Abstract
Bamboo has been introduced to coastal sandy areas in southeastern China to protect and restore coastal ecosystems. An understanding of the chemistries and enzymatic and microbial activities in the soils of these bamboo forests will aid our understanding of how bamboo plantations can improve soil fertility and will provide scientific evidence for policy makers for encouraging the planting of bamboo in other coastal areas. We investigated the physical and chemical properties of the rhizosphere soil [soil moisture content (SMC), pH, and contents of soil organic matter (SOM), total nitrogen (TN), available nitrogen (AN), total phosphorus (TP), available phosphorus (AP), total potassium (TK) and available potassium (AK)], enzymatic activities (sucrase, protease, urease and catalase) and microbial properties (counts of bacteria, fungi and actinomycetes) in five bamboo forests. The bamboo forests had significantly higher levels of SOM, TN, AN, TP, AP, TK, and AK and lower pH relative to a control soil sample from an area devoid of plants. Soil enzymatic activities and microbial communities were considerably higher in the bamboo forests than in the soil from the barren land. The chemical contents, enzymatic activities and microbial counts of the soil and the litter and root biomasses were higher in forests with the bamboo species Dendrocalamopsis oldhami and Pseudosasa amabilis than in forests with the other three species (Acidosasa edulis, Dendrocalamopsis vario-striata, and Dendrocalamopsis beecheyana var. pubescens), which suggests that these two species could adapt to sandy soil and grow well in a hostile environment. These results indicate that planting bamboo may help to both enrich soil fertility and increase the diversity of tree species in coastal ecosystems. The difference between aboveground and belowground biomass may have been responsible for these changes in soil properties.
INTRODUCTION
Bamboo is a group of productive evergreen plants belonging to the subfamily Bambusoideae of the grass family Gramineae, with approximately 1500 known species in 87 genera around the world. Bamboo is widespread and important in the subtropical and tropical regions of Asia, Africa and Latin America (Li and Kobayashi Citation2004; Zhou et al. Citation2011). China has the richest diversity of bamboo, with over 500 species in 39 genera distributed in more than 25 provinces throughout China, with half of the species in the provinces of Fujian, Jiangxi and Zhejiang (Cao et al. Citation2011; Zhou et al. Citation2011). Bamboo plants propagate, grow and regenerate rapidly. Once established, rapid growth and clonal reproduction increase bamboo’s ability to compete for light and space by forming dense stands that can be harvested long-term for timber and bamboo shoots (Silveira Citation2005). Bamboo’s special biological characteristics and growth habits enable bamboo forests to serve ecological and environmental functions such as land rehabilitation, water conservation and control of soil erosion (Zhou et al. Citation2005). Bamboo has thus been introduced into coastal sandy areas as an effective measure of increasing the diversity of tree species in coastal shelter belts (Zhang et al. Citation2007).
Sandy areas on the southeastern coast of China are generally characterized by poor fertility, salt spray, high soil salinity and drought. This area is subtropical with plentiful rainfall and high temperatures, but soil developed from sand has a poor capacity for retaining water and nutrients, which limits the biota of the soil and the establishment of forests (Ishikawa et al. Citation1995; Chen et al. Citation2005; Chang et al. Citation2011). The dominant vegetation is Casuarina equisetifolia, which was established more than 50 years ago to stabilize sand dunes and to protect inland vegetation. C. equisetifolia, however, is difficult to regenerate, matures quickly and declines in growth rate, which has plagued the development of coastal shelter belts (Xu Citation2005). An effective solution for achieving the sustainable management of coastal shelter belts must therefore be found, an urgent task indeed.
Bamboo was introduced to coastal sandy soils as a new tree species of shelter belts. Some studies have investigated biomass structure, dynamics of litter decomposition and nutrient content of bamboo forests in coastal sandy areas (e.g. Zheng et al. Citation2008), but soil enzymatic and microbial activities have only been measured in the soil of a C. equisetifolia forest (Chang et al. Citation2011). Previous research has shown that the practices of intensively managed bamboo plantations have a negative influence on the biological properties of soils (Xu et al. Citation2008). Soil properties in a Phyllostachys pubescens forest have been studied (Zhang et al. Citation2010), but evidence of this issue in bamboo forests in coastal ecosystems is scarce. We aimed to assess soil enzymatic activities and microbial properties and their relationships with soil physicochemical characteristics and plant litter in five bamboo forests in a coastal ecosystem in southeastern China. We hypothesized that the introduction of bamboo positively affects soil microbial and enzymatic activities. We tested this hypothesis by addressing three scientific questions: (1) do soil enzymatic activities differ among various bamboo forests, which vary in the quantity and quality of litter; (2) how do bamboo forests influence the structure of soil microbial communities, and (3) does the introduction of bamboo improve the fertility of sandy soil? To answer these questions, we investigated five bamboo forests in a coastal sandy area.
MATERIALS AND METHODS
Study site
The study site was a coastal forest at the Chishan Forest Farm (23°47'N, 117°18'E), Dongshan County, Fujian province, southeastern China. The climate is classified as subtropical oceanic monsoon of the south subtropical zone. The mean annual temperature is 20.9°C, the extreme high temperature is 39.8°C and the extreme low temperature is –3°C. The mean precipitation is 1103 mm, with 60–80% occurring from March to October. The sandy soil has excellent air and moisture permeability but low capacities to retain nutrients and water. Natural vegetation is scarce, with the dominant vegetation, C. equisetifolia, having been established for more than 50 years. The understory consists of scattered shrub species, such as Cajanus cajan, Spinifex littoreus and Vitex negundo.
Soil sampling and analysis
The study area was established 1 km inland from the beach. Samples were collected from five bamboo forests. The sites (approximately 667 m2 each) were artificial, pure forests of the bamboo species Pseudosasa amabilis, Acidosasa edulis, Dendrocalamopsis vario-striata, D. oldhami and D. beecheyana var. pubescens planted in 2000–2002. The tree density of each species was approximately 600 trees per ha, with the trees spaced in grids of 4 × 4 m. The plantations were fertilized (in circular trenches 30 cm in depth, with 30 cm around each tree) three times per year (March, June and September) in 2003 and 2004. The total rates of chemical fertilizer were 3.6, 2.4 and 1.2 kg ha−1 for urea (N), superphosphate (P), and potassium chloride (K), respectively. The amount of all fertilizers applied was 43.2 kg ha−1 during these 2 years.
In the summer of 2011, two plots (10 × 10 m) were selected for each forest. All forests were sympodial bamboo. describes the properties of the five forests. Within each plot, samples of rhizosphere soil were collected with a stainless-steel shovel near five randomly selected trees () and at three randomly selected points (20 cm in diameter, 0–20 cm deep) for each tree. The samples from the three points were bulked to produce one sample for the tree. A total of 10 soil samples were thus collected from each forest. A soil sample collected from barren land devoid of plants was also collected and served as a control.
Table 1 Characteristics of the five bamboo forests [means ± standard deviation (SD)].
Figure 1 Sampling at the site, which was 10 × 10 m, and the space of the bamboo species was 4 × 4 m. The sampled trees are represented by.
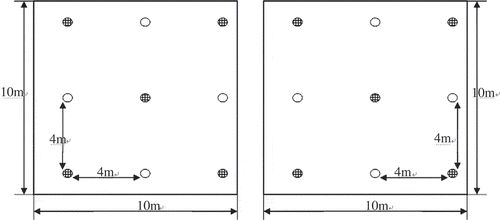
Samples of the rhizosphere soil were collected following the method proposed by Riley and Barber (Citation1971). Roots were carefully removed from the soil, gently shaken to dislodge the soil loosely adhering to the roots (within a maximum of 2 mm from the root surface), and the rhizosphere soil was collected by carefully separating it from the roots with a paintbrush.
Plant roots and litter were manually removed before sieving. Each sample was separated into three portions. The first portion was air dried for chemical analysis and sieved through a 2-mm sieve to obtain representative subsamples, and a quarter of the subsample sieved fraction subsequently was ground in an agate mortar to pass a through a 0.149-mm sieve (100-mesh). The 2-mm fraction was used for the analysis of pH, available nitrogen (AN), available phosphorus (AP) and available potassium (AK). The 0.149-mm fraction was used for the analysis of soil organic matter (SOM), total nitrogen (TN), total phosphorus (TP) and total potassium (TK). The second portion (air dried) was used for soil enzymatic studies and was sieved to 1 mm to remove small litter fragments. The third portion (fresh soil) was stored at 4°C immediately after collection and was used for microbiological analysis.
Soil physical and chemical analysis
Soil moisture content (SMC) was determined by weighing fresh soil samples before and after oven drying at 105°C for 24 h to a constant weight (LY/T 1213–1999). The pH of each soil core was measured at a soil: water ratio of 1:2.5 (LY/T 1239–1999). SOM content was measured by the classical method of potassium dichromate oxidation-outer heating (LY/T 1237–1999). TN content was evaluated by the semi-micro Kjeldahl method (LY/T 1228–1999). AN content was measured by a sodium hydroxide (sodium hydroxide (NaOH)) hydrolytic diffusion method (LY/T 1229–1999). TP content was determined by molybdenum antimony D-iso ascorbic acid colorimetry (MADAC) (LY/T 1232–1999). AP content was measured by hydrochloric acid/sulfuric acid (HCl/H2SO4) extraction (LY/T 1233–1999). TK content was estimated by means of alkali fusion-flame photometry (LY/T 1234–1999). AK content was measured by acetic ammonium extraction flame photometry (LY/T 1236–1999). The physical and chemical experiments were determined for three replicates.
Soil enzymatic activity
Four enzymes were selected for analysis: sucrase, protease, urease and catalase. They were assayed by methods previously described (Guan Citation1986), and the results were based on three replicates. Sucrase activity was measured as the volume (mL) of 0.1 N sodium thiosulfate (Na2S2O3) g−1 soil which resulted from the enzymatic degradation of adding sucrose with an incubation period of 24 h at 37°C. Protease activity was calculated as the mass (mg) of amine nitrogen (NH2-N) g−1 soil which resulted from the enzymatic degradation of adding casein with an incubation period of 24 h at 37°C. Urease activity was estimated as the mass (mg) of ammonia nitrogen (NH3-N) g−1 soil which resulted from the enzymatic degradation of adding urea with an incubation period of 24h at 7°C. Catalase activity was expressed as the volume (mL) of 0.1 N potassium permanganate (KMnO4) g−1 soil which resulted from the enzymatic degradation of adding hydrogen peroxide with a rocking incubation period of 20 minutes at 37°C.
Soil microbiological analysis
The populations of bacteria, fungi and actinomycetes were quantified by dilution plate-count techniques on a range of culture media for microorganisms (Zhang et al. Citation2010). Five grams of rhizosphere soil were aseptically weighed and transferred to flasks containing 45 mL sterile water, which were shaken for 10 min at 200 rpm on a rotary shaker. While the suspension was in motion, 5 mL were withdrawn and added to 45 mL sterile water in a screw-cap flask and shaken for 1 min; 5 mL of this suspension were transferred to 45 mL sterile water, and the process was repeated to a final dilution of 1:100 000. The dilutions from 1:1000 to 1:100 000 were spread onto plates containing nutrient agar, potato dextrose agar and modified Gauo’s 1 agar, for bacteria, fungi and actinomycetes, and incubated at 25°C for 3, 2 and 5 d, respectively. After the incubation, colonies were counted. The microbial populations were determined for five replicates.
Sampling and analysis of plant litter
The aboveground litter from each plot was collected from five randomly placed 50 × 50 cm quadrats, and also excavated to a depth of 20 cm to obtain root biomass. The collected litter was cleaned with a soft brush in the laboratory before oven drying at 65°C to a constant weight, and the root biomass was cleaned with water and oven dried at 65°C to a constant weight.
Statistical analysis
All statistical analysis was performed using SPSS v. 17.0 (SPSS Inc., Chicago, USA). Tests for significant differences of soil properties and litter and root biomasses were evaluated by one-way analyses of variance (ANOVA), and least significant differences (LSD) were determined for multiple comparisons. The relationships among soil properties, soil enzymes, soil microbial parameters, and litter and root biomasses were determined by Pearson correlations. The significance level was set at p < 0.05.
RESULTS
Soil physicochemical parameters
SMC and the nutrient contents of the soil from the sites were significantly higher than in the control, but the soils were lower in pH (). SMC differed significantly among the five sites. SMC and AP and AK contents were highest in the plots of A. edulis (, , i). SOM and TP contents were higher for D. oldhami than for the other species, and SOM content did not differ significantly among P. amabilis, A. edulis, D. vario-striata and D. beecheyana var. pubescens (). TN, AN and TK contents were highest for P. amabilis (, , h). TN content did not differ significantly among A. edulis, D. vario-striata, D. oldhami and D. beecheyana var. pubescens, but AN and TK contents did (, ).
Figure 2 Soil physicochemical characteristics of various bamboo forests (A = P. amabilis, B = A. edulis, C = D. vario-striata, D = D. oldhami, E = D. beecheyana var.pubescens, F = bare land). Error bars are standard deviation (SD). Different letters indicate significant differences (P < 0.05). SMC: soil moisture content, SOM: soil organic matter, TN: total nitrogen, AN: available nitrogen, TP: total phosphorus, AP: available phosphorus, TK: total potassium, AK: available potassium.
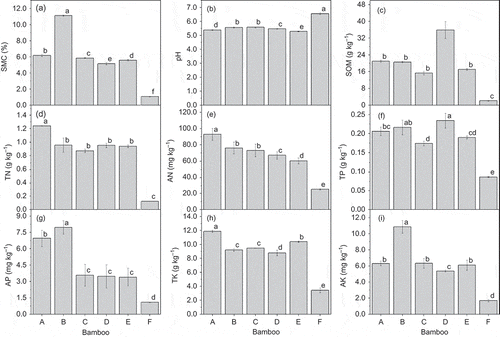
Enzymatic activity in soil
The activities of the soil enzymes for the various bamboo plots were significantly higher than those of the control (). Catalase activity significantly decreased in the order A. edulis > D. vario-striata > D. oldhami > D. beecheyana var. pubescens > P. amabilis (). Sucrase and protease activities, as with SOM and TP contents, were both higher for D. oldhami than for the other four species ( and ). However, no significant difference was found in the protease activity of P. amabilis, A. edulis and D. vario-striata compared to D. oldhami, as was observed in the sucrase activity. Urease activity was highest for P. amabilis () and differed only slightly among A. edulis, D. vario-striata, D. oldhami and D. beecheyana var. pubescens.
Figure 3 Soil enzyme activity of various bamboo forests (A = P. amabilis, B = A. edulis, C = D. vario-striata, D = D. oldhami, E = D. beecheyana var.pubescens, F = bare land). Error bars are standard deviation (SD). Different letters indicate significant differences (P < 0.05). Na2S2O3: sodium thiosulfate; NH2-N:amine nitrogen; NH3-N:ammonia nitrogen; KMnO4: potassium permanganate.
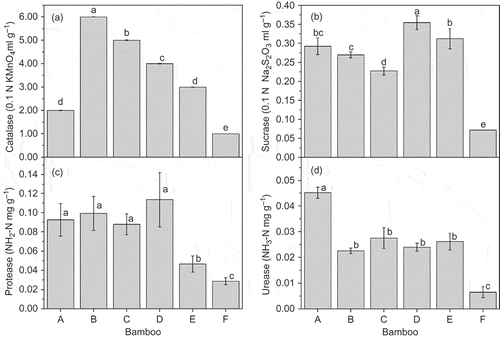
Soil microbiological properties
The populations of bacteria, fungi and actinomycetes were considerably higher in the bamboo plots than in the barren land. The total amount of microorganisms of D. oldhami was greater than the other four bamboo species. No significant differences were found among D. oldhami, P. amabilis and D. beecheyana var. pubescens (). The bacterial counts were highest for D. oldhami, but only slight differences were observed among the five species (). Counts for both fungi and actinomycetes were highest for D. beecheyana var. pubescens. Fungal counts showed significant differences among species, and the actinomycetes count did not significantly differ between D. oldhami and A. edulis or between P. amabilis and D. vario-striata ( and ).
Above- and belowground biomass
Aboveground litter biomass decreased in the following order: D. oldhami > P. amabilis > D. beecheyana var. pubescens > D. vario-striata > A. edulis. Litter biomass ranged from 32.96 to 73.20 g m−2. Aboveground biomass, however, did not differ significantly between D. vario-striata and A. edulis (). Root biomass was highest for D. oldhami, followed by P. amabilis. Root biomass did not differ significantly between D. vario-striata and D. beecheyana var. pubescens ().
Relationships among the physical, chemical, enzymatic and microbial properties of the soils
pH was negatively correlated with TN, AN, TP and TK contents, sucrase and catalase activities, bacterial and fungal counts, and root biomass (). Positive significant correlations were found between SMC and AP and AK contents and catalase activity. SOM was positively correlated with TP content, sucrase and protease activities, bacterial count, and litter and root biomasses. Positive significant correlations were observed between TN and AN, TP and TK contents, sucrase and urease activities, bacterial count, and litter and root biomasses. AN was positively correlated with TP, AP, TK, and AK contents, urease activity, bacterial count and root biomass. Positive significant correlation was determined between TK and sucrase and urease activities, bacterial count and root biomass. Sucrase activity was positively correlated with bacterial and fungal counts and litter and root biomasses. Protease activity was positively correlated with root biomass. Bacterial count was positively correlated with fungal count and litter and root biomasses.
Table 2 Pearson correlation coefficients between soil property, soil enzyme and soil microbial variables.
DISCUSSION
Physicochemical characteristics in soil
pH is a crucial factor determining soil fertility and can be strongly influenced by cultivated plants (Yan et al. Citation1996). Our study showed that the pH of the soils in the bamboo forests were lower than the pH of the soil from the barren land, consistent with studies that have found soil pH to be decreased by the cultivation of plants (Williams Citation1980; Mengel and Steffens Citation1982). The decrease in soil pH may be due to the release of protons by root organic anions such as malate, citrate and oxalate (Bolan et al. Citation1991; Mengel Citation1994).
SMC and SOM, TN, AN, TP, AP, TK and AK contents in the forest soils were significantly higher than those in the barren land (). The physicochemical properties of the soils were significantly better in the forests (Zhang et al. Citation2010), which suggests that the introduction of bamboo in coastal areas can improve the fertility of sandy soil. These results could be attributed to the release of carbon (C) and nitrogen (N) into the soil by litter decomposition (the litter of bamboo forests and Cajanus cajan) and mineralization and to the import of organic matter with the fine sand particles carried by the constant wind from the coast.
SOM strongly affects the fertility and the physical, chemical and biological properties of soil. The significant correlations between SOM content and sucrase activity (r = 0.901, P < 0.05) and between protease activity (r = 0.846, P < 0.05) and bacterial count (r = 0.854, P < 0.05) () support the finding that SOM can play an important role in determining soil fertility (Tiessen et al. Citation1994).
D. oldhami plots had the highest SOM and TP contents, and P. amabilis plots had the highest TN, AN and TK contents. TP, AN and TK contents differed significantly among the various species, but SOM and TN contents did not (). This result may have been influenced by the morphology of the root systems of the different species and by litter and root decomposition (). Our results showed that litter and root biomass levels were highly correlated with SOM and TN levels (). Higher levels of SOM in the soil could enhance soil enzymatic activity and increase microbial biomass (Crecchio et al. Citation2004; Acosta-Martínez and Harmel Citation2006).
Soil enzymatic activities
Soil enzymes play a crucial role in the cycling of C, N, phosphorus (P) and sulfur (S), and they may be indicators of soil health. Soil enzymes are also important components of the biochemical processes of soil and contribute to the decomposition of organic matter. They derive mainly from microorganisms but also from plants and animals (Guan Citation1986). Soil enzymatic activity has been used as a sensitive indicator of microbial activity, because the enzymes can respond quickly to disturbances and to changes in the conditions of the soil environment and the structure of microbial communities (Dick et al. Citation1996). In our study, sucrase, protease, urease and catalase activities were higher in the forests than in the barren land (). These results may be due to the effects of fertilization on enzymatic activity. Some studies have reported that soil enzymatic activity increased significantly after fertilization (Chang et al. Citation2007; Islam et al. Citation2011). Fertilizers can affect C use and metabolism by soil microbes and may stimulate microbial growth, resulting in the secretion of enzymes. Moreover, fertilizer uptake by bamboo plants increases litter and root residues. Decomposed litter and root exudates will likely increase the amount of organic matter in the soil () and enhance microbial populations (), favoring the formation of complexes with free enzymes and, therefore, the increase of soil enzymatic activities. The activities of soil enzymes are generally highly associated with SOM content (Beyer et al. Citation1995). Enzymatic activity in our study was positively correlated with SOM content, consistent with reports of increased soil enzymatic activities with increased levels of organic residue (Marinari et al. Citation2000; Graham and Haynes Citation2005).
Sucrase and catalase activities varied among the soils of the bamboo plots, indicating that soil enzymatic activity were influenced by bamboo, possibly due to root distribution and rate of litter decomposition (Zhang et al. Citation2010). Protease and urease activities, however, were not significantly different among A. edulis, D. vario-striata and D. oldhami (). A significant linear correlation was previously found between urease and microbial biomass in agricultural soil (Bhattacharyya et al. Citation2005; Chang et al. Citation2007, Citation2008), indicating that microbial biomass and roots have some ability to secrete protease and urease. Moreover, soil enzymatic activities were higher in the D. oldhami and P. amabilis plots, suggesting that enzymatic activity may have been affected by a high rate of litter decomposition, more root exudates and high activities of microorganisms, possibly due to the higher root biomasses in the plots of both D. oldhami and P. amabilis ().
Soil microbial community
Forest type affects soil microorganisms and their activities via root exudates and litter (Niemia et al. Citation2007). High soil fertility in forests is conducive to microbial growth (Cao et al. Citation2010). Some studies have shown that the activity of microorganisms depends mainly on N availability (Su et al. Citation2004). In our study, the properties of the bacteria were highly correlated with TN and AN contents ().
Our findings highlighted the larger microorganism populations of the bamboo forest soils relative to those of the barren land, which suggests that bamboo can adapt to hostile environments. In addition, our results support a previous study showing that microbial activities in soils with plant cover were higher than those of open areas (Prieto et al. Citation2011). Another study also found that microbial community structure varied with vegetation type (Chang et al. Citation2011). The bacterial count in the D. oldhami plots in our study was higher than in the other plots, which did not differ significantly in bacterial count (). Fungal and actinomycetes counts, however, did vary significantly. The high above- and belowground biomasses in the D. oldhami plots likely provided more substrates for bacterial growth. In this study, litter and root biomasses were positively correlated with bacterial count (). Bacterial count and SOM content were positively correlated, consistent with higher bacterial counts at higher SOM contents and substrate availabilities (Zelles et al. Citation1992; Bossio et al. Citation1998). The total amount of microorganisms was higher in the bamboo forests than in the barren land, which suggests that a higher abundance of microbes is an important factor determining the structure of microbial communities in forest soils (Chang et al. Citation2011).
CONCLUSIONS
The population levels of soil microorganisms were generally different among the different plantation types. The total amounts of microorganisms in the D. oldhami and P. amabilis plots were higher than in the plots of the other species in this coastal ecosystem. The high bacterial counts were also highly correlated with high chemical contents. The soil enzymatic activities were highest for the D. oldhami and P. amabilis forests and were highly associated with SOM, TN, AN, TP and TK contents but less associated with SMC. Litter and root biomasses were also higher for the D. oldhami and P. amabilis forests, which may have been responsible for the changes in soil physico- and biochemical properties. The introduction of bamboo may thus be able to improve soil fertility and microbial activity, while increasing tree diversity, in coastal sand-dune ecosystems where C. equisetifolia forests are dominant.
ACKNOWLEDGMENTS
This study was financially supported by the National Natural Science Foundation of China (No.41171028) and also supported by CFERN (Chinese Forestry Ecosystem Research Network) & GENE (Beijing Ecotek Technology Co., Ltd.) Award Funds on Ecological Paper.
REFERENCES
- Acosta-Martínez V, Harmel RD 2006: Soil microbial communities and enzyme activity under various poultry litter application rates. J. Environ. Qual., 35, 1309–1318. doi:10.2134/jeq2005.0470
- Beyer L, Blume HP, Elsner DC, Willnow A 1995: Soil organic matter composition and microbial activity in urban soils. Sci. Total Environ., 168, 267–278. doi:10.1016/0048-969795:04704-5
- Bhattacharyya P, Chakrabarti K, Chakraborty A 2005: Microbial biomass and enzyme activity in submerged rice soil amended with municipal solid waste compost and decomposed cow manure. Chemosphere, 60, 310–318. doi:10.1016/j.chemosphere.2004.11.097
- Bolan NS, Hedley MJ, White RE 1991: Processes of soil acidification during nitrogen cycling with emphasis on legume pastures. Plant Soil, 134, 53–63. doi:10.1007/BF00010717
- Bossio DA, Scow KM, Gunapala N, Graham KJ 1998: Determinants of soil microbial communities: Effects of agricultural management, season, and soil type on phospholipid fatty acid profiles. Microbial Ecol., 36, 1–12. doi:10.1007/s002489900087
- Cao Y, Fu S, Zou X, Cao H, Shao Y, Zhou L 2010: Soil microbial community composition under Eucalyptus plantations of different age in subtropical China. Eur. J. Soil Biol., 46, 128–135. doi:10.1016/j.ejsobi.2009.12.006
- Cao ZH, Zhou GM, Wong MH 2011: Special issue on bamboo and climate change in China. Bot. Rev., 77, 188–189. doi:10.1007/s12229-011-9064-1
- Chang EH, Chen CT, Chen TH, Chiu CY 2011: Soil microbial communities and activity in sand dunes of subtropical coastal forests. Appl. Soil Ecol., 49, 256–262. doi:10.1016/j.apsoil.2011.04.015
- Chang EH, Chung RS, Tsai YH 2007: Effect of different application rates of organic fertilizer on soil enzyme activity and microbial population. Soil Sci. Plant Nutr., 53, 132–140. doi:10.1111/j.1747-0765.2007.00122.x
- Chang EH, Chung RS, Wang FN 2008: Effect of different types of organic fertilizers on the chemical properties and enzymatic activity of an Oxisol under intensive cultivation of vegetables for 4 years. Soil Sci. Plant Nutr., 54, 587–599. doi:10.1111/j.1747-0765.2008.00264.x
- Chen TH, Chiu CY, Tian G 2005: Seasonal dynamics of soil microbial biomass in coastal sand dune forest. Pedobiologia, 49, 645–653. doi:10.1016/j.pedobi.2005.06.005
- Crecchio C, Curci M, Pizzigallo MDR, Ricciuti P, Ruggiero P 2004: Effects of municipal solid waste compost amendments on soil enzyme activity and bacterial genetic diversity. Soil Biol. Biochem., 36, 1595–1605. doi:10.1016/j.soilbio.2004.07.016
- Dick RP, Breakwell DP, Turco RF 1996: Soil enzyme activity and biodiversity measurements as integrative microbiological indicators. In Methods of Soil Analysis. Special Publication 49, Eds. Doran JW, Jones AJ, pp. 247–272. Soil Science Society of America, Madison, WI.
- Graham MH, Haynes RJ 2005: Organic matter accumulation and fertilizer-induced acidification interact to affect soil microbial and enzyme activity on a long-term sugarcane management experiment. Biol. Fert. Soils., 41, 249–256. doi:10.1007/s00374-005-0830-2
- Guan SY 1986: Soil Enzyme and its Research Method. China Agriculture Press, Beijing. (In Chinese).
- Ishikawa SI, Furukawa A, Oikawa T 1995: Zonal plant distribution and edaphic and micro-meteorological conditions on a coastal sand dune. Ecol. Res., 10, 259–266. doi:10.1007/BF02347851
- Islam MR, Chauhan PS, Kim Y, Kim M, Sa T 2011: Community level functional diversity and enzyme activities in paddy soils under different long-term fertilizer management practices. Biol. Fert. Soils., 47, 599–604. doi:10.1007/s00374-010-0524-2
- Li ZH, Kobayashi M 2004: Plantation future of bamboo in China. J. For. Res., 15, 233–242. doi:10.1007/BF02911032
- Marinari S, Masciandaro G, Ceccanti B, Grego S 2000: Influence of organic and mineral fertilizers on soil biological and physical properties. Bioresour. Technol., 72, 9–17. doi:10.1016/S0960-852499:00094-2
- Mengel K 1994: Symbiotic dinitrogen fixation - its dependence on plant nutrition and its ecophysiological impact. Z. Pflanz Bodenkunde., 157, 233–241. doi:10.1002/jpln.19941570311
- Mengel K, Steffens D 1982: Relationship between the cation/anion uptake and the release of protons by roots of red clover. Z. Pflanz Bodenkunde., 145, 229–236.
- Niemia RM, Vepsäläinena M, Erkomaab K, Ilvesniemic H 2007: Microbial activity during summer in humus layers under Pinus silvestrisand Alnus incana. For. Ecol. Manag., 242, 314–323. doi:10.1016/j.foreco.2007.01.049
- Prieto LH, Bertiller MB, Carrera AL, Olivera NL 2011: Soil enzyme and microbial activity in a grazing ecosystem of Patagonian Monte, Argentina. Geoderma, 162, 281–287. doi:10.1016/j.geoderma.2011.02.011
- Riley D, Barber SA 1971: Effect of ammonium and nitrate fertilization on phosphorus uptake as related to root-induced pH changes at the root-soil interface. Soil Sci. Soc. Am. J., 35, 301–306. doi:10.2136/sssaj1971.03615995003500020035x
- Silveira M 2005: A floresta aberta com bambu no sudoeste da Amazônia: padrões e processos em múltiplas escalas. pp. 1–153. EDUFAC, Rio Branco.
- Su Y, Zhao H, Li Y, Cui J 2004: Carbon mineralization potential in soils of different habitats in the semiarid Horqin sandy land: a laboratory experiment. Arid Land Res. Manag., 18, 39–50. doi:10.1080/15324980490244997
- Tiessen H, Cuevas E, Chacon P 1994: The role of soil organic matter in sustaining soil fertility. Nature, 371, 783–785. doi:10.1038/371783a0
- Williams CH 1980: Soil acidification under clover pasture. Aust. J. Exp. Agric. Anim. Husbandry, 20, 561–567. doi:10.1071/EA9800561.
- Xu JS 2005: A study on techniques of regeneration of protection forest of Casuarina equisetifolia on the coast of Fujian. Protect. For. Sci. Technol., 4, 5–8. doi:10.3969/j.issn.1005-5215.2005.04.002. (In Chinese).
- Xu Q, Jiang P, Xu Z 2008: Soil microbial functional diversity under intensively managed bamboo plantations in southern China. J. Soil Sediment, 8, 177–183. doi:10.1007/s11368-008-0007-3
- Yan F, Schubert S, Mengel K 1996: Soil pH changes during legume growth and application of plant material. Biol. Fert. Soils, 23, 236–242. doi:10.1007/BF00335950
- Zelles L, Bai QY, Beck T, Beese F 1992: Signature fatty acids in phospholipids and lipopolysaccharides as indicators of microbial biomass and community structure in agricultural soils. Soil Biol. Biochem., 24, 317–323. doi:10.1016/0038-071792:90191-Y
- Zhang CS, Xie GD, Fan SH, Zhen L 2010: Variation in vegetation structure and soil properties, and the relation between understory plants and environmental variables under different Phyllostachys pubescens forests in Southeastern China. Environ. Manage., 45, 779–792. doi:10.1007/s00267-010-9429-y
- Zhang M, Zheng YS, Chen LG 2007: A preliminary report on introduction experiment of bamboo into coastal sandy area. J. Southwest For. Coll., 27, 48–50, 62. doi:10.3969/j.issn.2095-1914.2007.01.012 (In Chinese).
- Zheng YS, Rong JD, Chen LG, Zhang LL, Xia HT, Zhang ZX 2008: Dynamics of litter decomposition and nutrient return of Dendrocalamus barbatus forest in the coastal sandy site. J. Fujian Agric. For. Univ. (Nat. Sci. Edn.), 37, 487–490. http://d.wanfangdata.com.cn/Periodical_ fjnydxxb200805010.aspx (In Chinese).
- Zhou BZ, Fu MY, Xie JZ, Yang XS, Li ZC 2005: Ecological functions of bamboo forest: research and application. J. For. Res., 16, 143–147. doi:10.1007/BF02857909
- Zhou GM, Meng CF, Jiang PK, Xu QF 2011: Review of carbon fixation in bamboo forests in china. Bot. Rev., 77, 262–270. doi:10.1007/s12229-011-9082-z