Abstract
In 2011, the radiocesium concentration of brown rice grown (Oryza sativa L.) in mountainous areas with irrigation water that flowed from a nearby mountain forest, in Fukushima Prefecture, Japan, exceeded 500 Bq kg–1. Migration of radiocesium from contaminated irrigation water was suspected. Here, we investigated the migration of dissolved radiocesium (137Cs) from irrigation water into brown rice by determining the concentration of 137Cs in brown rice grown in pots irrigated with water containing dissolved 137Cs (0.1, 1.0 or 10 Bq L–1 137Cs). The treatment water was prepared by extracting 137Cs from conifer branches collected from the contaminated area and diluting the stock solution with tap water. We used two Gray Lowland soils (soils A and B) collected from two paddy fields in Fukushima Prefecture. Rice plants (Oryza sativa L. cv. Hitomebore) were grown in pots and treatment water was kept at a depth of 3–5 cm throughout the growing season. We calculated the amount of 137Cs absorbed by the plants by subtracting 137Cs concentrations in brown rice grown with tap water from that in brown rice grown with treatment water. The concentration of 137Cs in brown rice increased in proportion to that in the treatment water. The concentration of 137Cs in brown rice grown with water containing 10 Bq L–1 137Cs in soil A was 4.9 times that in brown rice grown in soil B, but the concentration of 137Cs in brown rice grown in either soil was not significantly different when grown with water containing 0.1 Bq L–1 137Cs. Before plants were grown, the exchangeable potassium in soil B was 3.8 times that in soil A. At least, even with irrigation water containing about 0.1 Bq L–1 of radiocesium, the migration of radiocesium into brown rice is negligible for the provisional regulation value for radiocesium in brown rice under the condition of our experiment.
INTRODUCTION
The magnitude 9.0 earthquake and subsequent tsunami that occurred on March 11, 2011 in northeastern Japan caused major damage to the Fukushima Daiichi Nuclear Power Station (Tokyo Electric Power Company, Japan), resulting in large quantities of radionuclides being released into the environment. The air and soil in parts of eastern Japan were contaminated with the radionuclides cesium-134 (134Cs; half-life 2.07 years) and cesium-137 (137Cs; half-life 30.1 years; MEXT Citation2011). Fukushima Prefecture in particular was heavily contaminated (Kinoshita et al. Citation2011; AFFRC Citation2012).
Because radiocesium is adsorbed onto soil and binds strongly to clays, more than 90% of the fallout was distributed in the upper 6 cm of the soil in wheat fields, and in the upper 4 cm in rice paddies, orchards, and cedar forests (Ohno et al. Citation2012). Approximately 2 months after fallout deposition, 88% of the total radiocesium fallout (134Cs + 137Cs) was distributed within the upper 3 cm of the soil and 96% within the upper 5 cm in an undisturbed paddy field in Fukushima Prefecture (Shiozawa Citation2013). Before the disaster, the uptake of 137Cs from the top 20 cm to the aboveground biomass of rice plants was 0.003% of the soil inventory (Tsukada et al. Citation2008a). After the disaster, the uptake of 137Cs from the top 15 cm to the aboveground biomass of sunflower (Helianthus annuus L.), amaranth (Amaranthus L.), sorghum (Sorghum bicolor (L.) Moench), and buckwheat (Fagopyrum esculentum) was reported to be 0.013–0.093% of the soil inventory from a light-colored Andosol, and 0.007–0.038% from Gray Lowland soil (Suzuki et al. Citation2012). Recently, the soil-to-plant transfer factor for radiocesium was reported to be 0.048–0.170 for Amaranthus (Shinano et al. Citation2014).
Following the disaster at the power station, the Ministry of Agriculture, Forestry and Fisheries (MAFF), using data collected before the disaster and taking into account the safety coefficient of radiocesium, estimated the transfer indicator of the migration of radiocesium from soil to brown rice in Japan to be 0.1 (MAFF Citation2011). In 2011, the provisional regulation value for radiocesium (134Cs + 137Cs) in brown rice was set at 500 Bq kg–1. By using the transfer indicator, MAFF calculated the upper safe concentration limit for radiocesium in soil used for farming to be 5000 Bq kg–1 (mean soil concentration to a depth of 15 cm). However, in April 2012, the provisional regulation value for radiocesium in food was lowered from 500 to 100 Bq kg–1 (MHLW Citation2012).
After the 2011 harvest, the concentration of radiocesium in 0.02% of samples of brown rice grown in Fukushima Prefecture was found to be greater than the provisional regulation value of 500 Bq kg–1 (Fukushima Prefecture Citation2012a). The paddy fields in which these rice plants had been grown had several characteristics in common, including the fact that they were located near mountain forest and that they used irrigation water that flowed through the forest to the paddy field (Fukushima Prefecture Citation2012b). Migration of radiocesium into the rice plants from contaminated water flowing directly from the forest was suspected.
Although a number of studies examining the soil-to-plant migration of radiocesium have been conducted (Tsukada et al. Citation2002, Citation2008a; Komamura et al. Citation2006; Uchida et al. Citation2007), the mechanisms of this migration remain to be elucidated. Investigations have shown not only that the soil-to-plant migration of radiocesium can be limited by the application of potassium (K) to soil (NARO Citation2012; Kato Citation2012; Saito et al. Citation2012), but also that 4000 times more radiocesium translocates from water than from soil (Myttenaere et al. Citation1969). It has also been shown that irrigation water contains both suspended and dissolved radiocesium (Yasutaka et al. Citation2012), and that dissolved radiocesium migrates more easily into plants from water than from soil (Nemoto and Abe Citation2013). Radiocesium likely enters the agricultural water supply via irrigation ponds and forests, and the concentration of dissolved radiocesium in irrigation ponds and water from forests in Fukushima Prefecture was found to be ≤ 1 Bq L–1 (FFPRI Citation2012c; Ministry of the Environment Citation2013). Given these findings, it remains important to confirm the relationship between the concentration of radiocesium in irrigation water and that in brown rice grown with radiocesium-contaminated water.
Here, we examined the effects of low concentrations of radiocesium (137Cs) dissolved in irrigation water on the concentration of radiocesium in brown rice by growing rice plants under irrigation with either water spiked with 137Cs (0.1, 1.0, or 10 Bq L–1 137Cs) or one of two types of irrigation water obtained from a site in Fukushima Prefecture. We also examined the kinetics of radiocesium in a paddy field ecosystem.
MATERIALS AND METHODS
Preparation of treatment water and sampling of irrigation water
Conifer branches collected on May 24, 2012 in the northern part of the Hamadori region of Fukushima Prefecture, 45 km from the power station, were soaked in tap water (weight/volume, 1:50) and the branches and water were left outside at the outside temperature (18°C average temperature) for 13 d. The solution was then passed through a membrane filter (pore size, 0.45 μm; Thermo Scientific Nalgene Disposable Filter Unit; Thermo Fisher Scientific K.K., Kanagawa, Japan) and the filtrate was stored in a refrigerator (5°C) until analysis. After determining the radioactivity of the filtrate (970 Bq L–1 137Cs), we serially diluted the filtrate with tap water (0.027 Bq L–1 137Cs) to concentrations of 0.1, 1.0 or 10 Bq L–1 137Cs.
Two samples of irrigation water were collected from a field located in the northern part of the Nakadori region (the soil A site; see section Collection of soil samples), one on June 22, 2011 (WS1) after a heavy rain, the other on June 26, 2011 after fine weather (WS2). The samples were passed through a polyethylene net (pore size, 2 mm) to remove any large floating material.
Collection of soil samples
Two samples of Gray Lowland soil were collected from paddy fields in Fukushima Prefecture. One was collected from a field located in the northern part of the Nakadori region (soil A, collected on June 12, 2012), and the other was collected from a field at the Fukushima Agricultural Technology Center in Koriyama City (soil B, collected on June 14, 2012). At the 2011 harvest, the total concentration of radiocesium in brown rice grown in the soil A field was > 500 Bq kg–1, but that in brown rice grown in the soil B field was < 100 Bq kg–1. To minimize the migration of radiocesium from the soil as much as possible, we collected samples from the 15–45-cm layer of soil A and from the 5–45-cm layer of soil B.
Both soils were air-dried, passed through a 1-cm sieve and homogenized for 5 min in a fertilizer mixer (Hi Back Mazeta-kun B-180; Hokuetsu Co., Ltd., Niigata, Japan). The chemical properties of the soil samples before cultivation were determined and are shown in (Editorial Boards of Methods for Soil Environment Analysis Citation1997).
Table 1 Physical and chemical properties of the soils
Cultivation of rice plants
Rice seeds (Oryza sativa L. cv. Hitomebore) were sown in granular culture soil (Kumiai-Ryuujou-Baido; Sun Agri Co., Ltd., Tokyo, Japan) on June 6, 2012. Four seedlings per pot were transplanted into 4-L Wagner pots (diameter, 16 cm; height, 20 cm; Fujiwara Scientific Company Co., Ltd., Tokyo, Japan) containing 3.2 kg of one of the soils, on July 3, 2012. The pots were kept in a glasshouse (ventilated; temp, with > 20°C) at the Fukushima Agricultural Technology Centre, Koriyama, Japan. Three pots were planted for each treatment, and each experiment was conducted in triplicate. As fertilizer, 0.1 g nitrogen (Kumiai ammonium sulfate; Mitsubishi Chemical Corporation, Tokyo, Japan) and 0.087 g phosphorus (Kumiai-20 calcium superphosphate; Co-Op Chemical Co., Ltd., Tokyo, Japan) were applied to each pot as a basal dressing on July 3, 2012, and 0.24 g nitrogen (Kumiai ammonium sulfate; Mitsubishi Chemical Corporation) was applied as topdressing on August 4 and September 2, 2012. K was not applied.
In all experiments, enough treatment water, irrigation water (WS1, WS2) and tap water (control) was prepared to keep the water level in each pot at a depth of 3–5 cm throughout the growing season. In the experiments using treatment water, each pot used 21.3–23.5 L (1065–1175 L m−2) of irrigation water during the growth of the plants. In the experiments using irrigation water, each pot used 19.0–21.8 L (950–1090 L m−2).
Surface water-to-soil adsorption
To investigate the kinetics of radiocesium in a water–soil system, we placed dried soil samples (20.0 g) in polypropylene containers (100 mL; outer diameter, 5.0 cm; height, 9.5 cm), to which 100 mL of treatment water (200 Bq kg–1 dissolved 137Cs) was added with distilled water to dilute the filtrate (970 Bq kg–1 137Cs; see section Preparation of treatment water and sampling of irrigation water). The mixtures were left in the dark at 25°C, and the supernatant was sampled at 1, 24, 48, 72 and 168 h. The supernatants were passed through a membrane filter (pore size, 0.45 μm) and the concentration of dissolved 137Cs was determined.
Analysis of brown rice, soil and water samples
Brown rice and soil samples
Plants were harvested on October 3, 2012, and the brown rice was dried in a constant-temperature oven (DKN302; Yamato Scientific Co., Ltd., Tokyo, Japan) at 105°C for 2 d. The soil in the pots was then divided into the surface layer (0–5 cm) and the subsurface layer (5–15 cm). After air-drying at 25°C for 10 d, the soil samples were passed through a 2-mm sieve and homogenized for 5 min with stirring. The brown rice or soil samples were then compressed into separate cylindrical polystyrene containers (inner diameter, 5.0 cm; outer diameter, 5.6 cm; height, 6.8 cm) and the concentration of 137Cs was determined.
Exchangeable K was extracted from 2 g of soil samples by adding 20 mL ammonium acetate (CH3COONH4) solution (1 M, pH 7) and stirring for 1 h. Exchangeable 137Cs was extracted from 80 g of soil sample by adding 800 mL CH3COONH4 solution (1 M, pH 7) and stirring for 1 h (Tsukada et al. Citation2008b).
Irrigation water
To determine the amount of 137Cs (suspended and dissolved) in the samples of irrigation water, 20 L of irrigation water (WS1, WS2) was filtered through a 0.45-µm filter. Suspended matter on the filters was compressed into cylindrical polystyrene containers (inner diameter, 5.0 cm; outer diameter, 5.6 cm; height, 6.8 cm) for analysis. The filtrates were concentrated down to 2 L and then placed in 2-L Marinelli beakers (outer diameter, 17 cm; height, 19 cm) for analysis (MEXT Citation1982). The total concentration of 137Cs in WS1 was 1.4 Bq L–1 (dissolved 137Cs, 0.18 Bq L–1; suspended 137Cs, 1.2 Bq L–1). The total concentration of 137Cs in WS2 was 0.30 Bq L–1 (dissolved 137Cs, 0.17 Bq L–1; suspended 137Cs, 0.13 Bq L–1).
Tap water
To determine the low concentration of 137Cs in tap water, we captured dissolved 137Cs on nonwoven fabric (density, 130 g m−3; thickness, 0.85 cm; Japan Vilene Ltd., Tokyo, Japan) impregnated with Prussian blue (Dainichiseika Color & Chemicals Mfg. Co., Ltd., Tokyo, Japan), which absorbs radiocesium (Yasutaka et al. Citation2013). We passed 60 L of tap water through six columns (internal diameter, 65 mm; height, 100 mm), each containing seven Prussian blue-impregnated fabric discs, at a flow rate of 2.5 L min−1. The disks were collected from the columns, grouped as columns 1–3 and columns 4–6, and placed in cylindrical polystyrene containers for analysis. Under these conditions, the recovery rate of dissolved 137Cs was estimated to be about 90% (Yasutaka et al. Citation2013). We also determined the concentration of 137Cs after evaporating tap water to dryness; comparable values were obtained for both methods (MEXT Citation1982).
Absorption rate of dissolved 137Cs from irrigation water into brown rice
Absorption rate was used as a measure of the movement of dissolved 137Cs from the irrigation water into the brown rice, and was calculated as:
where WGi = brown rice grain weight in treatment i (kg dry wt.); CGi = concentration of 137Cs in brown rice in treatment i (Bq kg–1 dry wt.); WG0 = brown rice grain weight under control conditions; CG0 = concentration of 137Cs in brown rice under control conditions; VWi = volume of water applied during treatment i (L); and CWi = concentration of 137Cs in treatment water (Bq L–1). WG0 × CG0 represents the amount of 137Cs absorbed by the soil from the tap water.
Analytical equipment
The amount of K in samples was determined with an atomic absorption spectrophotometer (AA280FS; Agilent Technologies Japan, Ltd., Tokyo, Japan). The radioactivity of the samples was measured with a germanium semiconductor detector with multichannel analyzers (GC2020, GC3020, GC3520, GC4020, Canberra USA) over 4000–72,000 s for plant samples and extract solutions, 1200 s for soil samples, and 4000 s for supernatants. The concentration of 137Cs was decay-corrected back to the sample collection date (October 3, 2012) or time of treatment. The residual ratio of the supernatants was defined as the percentage ratio of the concentration of dissolved 137Cs remaining to the concentration of dissolved 137Cs in the treatment water.
Statistical analysis
All experiments were performed in triplicate with at least three independent repetitions. Values are expressed as mean ± SEM (standard error of the mean). Statistical analyses were conducted with R statistical software (version 2.10.1; R Foundation for Statistical Computing, Vienna, Austria). Differences between treatments by Student’s t-test or Tukey’s multiple range test at P < 0.05 were considered statistically significant.
RESULTS
The concentration of 137Cs in brown rice and soils irrigated with treatment water
When irrigated with water containing 0.1, 1.0 or 10 Bq L–1 137Cs, the concentration of 137Cs in brown rice grown in soil A was increased by 3.7, 40.4 and 308.8 Bq kg–1 dry weight, respectively, and that in soil B was increased by 0.5, 8.4 and 63.2 Bq kg–1 dry weight, respectively, compared with that in plants irrigated with tap water (). The increase in the concentration of 137Cs was statistically significant in brown rice grown in soil A irrigated with water containing 1 or 10 Bq L–1 137Cs, and in brown rice grown in soil B irrigated with water containing 10 Bq L–1 137Cs (). In brown rice irrigated with water containing 10 Bq L–1 137Cs, the increase in the concentration of 137Cs in the rice grown in soil A (308.8 Bq kg–1) was 4.9 times that in the rice grown in soil B (63.2 Bq kg–1). In rice plants irrigated with water containing 10 Bq L–1 137Cs, the water-to-plant 137Cs absorption rate in brown rice grown in soil A was 4.4 times that in brown rice grown in soil B ().
Table 2 Rate of absorption of radiocesium (137Cs) from treatment water by rice plants (Oryza sativa L. cv. Hitomebore)
Figure 1 Concentration of radiocesium (137Cs) in brown rice (Oryza sativa L. cv. Hitomebore) irrigated with water containing the indicated concentrations of 137Cs. Values are means ± SEM (standard error of the mean) (n = 3). Columns with the same letter are not significantly different at P < 0.05 (Tukey’s multiple range test).
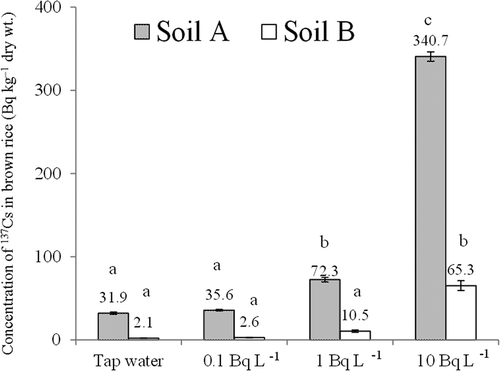
After crop growth, irrigation with water containing 10 Bq L–1 significantly increased the concentration of 137Cs in soil B (P < 0.05; ). The amount of exchangeable K in soil B was 2.4–2.8 times that in soil A; however, there were no significant changes in the amount of exchangeable K compared with control ().
Table 3 Exchangeable potassium (K) and radiocesium (137Cs) in the soils after crop growth
The distribution profile of 137Cs in both soils was also significantly changed after crop growth under irrigation with water containing 10 Bq L–1 137Cs (). After harvest, the concentration of 137Cs in the surface layer (0–5 cm) of both soil A and soil B was significantly increased (by 217.6 and 228.0 Bq kg–1, respectively) compared with that in the subsurface layer (5–15 cm). Furthermore, before crop growth, the concentration of exchangeable 137Cs in soil A and soil B was 12.5 ± 0.78 and 6.0 ± 0.44 Bq kg–1 (mean ± SEM), respectively; after irrigation with water containing 10 Bq L–1 137Cs, the concentration in the surface layer of soil B was significantly higher (8.9 Bq kg–1) than that in the subsurface layer ().
Figure 2 Concentration of total radiocesium (137Cs) and exchangeable 137Cs in soils irrigated with water containing the indicated concentrations of 137Cs. Values are means ± SEM (standard error of the mean) (n = 3). Surface soil, 0–5 cm; subsurface soil, 5–15 cm. *P < 0.05 (Student’s t-test) between surface and subsurface soil layers.
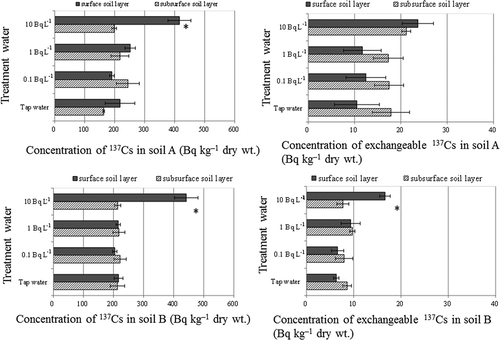
The concentration of 137Cs in brown rice and soils irrigated with collected irrigation water
The concentration of 137Cs in brown rice did not differ significantly after crop growth under irrigation with WS1 (obtained after heavy rain; total 137Cs concentration, 1.4 Bq L–1), WS2 (obtained after fine weather; 0.3 Bq L–1) or tap water (). The concentrations of 137Cs and exchangeable K in soil A also did not differ significantly after irrigation with WS1, WS2 or tap water ().
Table 4 Exchangeable potassium (K) and radiocesium (137Cs) in the soils after crop growth
Figure 3 Concentration of radiocesium (137Cs) in brown rice (Oryza sativa L. cv. Hitomebore) irrigated with water of different origins. Values are means ± SEM (standard error of the mean) (n = 3). WS1, irrigation water sampled on June 22, 2011 after heavy rain; WS2, irrigation water sampled on June 26, 2011 after fine weather; ns, not significantly different; P > 0.05 (Tukey’s multiple range test).
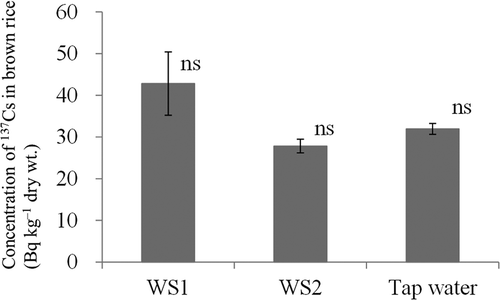
The distribution profile of 137Cs within soil A was also changed after crop growth under irrigation with the collected irrigation water. After irrigation with WS1, the concentration of 137Cs in the surface layer (0–5 cm) after irrigation with WS1 was significantly higher (44.8 Bq kg–1) than that in the subsurface layer (5–15 cm). Furthermore, after irrigation with WS2, the concentration of exchangeable 137Cs in the surface layer was significantly lower (9.8 Bq kg–1) than that in the subsurface layer ().
Figure 4 Concentration of total radiocesium (137Cs) and exchangeable 137Cs in soils irrigated with water of different origins. WS1, irrigation water sampled on June 22, 2011 after heavy rain; WS2, irrigation water sampled on June 26, 2011 after fine weather. Values are means ± SEM (standard error of the mean) (n = 3). Surface soil, 0–5 cm; subsurface soil, 5–15 cm. **P < 0.01 (Student’s t-test) between surface and subsurface soil layers.
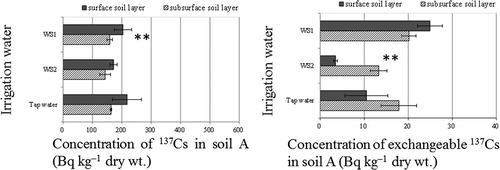
Residual ratio of supernatants after movement of 137Cs from water to soil
The residual ratio of dissolved 137Cs in the treatment water at 1, 24 and 48–168 h was 3.3%, 1.2% and < 1.0%, respectively, for soil A, and 4.5%, 3.8% and < 1.0%, respectively, for soil B ().
Table 5 Residual ratio of radiocesium (137Cs) in treatment water after adsorption to soil (%)
DISCUSSION
Radiocesium concentration in brown rice
In 2012, the concentrations of radiocesium were < 1 Bq L–1 in filtered stream water and < 0.1 Bq L–1 in irrigation water collected throughout Fukushima Prefecture (FFPRI Citation2012a, Citation2012b; Yasutaka et al. Citation2012). In the present study, irrigation with treatment water containing 0.1 Bq L–1 did not increase the concentration of 137Cs in brown rice compared with tap water. However, the concentration of 137Cs in brown rice irrigated with water containing 1 Bq L–1 137Cs was increased in soil A ().
When plants were irrigated with water containing increasing concentrations of 137Cs, the concentration of 137Cs in the brown rice grown in both soils increased (). Therefore, the concentration of 137Cs in brown rice is clearly related to the concentration of 137Cs in the irrigation water ().
Figure 5 Relationship between the concentration of radiocesium (137Cs) in brown rice and that in treatment water. Values are means ± SEM (standard error of the mean) (n = 3). *P < 0.05 (Pearson’s product-moment correlation coefficient).
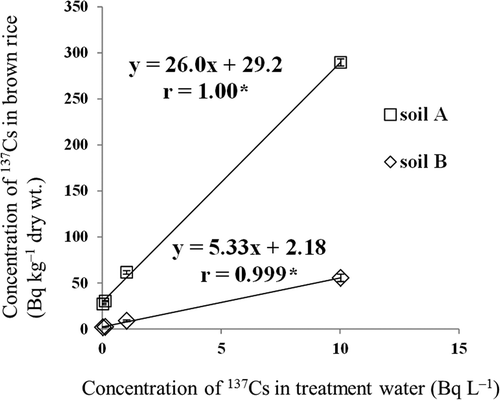
shows that when irrigated with water containing 1.4 Bq L–1 dissolved 137Cs (same concentration as in WS1), the concentration of 137Cs in brown rice grown in soil A should be increased by 56.6 (40.4 × 1.4) Bq kg–1 dry weight. However, when brown rice was grown under irrigation with WS1, the increase in the concentration of 137Cs was not significant (). This is likely attributable to the fact that WS1 contained both suspended 137Cs and dissolved 137Cs. Furthermore, the concentrations of 137Cs and exchangeable K in soil A were not changed after irrigation with WS1, WS2 or tap water (). The reason why the concentration of 137Cs in the surface layer after irrigation with WS1 was significantly higher than that in the subsurface layer, and why the exchangeable 137Cs in the surface layer after irrigation with WS2 was significantly lower than that in the subsurface layer, is likely related to the presence or absence of suspended 137Cs (). Therefore, the total amount of 137Cs that migrated into plants from the samples of irrigation water (suspended + dissolved 137Cs) was less than what migrated from the treatment water (dissolved 137Cs only). These data imply that the likelihood of the concentration of 137Cs in brown rice exceeding > 100 Bq kg–1 due to growth under irrigation with water containing low levels of dissolved radiocesium is low for both soil types.
The data also suggest that most 137Cs in irrigation water is adsorbed within the upper 5 cm of the soil (), and that > 95% of the 137Cs dissolved in the treatment water was adsorbed onto both soils within 1 h (). Therefore, most of the 137Cs in the irrigation water was adsorbed immediately to the surface soil layer.
Radiocesium concentration and exchangeable K in soil after harvest
The concentration of 137Cs in brown rice irrigated with treatment water containing 10 Bq L–1 and grown in soil A (308.8 Bq kg–1) was 4.9 times that in brown rice grown in soil B (63.2 Bq kg–1; ). Before crop growth, the amount of exchangeable K in soil B was 3.8 times that in soil A (), but after harvest, it was only 2.4–2.9 times that in soil A (). Application of K to soil inhibits the soil-to-plant migration of radiocesium (Kato Citation2012; NARO Citation2012; Saito et al. Citation2012). Therefore, it is likely that the exchangeable K in soil B inhibited the migration of 137Cs from the irrigation water into the brown rice (). These results confirm that a high level of exchangeable K in soil can limit the transfer of 137Cs from irrigation water containing low levels of 137Cs (0.1–1.0 Bq L−1).
Furthermore, our results show that irrespective of the amount of exchangeable K in the soil, the migration of 137Cs from irrigation water containing low levels of 137Cs (< 0.1 Bq L–1) into brown rice is limited. At least, even with irrigation water containing about 0.1 Bq L–1 of radiocesium, the migration of radiocesium into brown rice is negligible for the provisional regulation value for radiocesium in brown rice under the conditions of our experiment.
ACKNOWLEDGMENTS
This study was supported in part by the National Institute of Advanced Industrial Science and Technology, Japan. The authors would like to thank the Ministry of Agriculture, Forestry and Fisheries, Fukushima Agricultural Technology Centre Analysis Division, Isotope Research Institute, Inc., Japan Environment Science Co., Ltd. and EAC Corporation for their support with the analyses.
REFERENCES
- AFFRC 2012: http://www.s.affrc.go.jp/docs/map/pdf/02_2_00bunpu_zenken.pdf (in Japanese December, 2013)
- Editorial Boards of Methods for Soil Environment Analysis 1997: Methods for Soil Environment Analysis, Hakubunkan Shinsha Publishers Press, Tokyo.
- FFPRI 2012a: http://www.ffpri.affrc.go.jp/press/2012/20120612/documents/20120612.pdf (in Japanese December, 2013)
- FFPRI 2012b: http://www.ffpri.affrc.go.jp/press/2012/20120921/documents/20120921.pdf (in Japanese December, 2013)
- FFPRI 2012c http://www.ffpri.affrc.go.jp/press/2012/20121220/documents/20121220.pdf (in Japanese July, 2014)
- Fukushima Prefecture 2012a: http://wwwcms.pref.fukushima.jp/download/1/suiden_kinkyu_120203_torimatome_0207teisei.pdf (in Japanese December, 2013)
- Fukushima Prefecture 2012b: http://www.pref.fukushima.jp/keieishien/kenkyuukaihatu/gijyutsufukyuu/05gensiryoku/240112_tyukan.pdf (in Japanese December, 2013)
- Kato N 2012: Countermeasures to reduce radiocaesium contamination in paddy rice, soybean and cabbage. In International Science Symposium on combating radionuclide contamination in Agro-soil environment, Fukushima, Japan. pp. 317–318
- Kinoshita N, Sueki K, Sasa K et al. 2011: Assessment of individual radionuclide distributions from the Fukushima nuclear accident covering central-east Japan. Proc. Natl. Acad. Sci., 108(49), 19526–19529. doi:10.1073/pnas.1111724108
- Komamura M, Tsumura A, Yamaguchi N, Fujiwara H, Kihou N, Kodaira K 2006: Long-term Monitoring and Analysis of 90Sr and 137Cs concentrations in rice, wheat and soils in Japan from 1959 to 2000. National Institute for Agro-Environmental Sciences Report 24, pp. 1–21 (in Japanese with English summary)
- MAFF 2011: http://www.maff.go.jp/j/press/seisan/sien/pdf/110422-03.pdf (in Japanese December, 2013)
- MEXT 1982: Radioactivity measurement. In Series No. 13., p. 6. Japan Chemical Analysis Center Press, Chiba (in Japanese)
- MEXT 2011: http://radioactivity.nsr.go.jp/ja/contents/4000/3710/24/1305820_20110506.pdf (in Japanese July, 2014)
- MHLW 2012: http://www.mhlw.go.jp/shinsai_jouhou/dl/tuuchi_120316.pdf (in Japanese March, 2014)
- Ministry of the Environment 2013: http://www.env.go.jp/jishin/monitoring/result_pw130809-3.pdf (in Japanese July, 2014)
- Myttenaere G, Bourdeau P, Masset M 1969: Relative importance of soil and water in the indirect contamination of flooded rice with radiocaesium. Health Phys., 16, 701–707. doi:10.1097/00004032-196906000-00004
- NARO 2012: http://www.naro.affrc.go.jp/publicity_report/press/laboratory/narc/027913.html (in Japanese March, 2014)
- Nemoto K, Abe J 2013: Radiocesium absorption by rice in paddy field ecosystems. In Agricultural Implications of the Fukushima Nuclear Accident, Ed. Nakanishi TM, Tanoi K, pp. 19–27. Springer Japan, Tokyo.
- Ohno T, Muramatsu Y, Miura Y, Oda K, Inagawa N, Ogawa H, Yamazaki A, Toyama C, Sato M 2012: Depth profiles of radioactive cesium and iodine released from the Fukushima Daiichi nuclear power plant in different agricultural fields and forests. Geochem J., 46, 287–295. doi:10.2343/geochemj.2.0204
- Saito T, Ohkoshi S, Fujimura S et al. 2012: effect of potassium application on root uptake of radiocesium in rice. In Proceedings of international symposium on environmental monitoring and dose estimation of residents after accident of TEPCO’s Fukushima Daiichi Nuclear Power Station, pp. 165–169. Kyoto University Research Reactor Institute Press. Kyoto.
- Shinano T, Watanabe T, Chu Q, Osaki M, Kobayashi D, Okouchi T, Matsunami H, Nagata O, Okazaki K, Nakamura T 2014: Varietal difference in radiocesium uptake and transfer from radiocesium deposited soils in the genus Amaranthus. Soil Sci. Plant Nutr., 1–9. http://www.tandfonline.com/doi/full/10.1080/00380768.2014.922035#.U_B8V7kcSUk
- Shiozawa S 2013: Vertical migration of radiocesium fallout in soil in Fukushima. In Agricultural Implications of the Fukushima Nuclear Accident, Ed. Nakanishi TM, Tanoi K, pp. 49–60. Springer, Japan Tokyo.
- Suzuki Y, Saito T, Tsukada H 2012: Phytoremediation of radiocesium in different soils using cultivated plants. In Proceedings of international symposium on environmental monitoring and dose estimation of residents after accident of TEPCO’s Fukushima Daiichi Nuclear Power Station, pp. 170–173. Kyoto University Research Reactor Institute Press. Kyoto.
- Tsukada H, Hasegawa H, Hisamatsu S, Yamasaki S 2002: Transfer of 137Cs and stable Cs from paddy soil to polished rice in Aomori, Japan. Journal of Environmental Radioactivity, 59, 351–363. doi:10.1016/S0265-931X(01)00083-2
- Tsukada H, Takeda A, Hasegawa H 2008a: Uptake and distributions of 90Sr and 137Cs in rice plants. 16th pacific Basin Nuclear Conference, Aomori, Japan, Paper ID P16P1121.
- Tsukada H, Takeda A, Hisamatsu S, Inaba J 2008b: Concentration and specific activity of fallout 137Cs in extracted and particle-size fractions of cultivated soils. J. Environ. Radioact., 99, 875–881. doi:10.1016/j.jenvrad.2007.11.014
- Uchida S, Tagami K, Hirai I 2007: Soil-to-plant transfer factors of stable elements and naturally occurring radionuclides: (2) rice collected in Japan. J. Nucl. Sci. Technol., 44(5), 779–790. doi:10.1080/18811248.2007.9711867
- Yasutaka T, Kawabe Y, Kurosawa A, Komai T 2012: Monitoring dissolved radioactive cesium in Abukuma River in Fukushima prefecture. In Proceedings of International Symposium on Environmental monitoring and dose estimation of residents after accident of TEPCO’s Fukushima Daiichi Nuclear Power Station, pp. 137–140. Kyoto University Research Reactor Institute Press. Kyoto.
- Yasutaka T, Kawamoto T, Kawabe Y, Sato T, Sato M, Suzuki Y, Nakamura K, Komai T 2013: Rapid measurement of radiocesium in water using a Prussian blue impregnated nonwoven fabric. J. Nucl. Sci. Technol., 50, 674–681. doi:10.1080/00223131.2013.797936