and Leytem Citation2004; Wienhold and Miller Citation2004; Toor et al. Citation2005; Zuvomuya et al. Citation2006; Ito et al. 2010). However, the water solubility of each P fraction is not clear because the extractants used in the modified version of Hedley’s method have different natures. The phosphate species that may affect P solubility in AMC have been examined in several studies. Toor et al. (Citation2005) estimated the ratios of aqueous phosphate and dicalcium phosphate to total P in manure by means of X-ray absorption near-edge structure (XANES) spectroscopy. Kleinman et al. (2005) found positive correlations between WEP in manure and water-extractable calcium (Ca), and magnesium (Mg). Komiyama et al. (2013) showed that P in struvite (MgNH4PO4 · 6H2O) accounted for 66% of the WEP in swine manure compost based on chemical analysis and X-ray diffraction measurements. Continuous extraction has been applied to determine the dissolution rate of soluble substances. For instance, Dahlgren and Walker (Citation1993) adopted this method to determine the rate of aluminum (Al) release from soils, and determined the source of Al dissolved from the soils. If AMC is extracted continuously with a large volume of water, it might be possible to determine the maximum WEP and predict the cation species that affect P solubility. Furthermore, the maximum amount of WEP can be estimated from the P fractions determined by sequential extraction, a method that has been widely used for P characterization of manure and compost.
The objectives of the present study were to determine the maximum WEP in several AMCs produced in Japan by means of continuous water extraction, and to determine whether the maximum WEP can be estimated based on the P fractions measured by means of conventional sequential extraction. Another objective was to identify the cation species that affect the solubility of P in AMC by comparing the amounts of cations and of P extracted by water.
MATERIALS AND METHODS
Samples and chemical analysis
We collected 16 AMCs from several prefectures in Japan: four cattle manure composts (CMC1 to CMC4), five swine manure composts (SMC1 to SMC5), five layer (chicken) manure composts (LMC1 to LMC5), and two broiler (chicken) litter composts (BLC1 and BLC2). Water contents were low (<5 0%), especially for the LMCs (less than 20%). The samples were air-dried and ground to pass through a 0.5-mm screen before analysis.
For measurements of total P and the total for the other elements, each sample was digested in a nitric acid-perchloric acid mixture (Page et al. 1982). After digestion, the concentration of P in the solution was determined colorimetrically using the method of Murphy and Riley (Citation1962). The concentrations of potassium (K), Ca, Mg, iron (Fe) and Al in the solution were determined by atomic absorption spectrometry (Z6000; Hitachi, Tokyo, Japan). Total contents of N and carbon (C) were determined by dry combustion using an NC analyzer (NC-80S; Sumika Chemical Analysis Service, Ltd., Osaka, Japan). In order to characterize the P composition of the AMC, P fractionation was conducted by means of the sequential extraction method of Hedley et al. (Citation1982), as modified by Frossard et al. (Citation1994). We sequentially extracted 0.15 g of air-dried AMC using 30 mL of deionized water, 30 mL of 0.5 M NaHCO3 (pH = 8.5), 30 mL of 0.1 M NaOH, and 30 mL of 1 M HCl. After each extraction, which was conducted with continuous shaking for 16 h at 25°C, the inorganic P concentration of the supernatant was determined colorimetrically (Murphy and Riley Citation1962). In this study, the organic P concentrations of each fraction were not determined because Sharpley and Moyer (Citation2000) and Yokota et al. (Citation2003) showed that most of the P in AMC was present in an inorganic form. We therefore assumed that the sum of all four P fractions equaled the total inorganic P, and defined the difference between the total P and the inorganic P as the residual P (Resid-P).
Continuous water extraction for P and cations
We conducted a continuous water extraction experiment to determine the dissolution rates and the maximum amount of WEP and cations in the AMC using a stirred, flow-through reaction vessel (Dahlgren and Walker Citation1993) at 25°C (). We added 0.25 g of a given AMC to the 75-mL reaction vessel with 50 mL of distilled water. The initial ratio of compost to water was the same as in the sequential chemical fractionation method. The reaction vessel contained a magnetic stirrer and the stirred solution was drawn out of the cell through a 0.45-μm nylon membrane filter. The water flow rate through the reaction cell was maintained at about 40 mL h−1 by a peristaltic pump; the accuracy of the flow rate was determined by measuring the solution weight in each collection tube. The cumulative water volume and total extraction time were about 800 mL and 20 h, respectively. P concentrations in the extracts were determined colorimetrically for each 10–20 mL of collected solution (i.e., for each 15- to 30-min interval) until the cumulative water volume reached 200 mL (a total extraction time of 5 h); thereafter, measurements were obtained for each 50–100 mL of solution (i.e., each 75- to 150-min interval) from 200 to 800 mL of cumulative water volume (i.e., from 5 to 20 h of extraction time). We also determined the concentrations of dissolved Ca and Mg in all extracts from all composts by means of atomic absorption spectrometry. We determined the K concentrations for eight samples (CMC1, CMC2, SMC1, SMC2, LMC1, LMC2, BLC1 and BLC2). We also measured the Fe and Al concentrations by means of atomic absorption spectrometry, but they were below the detection level.
Statistical analysis and model development
We tested for significant differences between the means of the chemical compositions and the P fractions of the AMCs using Tukey’s highly significant difference (HSD), with significance at P < 0.05. In the continuous water extraction experiment, we described the dissolution patterns of each element (P, Ca, Mg and K) using the two simple kinetics models examined by Gérard-Marchant et al. (2005). The cumulative dissolution of P from the AMC was fitted to first-order (Eq. 1) and second-order (Eq. 2) equations:
We also analyzed the cumulative dissolution patterns for Ca, Mg, and K from the AMC using the same kinetics models and determined WECamax, WEMgmax and WEKmax, which represent the maximum amount of water-extractable Ca, Mg and K, respectively. All analyses were conducted using JMP v4.0.5.J (SAS Institute 2001).
RESULTS
Chemical properties and P compositions of the AMCs
The chemical properties of the AMCs differed among the livestock types (). The mean P content was highest for SMC (36.0 mg P g−1), followed by LMC (28.9 mg P g−1), BLC (16.8 mg P g−1), and CMC (12.4 mg P g−1). The P content was significantly higher for SMC and LMC than for CMC. The mean Ca content of LMC (155 mg Ca g−1) was significantly greater than those of the other AMCs because Ca compounds are usually added to the feed of layer chickens to produce thick eggshells. The content of Fe in SMC2 (15.8 mg Fe g−1) was much higher than that in any other AMC, possibly due to Fe derived from the flocculant used for sewage treatment.
Table 1 Chemical properties of the animal manure composts used in this study
shows the P concentrations for each fraction obtained from the AMCs by means of sequential fractionation. The inorganic P in CMC, SMC, LMC and BLC accounted for 89, 93, 78 and 58% of total P, respectively. These results are consistent with those in previous studies, in which inorganic P accounted for more than 92% of the total in dairy compost (Sharpley and Moyer Citation2000), more than 87% in poultry compost (Sharpley and Moyer Citation2000) and more than 88% in cattle manure compost (Yokota et al. Citation2003). Two of the LMCs (LMC4 and LMC5) and all of the BLCs contained more Resid-P than the other AMCs, with values reaching more than 25% of total P.
Table 2 The fractionation of phosphorus (P) in the animal manure composts by sequential chemical extraction
Water-extractable P (H2O-P) and its proportion of total P obtained by the sequential fractionation differed among the manure types, although the differences were not significant because of the high variation (). The mean proportion of H2O-P in CMC was higher than in the other AMCs. The mean proportion of H2O-P in BLC (19% of the total) was lowest of all the composts because this manure had a high Resid-P (an average of 42% of total P). The mean proportion of H2O-P in LMC (20% of total P) was also low because of its high Ca content (155 mg Ca g−1; ), which would decrease P solubility (Ito et al. 2010). A high Ca content in AMC promotes the formation of less-soluble Ca phosphates such as hydroxyapatite. Swine manure composts had an intermediate proportion of H2O-P (an average of 28%) and the highest H2O-P (36 mg P g−1; ) among the four types of manure compost.
The proportion of P extracted by NaHCO3 (NaHCO3-P) was significantly greater for CMC (an average of 35%) than for the other composts. The concentration of NaHCO3-P was highest for SMC (an average of 7.3 mg P g−1), but its proportion of total P was significantly lower than that of CMC because SMC contained significantly higher HCl-extractable P (HCl-P). The concentrations of HCl-P in SMC and LMC were significantly greater than those of CMC and BLC. The proportions of HCl-P for SMC (an average of 42%) and LMC (an average of 46%) were also greater than those of CMC (an average of 16%) and BLC (an average of 33%), but were only significantly higher than those in CMC. HCl dissolves mainly apatite-type minerals (Williams et al. Citation1971) in the sequential fractionation. Toor et al. (Citation2005) found that hydroxyapatite formation accelerates in manures with a Ca/P ratio greater than 2, and the Ca/P ratios in the LMC used in the present study ranged from 2.8 to 6.5 (). The formation of hydroxyapatite therefore seemed likely to occur in LMC. On the other hand, CMC had a lower ratio of Ca/P (1.0–1.2) and a lower proportion of HCl-P, except for CMC4, which had a higher Ca concentration.
Continuous water extraction
The relationships between water volume and the cumulative amounts of P extracted from each AMC are shown in . and show the dissolution patterns of Ca and Mg, respectively. The dissolution patterns of P, Ca and Mg for all AMCs significantly fitted the first- or second-order kinetics models (). Because the kinetics models with the best fit differed even among the same type of manure, we adopted the model with the highest coefficient of determination (R2) for each element and AMC in our subsequent analysis. The wide ranges of WEPmax, WECamax and WEMgmax appear to relate to differences in the compositions and concentrations of the various chemical compounds among the AMCs.
Figure 2 The dissolution patterns of phosphorus (P) as a function of the cumulative water volume in the continuous water extraction. Curves represent the first-order and second-order regression equations; the best-fit models and the regression parameters are summarized in . CMC, cattle manure compost; SMC, swine manure compost; LMC, layer (chicken) manure compost; BLC, broiler (chicken) litter compost.
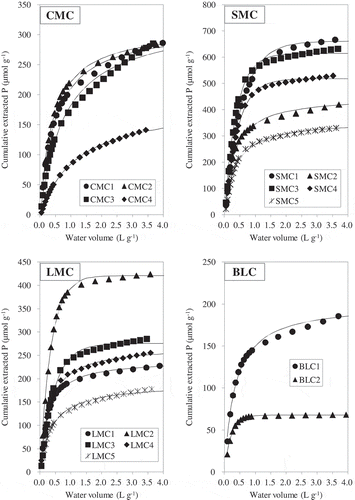
Figure 3 The dissolution patterns of calcium (Ca) as a function of cumulative water volume in the continuous water extraction. Curves represent the first-order and second-order regression equations; the best-fit models and the regression parameters are summarized in . CMC, cattle manure compost; SMC, swine manure compost; LMC, layer (chicken) manure compost; BLC, broiler (chicken) litter compost.
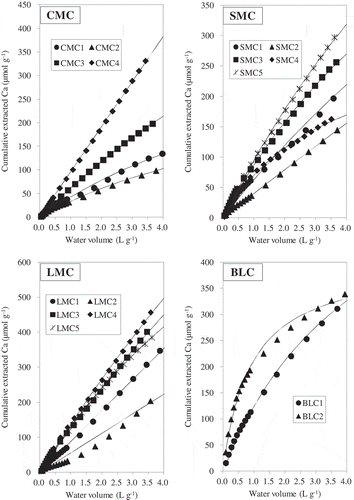
Figure 4 The dissolution patterns of magnesium (Mg) as a function of cumulative water volume in the continuous water extraction. Curves represent the first-order and second-order regression equations; the best-fit models and the regression parameters are summarized in . CMC, cattle manure compost; SMC, swine manure compost; LMC, layer (chicken) manure compost; BLC, broiler (chicken) litter compost.
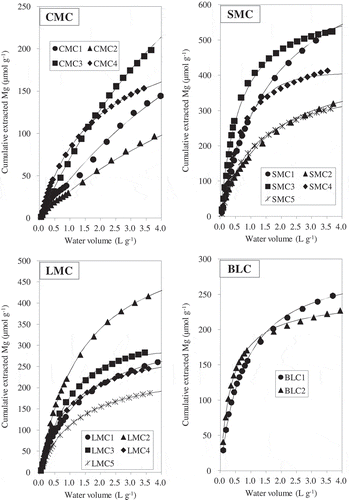
Table 3 Fitted values of the parameters used to model the phosphorus (P), calcium (Ca), magnesium (Mg) and potassium (K) release patterns, and the associated coefficient of determination (R2)
The values of WEPmax were 189–327 µmol g−1 (5.9–10.1 mg P g−1) for CMC, 362–661 µmol g−1 (11.2–20.5 mg P g−1) for SMC, 190–421 µmol g−1 (5.9–13.0 mg P g−1) for LMC, and 68–201 µmol g−1 (2.1–6.2 mg P g−1) for BLC. The proportions of WEPmax for CMC, SMC, LMC and BLC were 72 ± 11% (mean ± standard deviation), 46 ± 7%, 30 ± 3%, and 23 ± 9% of total P, respectively. In most of the AMCs, the amount of extractable P approximated its maximum when V reached 4.0 L g−1 (). The amounts of extractable P at 4.0 L g−1 accounted for 85 ± 6%, 97 ± 4%, 96 ± 4% and 96 ± 5% of WEPmax for CMC, SMC, LMC and BLC, respectively. The H2O-P determined by means of the single extraction amounted to an average of 63% of WEPmax ( and ). For the CMCs, WEPmax differed more from H2O-P obtained by means of single extraction than was the case for the other manure types, indicating that CMC contained more moderately soluble P compounds. For instance, the proportion of WEPmax accounted for by water-extractable P after water volume at 1.0 L g−1 was 39% for CMC, which was higher than in the other composts (17%, 15%, and 19% for SMC, LMC and BLC, respectively).
The release patterns of Ca for most samples were linear (), and differed from those of P and Mg ( and ). The linear dissolution patterns of Ca indicate that AMCs contain a lower concentration of soluble Ca compounds. Komiyama et al. (2013) showed that SMC contained calcite (calcium carbonate; CaCO3) as a crystalline compound by means of X-ray diffraction. Toor et al. (Citation2005) showed the existence of dicalcium phosphate (CaHPO4) and tricalcium phosphate [Ca3(PO4)2] in poultry manure by means of XANES spectroscopy. Hunger et al. (Citation2008) showed that poultry litter contained calcite and weddellite (calcium oxalate; CaC2O4 · 2H2O). These compounds have low solubility and dissolve gradually in water. The values of WECamax determined mathematically for several AMCs (CMC4, LMC1, LMC2; ) were higher than the total Ca contents (). In this experiment, the water volume was insufficient to determine the maximum amount of water-soluble Ca by fitting a kinetics model because the dissolution rate had not reached an asymptote in most AMCs at 4.0 L g−1.
The release patterns of Mg for most AMCs fitted the second-order kinetics model. Four composts (CMC1, CMC3, SMC4 and LMC3) fitted the first-order model (, ). The mean proportions of total Mg accounted for by WEMgmax for CMC, SMC, LMC and BLC were 81 ± 14%, 88 ± 12%, 84 ± 7%, and 82 ± 5%, respectively. These proportions were higher than the proportions of total P accounted for by WEPmax.
The release patterns of K for all AMCs fitted the first-order kinetics model, and the proportion of total K accounted for by WEKmax averaged 94% (data not shown). The release rate of K was faster than those of the other cations.
DISCUSSION
The maximum amount of WEP
shows the relationship between the H2O-P or the sum of H2O-P and NaHCO3-P determined using the sequential extraction method of Frossard et al. (Citation1994) and WEPmax. Linear regression analysis indicates that the values of WEPmax were significantly related to the values of H2O-P evaluated by a single water extraction (R2 = 0.846, P < 0.001) in this study. However, the slope of the linear regression was 1.70, indicating that the H2O-P determined by the single extraction method (a compost to water ratio of 1:200 w/v, 16-h extraction) potentially underestimate the water-soluble P. On the other hand, the sum of H2O-P and NaHCO3-P obtained by the sequential fractionation corresponded almost exactly to WEPmax (i.e., a slope of 0.90, with R2 = 0.978, in ). The averaged ratio of WEPmax to the sum of H2O-P and NaHCO3-P was 98%, indicating that the NaHCO3-extractable P potentially represents the water-soluble fraction. This suggests that the sum of H2O-P and NaHCO3-P provides a better indication of the WEPmax than using H2O-P alone. The continuous water extraction used in this study is a time-consuming method with special equipment. These results suggest that the maximum WEP can be rapidly estimated using the conventional sequential extraction method. In the NaHCO3 extraction, Ca and Mg carbonates and bicarbonates form, and the Ca and Mg phosphates are therefore easily dissolved. Ca and Mg phosphates extracted by NaHCO3 are thought to be present as amorphous forms in the AMC (Toor et al. Citation2006), and can be dissolved by large amounts of water. Josan et al. (Citation2005) found that continuous P release from sandy soils amended with a dairy manure was accompanied by the release of Ca and Mg in repeated water extraction experiments. The results obtained by Josan et al. (Citation2005) and Toor et al. (Citation2006) suggest the existence of poorly crystallized Ca and Mg phosphates in the manure or AMC.
Figure 5 The relationships between the H2O-P (open symbols) or H2O-P + NaHCO3-P (filled symbols) and the maximum water-extractable P (WEPmax). H2O-P and NaHCO3-P is water- and sodium bicarbonate (NaHCO3-) extractable P using the modified Hedley sequential extraction method. CMC, cattle manure compost; SMC, swine manure compost; LMC, layer (chicken) manure compost; BLC, broiler (chicken) litter compost. The dashed line represents y = x. ***, significant at P < 0.001.
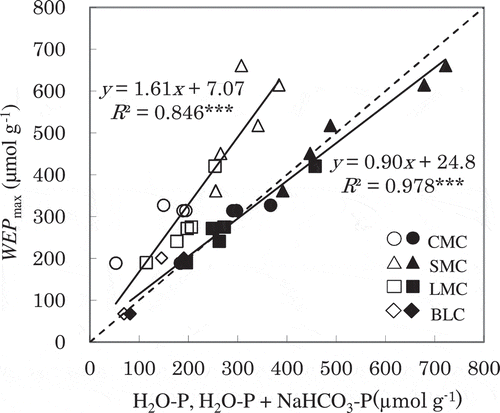
Relationship between P dissolution and K, Ca and Mg dissolution
To determine the phosphate species that were present in the WEP pools, we examined the relationships between the concentrations of P and of three cations that we extracted with a large volume of water. The values of WECamax were greater than the total Ca contents in several AMCs. We therefore compared the cumulative extractable Ca at 4.0 L g−1 with the WEPmax values because more than 95% of WEPmax was extractable when V reached 4.0 L g−1 in most AMCs. There was a significant but weak negative correlation (r = –0.492, P < 0.05) between the amount of extractable Ca at 4.0 L g−1 and WEPmax (). Some of the AMCs in the present study (all LMCs and CMC4) with a high water-soluble Ca concentration had low WEPmax values. The negative correlation between water-soluble Ca and WEPmax does not necessarily mean that water-soluble Ca phosphates were not present in the AMCs because Ca release may be greatly affected by the existence of CaCO3 in the AMCs, and this may obscure the relationship between Ca and P dissolution. Kleinman et al. (2005) reported a significant positive correlation between the water-extractable Ca and WEP in dairy cattle and swine manures, which contained higher levels of water-extractable Ca than the other manures that they studied.
Figure 6 The relationships between WEPmax and (left) the amount of extractable calcium (Ca) at 4.0 L g−1 or (right) WEMgmax. WEPmax, maximum amount of water-extractable phosphorus determined by means of non-linear regression; WEMgmax, maximum amount of water-extractable magnesium determined by means of non-linear regression. CMC, cattle manure compost; SMC, swine manure compost; LMC, layer (chicken) manure compost; BLC, broiler (chicken) litter compost. *, significant at P < 0.05; **, significant at P < 0.01.
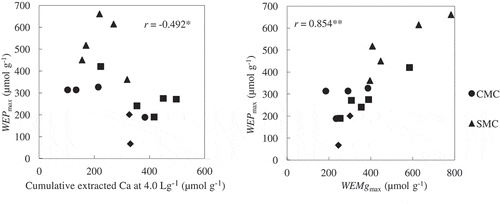
On the other hand, there was a strong and significant positive correlation (r = 0.854, P < 0.01) between WEMgmax and WEPmax. This suggests that WEPmax in the AMCs is closely related to the quantity of water-soluble Mg compounds. Kleinman et al. (2005) also showed a significant positive correlation between the water-extractable Mg and P in swine manures that had a higher content of water-extractable Mg (4.2 mg g−1) than the other manures that they studied. The values of WEMgmax for the SMCs in the present study (9.6–19.0 mg g−1) were greater than the water-extractable Mg of swine manure obtained by Kleinman et al. (2005). There was no significant correlation between WEPmax and WEKmax based on our data (data not shown). Because potassium phosphate is more easily soluble to water than calcium and magnesium phosphates, Ca or Mg is likely to combine more preferentially than K with phosphorus in the AMCs.
Harris et al. (Citation1994) showed that various components in manures [i.e., organic acids, Mg, silicon (Si)] inhibited the crystallization of stable calcium phosphates, thereby maintaining high P solubility in soil. Josan et al. (Citation2005) demonstrated the existence and dissolution of struvite (MgNH4PO4 · 6H2O), farringtonite [Mg3(PO4)2], and newberyite (MgHPO4 · 3H2O) in soils to which manure had been applied in a water extraction experiment and a chemical equilibrium modeling analysis. Tanahashi et al. (Citation2010) showed the existence of struvite in cattle and swine manure composts. Komiyama et al. (2013) also showed the existence and water solubility of struvite in a swine manure compost by means of X-ray diffraction analysis and chemical extraction. Hunger et al. (Citation2008) also found evidence for struvite in poultry litter. Tanikawa et al. (Citation1957) showed that the solubility of struvite at pH 9.0 was 0.02%, which is approximately equal to 200 mg L−1 (800 µmol L−1). This solubility of struvite exceeds the WEPmax of all AMCs in this study (), indicating that struvite can dissolve during continuous water extraction if it existed in the AMCs. The positive relationship that we found between P and Mg dissolved in water () corresponded with the results of the abovementioned studies that indicated the existence of magnesium phosphates in the AMCs.
CONCLUSIONS
We applied continuous water extraction and kinetics analysis to determine the concentrations of WEPmax in 16 AMCs. We found that WEPmax was greatest in SMC, followed by CMC, LMC and BLC. The WEPmax values were greater than the H2O-P determined by means of a single extraction (at a compost:water ratio of 1:200 w/v), but corresponded well to the sum of H2O-P and NaHCO3-P, indicating that NaHCO3-P is part of the water-soluble fraction. This suggests that WEPmax can be estimated from the sum of H2O-P and NaHCO3-P, which is obtained by means of conventional sequential chemical extraction. We found a significant positive correlation between WEMgmax and WEPmax (r = 0.854, P < 0.01). This relationship suggests that the amount of water-soluble P in the AMCs may be affected by water-soluble magnesium compounds.
REFERENCES
- Dahlgren RA, Walker WJ 1993: Aluminum release rates from selected Spodosol Bs horizons: effect of pH and solid-phase aluminum pools. Geochim. Cosmochim. Acta., 57, 57–66.
- Dou Z, Toth JD, Galligan DT, Ramberg CF, Ferguson JD 2000: Laboratory procedures for characterizing manure phosphorus. J. Environ. Qual., 29, 508–514.
- Eghball B, Power JF 1999: Phosphorus and nitrogen-based manure and compost application: corn production and soil phosphorus. Soil Sci. Soc. Am. J., 63, 895–901.
- Frossard E, Tekely P, Grimal JY 1994: Characterization of phosphate species in urban sewage sludges by high-resolution solid-state 31P-NMR. Eur. J. Soil Sci., 45, 403–408.
- Gérard-Marchant P, Walter MT, Steenhuis TS 2005: Simple models for phosphorus loss from manure during rainfall. J. Environ. Qual., 34, 872–876.
- Harris WG, Wang HD, Reddy KR 1994: Dairy manure influence on soil and sediment composition: implication for phosphorus retention. J. Environ. Qual., 23, 1071–1081.
- He Z, Honeycutt CW, Griffin TS 2003: Comparative investigation of sequentially extracted phosphorus fractions in a sandy loam soil and a swine manure. Commun. Soil Sci. Plant Anal., 34, 1729–1742.
- Hedley MJ, Stewart JWB, Chauhan BS 1982: Changes in inorganic and organic soil phosphorus fractions induced by cultivation practices and by laboratory incubations. Soil Sci. Soc. Am. J., 46, 970–976.
- Hunger S, Sims JT, Sparks DL 2008: Evidence for struvite in poultry litter: effect of storage and drying. J. Environ. Qual., 37, 1617–1625.
- Ito T, Komiyama T, Saigusa M, Morioka M 2010: Phosphate composition of swine and poultry manure composts. Jpn. J. Soil Sci. Plant Nutr., 81, 215–223. (In Japanese with English summary).
- Josan MS, Nair VD, Harris WG, Herrera D 2005: Associated release of magnesium and phosphorus from active and abandoned dairy soils. J. Environ. Qual., 34, 184–191.
- Kleinman PJA, Wolf AM, Sharpley AN, Beegle DB, Saporito LS 2005: Survey of water-extractable phosphorus in livestock manures. Soil Sci. Soc. Am. J., 69, 701–708.
- Komiyama T, Niizuma S, Fujisawa E, Morikuni H 2013: Phosphorus compounds and their solubility in swine manure compost. Soil Sci. Plant Nutr., 59, 419–426.
- Murphy J, Riley JP 1962: A modified single solution method for determination of phosphate in natural waters. Anal. Chim. Acta., 27, 31–36.
- Page AL, Miller RH, Keeney DR 1982: Methods of Soil Analysis Part 2—Chemical and Microbiological Properties. 2nd Edn. pp. 275–300. American Society of Agronomy, Madison, WI.
- SAS Institute Inc 2001: JMP user’s guide, version 4.0. J. SAS Institute, Cary, NC.
- Sharpley AN, Moyer B 2000: Phosphorus forms in manure and compost and their release during simulated rainfall. J. Environ. Qual., 29, 1462–1469.
- Tanahashi T, Yano H, Itou H, Oyanagi W 2010: Magnesium ammonium phosphate in cattle and swine manure composts and an extraction method for its evaluation. Jpn. J. Soil Sci. Plant Nutr., 84, 329–335. (In Japanese with English summary).
- Tanikawa E, Nagasawa Y, Sugiyama T 1957: The formation of magnesium-ammonium-phosphate crystals in canned sea foods: II. Solubility of struvite formed in canned sea foods and MgNH4PO4 · 6H2O crystals synthesized in laboratory. Bull. Fac. Fisheries Hokkaido Univ., 7, 300–305.
- Toor GS, Hunger S, Peak JD, Sims JT, Sparks DL 2006: Advances in the characterization of phosphorus in organic wastes: environmental and agronomic applications. Adv. Agron., 89, 1–72.
- Toor GS, Peak JD, Sims JT 2005: Phosphorus speciation in broiler litter and turkey manure produced from modified diets. J. Environ. Qual., 34, 687–697.
- Turner BL, Leytem AB 2004: Phosphorus compounds in sequential extracts of animal manures: chemical speciation and a novel fractionation procedure. Environ. Sci. Technol., 38, 6101–6108.
- Wienhold BJ, Miller PS 2004: Phosphorus fractionation in manure from swine fed traditional and low-phytate corn diets. J. Environ. Qual., 33, 389–393.
- Williams JDH, Syers JK, Harris RF, Armstrong DE 1971: Fractionation of inorganic phosphate in calcareous lake sediments. Soil Sci. Soc. Am. Proc., 35, 250–255.
- Yokota T, Ito T, Ono T, Takahashi M, Saigusa M 2003: Composition of inorganic phosphate in cattle manure compost with different production conditions. Jpn. J. Soil. Sci. Plant. Nutr., 74, 133–140. (In Japanese with English summary).
- Zuvomuya F, Helgason BL, Larney FJ, Janzen HH, Akinremi OO, Olsen BM 2006: Predicting phosphorus availability from soil-applied composted and non-composted cattle feedlot manure. J. Environ. Qual., 35, 928–937.