Abstract
According to the slash-and-burn technique used in Eastern Province, Zambia, cut trees are piled and burned in only a part of the cleared fields, because adequate tree biomass is not available to burn the entire field. Due to a recent decrease in emergent trees, not only emergent tree piles but also bush tree piles may exist. Therefore, our objective was to evaluate the changes in soil organic matter followed by nutrient release occurring immediately after burning in spots unburned and burned with emergent and bush trees. Fire intensity was significantly higher where emergent tree piles were present. Total carbon (C) decreased by 25.1% and 14.7% in spots burned with emergent and brush tree piles, respectively, while total nitrogen (N) decreased by 15.0% only at spots burned with emergent tree piles and did not change significantly elsewhere. Additionally, the mortality of microbes with soil heating caused an increase in C mineralization after fire. The levels of available nutrients, such as ammonium nitrogen (NH4-N), available phosphorus (P), and exchangeable potassium (K) and calcium (Ca), increased following the decomposition of soil organic matter and microbial mortality that occurred with an increase in fire intensity. Net N mineralization did not occur, especially in spots burned with emergent tree piles, because the N content of labile organic matter decreased. Maize (Zea mays L.) grain yield increased with fire intensity, because fire increased nutrient availability and limited weed biomass. Although the burned emergent and bush tree piles occupied only 6.9 and 7.5% of total cleared field, respectively, the grain yield in spots burned with emergent and bush trees accounted for 21% and 15% of the total yield, respectively. Therefore, the burning of bush trees, which is increasing because of the decreased number of emergent trees, could result in a decrease in grain yield but could also alleviate the overall severe loss of soil organic matter.
INTRODUCTION
Soil organic matter (SOM) is an essential resource for an available form of nutrients for plants (Cambardella and Elliot Citation1992). In the semiarid tropics of southern Africa, recent rapid conversion of woodland to cropland may cause a decrease in SOM (Jaiyeoba Citation2003; Walker and Desanker Citation2004; Okore et al. Citation2007; Mapanda et al. Citation2013). However, different slash-and-burn techniques used to clear fields, based on the aboveground biomass present in an area, result in varied responses. In tropical humid regions such as Southeast Asia, South America and Central Africa, with aboveground biomass of about 150–350 Mg ha−1 (Hertel et al. Citation2009), slashed trees cover an entire cleared area and the entire area can be easily burned (Rasul and Thapa Citation2003). In contrast, the aboveground biomass in the semiarid tropics of southern Africa covered by miombo woodland composed of emergent trees and bush trees was only 69 Mg ha−1 (Chidumayo Citation1997). In Northern Province, Zambia, trees are collected from a cleared field and branches are also collected from surrounding woodland to compensate for the low levels of biomass; then slash piles are created to cover the entire cleared field and the area is burned (Strømgaard Citation1984). In Eastern Province, trees are cut only within the field being opened, and the cut trees are collected and piled only in part of the field because of the low levels of available biomass. Therefore, only the spots with the tree piles can be burned; the remaining cleared spots without piles are not burned. Traditionally, most of the piles were composed of the emergent trees because more emergent trees were present in the past. Recently, because most emergent tree biomass has disappeared as a result of the short fallow periods currently used, the trees piles composed of bush trees are increasing. Therefore, two kinds of tree piles, such as emergent and bush trees piles, could be present simultaneously within the cleared field. This recent practice may increase the spatial variability of soil fertility and crop production because of the different extents of burning in these mosaic spots of unburned and burned with two levels of biomass.
Burning consumes and alters SOM and is followed by the release of available nutrients. The extent of SOM degradation and nutrient release depends on fire intensity, which can be determined by the maximum temperature and the duration of burning (Hatten and Zabowski Citation2009). Fire intensity increases as the amount of biomass burned per unit area increases. In laboratory experiments, the amount of SOM remaining after a fire decreased with an increase in fire intensity (Dunn et al. Citation1979). However, no coherent results related to the changes of SOM have been observed in field studies of slash-and-burn. This occurs because almost all the burned sites had different soil textures and soil moisture content that affected the change in SOM during burning (Strømgaard Citation1992; Kauffman et al. Citation1993, Citation1995; Giardina et al. Citation2000b; Kendawang et al. Citation2004, Citation2005; Tanaka et al. Citation2004). For instance, Giardina et al. (Citation2000b) reported that the amount of SOM decreased with an increase in fire intensity in a sandy loam soil with low soil moisture content; Strømgaard (Citation1992) showed that levels of SOM increased with any fire intensity in sandy soil with low moisture content. The different changes in SOM quality caused by different soil heating affected the release of labile carbon (C) and available nutrients (Strømgaard Citation1992; Ellingson et al. Citation2000; Giardina et al. Citation2000b; Tanaka et al. Citation2001, Citation2004). Thus, in situ evaluation of changes in SOM after burning is needed in Eastern Province, Zambia.
Although determining in situ fire intensity by measuring both maximum temperature and temporal change of soil temperature during burning provides valuable information, most past studies that determined fire intensity have been based on only maximum temperature (Strømgaard Citation1992; Kauffman et al. Citation1993; Giardina et al. Citation2000a; Tanaka et al. Citation2001, Citation2004; Kendawang et al. Citation2004, Citation2005). Moreover, few studies in Eastern Province have analyzed in situ changes of SOM and labile C as well as available nutrients considering variations in fire intensity inside cleared fields (Chidumayo and Kwibisa Citation2003). Therefore, our objectives were to evaluate in situ changes of SOM, labile C and available nutrients immediately after burning in the entire cleared field including unburned spots, and burned spots with emergent and bush tree piles, and to reveal the relationship between degradation of SOM and fire intensity determined by maximum soil temperature and duration of burning.
MATERIALS AND METHODS
Description of the study site
The study site is located in a village in Eastern Province, Zambia (14°08'S, 31°43'E; 890 m above sea level). The climate has a unimodal distribution of annual rainfall with a rainy season from November to April, and a dry season in the remaining months. The mean annual air temperature was 24°C from 2008 to 2010 and annual rainfall in the rainy season was 762 mm in 2008/2009, 986 mm in 2009/2010 and 1019 mm in 2010/2011. The woodland vegetation type is classified as eastern dry miombo (Chidumayo Citation1997), dominated by Brachystegia manga Dwild., Julbernardia globiflora Benth. and Diplorhynchus condylocarpon (Mull. Arg.) Pichon. The soil was classified as Typic Plinthustalfs (Soil Survey Staff Citation2006). The soil at the depth of 0–5 cm in long fallow had a pH of 6.8 with a water to soil ratio of 5, a soil texture of sandy loam containing 74.8% sand, 14.1% silt and 11.1% clay, total C of 1.40% and total nitrogen (N) of 0.082% (Shinjo et al. Citation2010).
Experimental design
The woodland experimental site (100 m × 230 m) was composed of both emergent trees and bush trees. The flat area of the site was relatively uniform in terms of total C and total N (Shinjo et al. Citation2008). The woodland biomass was 38.3 Mg ha−1, which is much lower than that reported by Chidumayo (Citation1997); this suggests that emergent trees have been lost under serious land use pressure within the region. A part of the area had been opened by slash-and-burn techniques during 2008, 2009 and 2010. Experimental fields (36 m × 31 m) were established with three replications in each year. After trees were cut within each field being opened, bush trees and emergent trees were piled separately in different parts of the experimental field. Trees with a diameter at breast height (DBH) smaller than 27 cm are referred to as “small trees” or brush trees, and trees with a DBH larger than 27 cm as “large trees” or emergent trees, hereinafter. The remaining spots without piles were plowed prior to burning to prevent them from burning. Then, we divided the field into three treatment areas: (1) Unburned: consisting of unburned spots after clearing (950 m2); (2) Bur S: spots burned with piles of small trees (89 m2); (3) Bur L: spots burned with piles of large trees (77 m2).
The burned spots were relatively small and accounted for only 6.9% (Bur L) and 7.5% (Bur S) of the total area of fields (). The piles were left to dry during the dry season and burned at the end of the dry season. The burned spots were not plowed during the first year, following traditional local farming practices. Three seeds of maize (Zea mays L.) were planted in holes using a 1 m × 1 m grid. Weeds were eradicated about 3 and 6 weeks after planting.
Table 1 Characteristics of burned trees and burned spots in 2008–2010
Soil and ash sampling
In October 2008, soils from Unburned were sampled at a depth of 0–15 cm with a 0.3-L soil core because the soil at this depth was mixed uniformly by plowing. Samples collected at nine locations in each treatment were thoroughly mixed to make a composite sample. In October 2008 and 2009, ash samples were collected with an 18.5 cm × 18.5 cm quadrate for both Bur S and Bur L. The ash was defined here as all the residual materials on the ground after burning the tree piles (Giardina et al. Citation2000b). Then, soil samples were collected separately from depths of 0–5, 5–10 and 10–15 cm with a 0.1-L soil core because those areas had not been plowed. Ash and soil samples were collected at each depth from nine and three locations in each treatment for Bur L and Bur S, respectively, and were mixed to make a composite sample. The composite samples were sieved through a 2-mm mesh after removing visible plant debris, then immediately transported to the laboratory and refrigerated at 4°C until testing.
Determination of fire intensity
Fire intensity was determined using maximum soil temperature and duration of the soil heating. The maximum soil temperature was determined by thermo-crayons (Thermo-Crayon M, Nichiyu Giken Kogyo Co., Ltd., Japan) at the Bur L and Bur S in 2008. The crayons were whittled and put between stainless steel nuts. Crayons with melting points of 50, 100, 200, 300, 460, 615, 765 and 885°C were buried at depths of 0, 5, 10 and 15 cm. Temporal change in soil temperature during burning was measured at depths of 1, 3, 5, 10 and 15 cm at the Bur L and Bur S by digital thermometers in 2010 (CT-2310, Custom Corp., Japan). The temperature was recorded every 10 or 15 minutes during the first 7 h after ignition and every 0.5–2 h until the temperature equaled annual average soil temperature. All parts of the thermometer and cables were buried to protect them from fire. Both thermo-crayons and digital thermometer measured the soil temperatures with three replications. The temperature during burning could be considered equal in 2008, 2009 and 2010 because soil water content at the end of the dry season was always less than 2% for all three years and the amount of burned biomass per burned unit area did not differ significantly.
Physicochemical analysis of soil and ash
Soil bulk density was determined by oven-drying soil for 24 h. The dry weight of ash was measured after oven-drying for 24 h, and after grinding soil prior to analysis. Soil pH (H2O) was measured with a soil to water ratio of 1:5. The total C and total N contents of the soil and ash were analyzed by the dry combustion method with a NC analyzer (Vario Max CHN; Elementar, Germany). Available phosphorus (P) was extracted by the Bray-1 method (Bray and Kurtz Citation1945) and was measured with the molybdenum blue method. Exchangeable calcium (Ca), magnesium (Mg), potassium (K) and sodium (Na) were extracted with 1 mol L−1 ammonium acetate at pH 7. Total P, Mg, Ca, K and Na in ash were determined by wet digestion with nitric and sulfuric acid. The Mg and Ca content in the extracts and digested solutions were determined by atomic adsorption spectrometry, the K and Na contents by flame photometry (A-A-640, Shimadzu, Japan) and the P contents by the molybdenum blue method.
Carbon and nitrogen mineralization
C mineralization was determined by the aerobic incubation method (Kendawang et al. Citation2004). The samples were incubated at 30°C for 56 d. The carbon dioxide (CO2) collected by the alkali trap was sampled after 3, 7, 14, 28, 42 and 56 d of incubation and measured by an automatic titrimetric analyzer (COM-1600, Hiranuma Sangyo Co., Ltd., Japan).
N mineralization was determined by the aerobic incubation method (Funakawa et al. Citation2006). After incubation at 30°C for 3, 7, 14, 28, 42 and 56 d, the samples were extracted with 2 mol L−1 potassium chloride (KCl). The ammonium nitrogen (NH4-N) in the extract was determined by the modified indophenol blue method (Rhine et al. Citation1998) and the nitrate nitrogen (NO3-N) in the extract was determined by the Griess-Ilosvay method (Mulvaney Citation1996) after reduction to nitrite nitrogen (NO2-N) with cadmium (Cd).
The daily rate of C and N mineralization for 3 d and 56 d was calculated by Eq. 1:
Plant sampling and analysis
The dry weight was estimated from an allometric equation reported by Chidumayo (Citation1997). Maize stover and grain were collected at three hills for each treatment in April 2009 and 2010. At weeding and harvesting times, aboveground parts of woody and herbaceous weeds were collected separately from three 1 m × 1 m quadrates for each treatment. The samples were weighed after drying at 70°C for 48 h.
Statistical analyses
All statistical analyses were performed with Sigma plot 11.0 (SYSTAT Software Inc., San Jose, CA, USA). All data were expressed on a dry-weight basis (mean ± standard error). One-way analysis of variance (ANOVA) was used to detect significant differences between variables. When ANOVA indicated a significant difference, mean comparisons were performed with Tukey-Kramer multiple comparison test. Soils from the Unburned had been plowed causing them to be uniform at a depth of 0–15 cm. Thus, the mineralization rate in Unburned soil at a depth of 0–15 cm was compared with those in Bur L and Bur S at each depth (0–5, 5–10 and 10–15 cm). Comparing the mass data between Unburned and Burned treatments, we summed the values for 0–5 cm, 5–10 cm and 10–15 cm in each treatment of Bur L and Bur S after converting the unit of the values from concentration (mg kg−1) to mass (Mg ha−1 or kg ha−1).
RESULTS
Biomass of burned trees
shows characteristics of the burned trees and burned spots through the experiment. The burned area covered 6.9% and 7.5% of the total cleared area in Bur L and Bur S, respectively, and burned biomass per unit burned area of Bur L and Bur S was 209.4 ± 20.1 Mg ha−1 and 84.5 ± 13.4 Mg ha−1, respectively. As indicated by the small values of standard error, the burned biomass per unit area was not different significantly among the three experimental years.
Fire intensity
shows the maximum soil temperature during burning. The maximum temperature in Bur L rose at the depth of 0–10 cm, whereas in Bur S it increased only at the depth of 0–5 cm. In Bur L, the temperature rose to 300–460°C and 100–200°C, at depths of 5 and 10 cm, respectively. In Bur S, the temperature rose to only 50–100°C and 50°C at depths of 5 and 10 cm, respectively. In the 2008 experiment, it took 2–3 d in Bur L and 2–3 h in Bur S to completely burn the trees. This observation was followed by the temporal change in soil temperature in 2010 (). The average of maximum temperature was 442, 294, 159, 108 and 62°C at depths of 1, 3, 5, 10 and 15 cm in Bur L (a), and was 220, 136, 110, 82 and 62°C at depths of 1, 3, 5, 10 and 15 cm in Bur S (), respectively. Soil temperature above 80°C at 3 cm deep lasted for 56 h in Bur L (), but for only 9 h in Bur S (). Thus, burning was more intense in Bur L than that in Bur S in terms of maximum temperature, and the depth and duration of soil heating.
Table 2 Maximum soil temperature during burning measured by Thermo-crayon in 2008
Soil and ash physicochemical properties
The soil water content before burning was 1.77 ± 0.03% in all the treatments. shows the soil and ash physiochemical properties after applying the treatments. Total C at 0–15 cm deep decreased in Bur L and Bur S by 25.1 and 14.7%, respectively, compared with the Unburned. A significant difference in total C between Bur L and Bur S was found only at the depth of 0–5 cm. Total N at 0–15 cm deep decreased only in Bur L by 15.0%, and seemed to decrease in Bur S by 9% but not significantly. Compared with the value of soil pH in the Unburned, those in Bur L and Bur S rose, although no significant difference was found between Bur L and Bur S. Available P at the depth of 0–15 cm increased with an increase in fire intensity and was higher in Bur L than that in Bur S at depths of 0–5 and 5–10 cm. Exchangeable Ca and K at the depth of 0–15 cm increased only in Bur L compared with Unburned. The C contents of ash were 10%, and the total C, total P and bases in ash were significantly higher in Bur L than those in Bur S because the mass of ash was 37.07 ± 1.13 Mg ha−1 in Bur L and 12.26 ± 0.84 Mg ha−1 in Bur S.
Table 3 Physicochemical properties of soil and ash in burned and unburned spots
Carbon mineralization
shows the cumulative C mineralization at a depth of 0–15 cm. The amount of C mineralization for 56 d of incubation was 951 ± 46, 939 ± 30, 1277 ± 36 kg C ha−1, in the Unburned, Bur S and Bur L, respectively. C mineralization in Bur L was significantly larger than those in Unburned and Bur S through incubation (P < 0.05). Also, C mineralization in Bur S was larger than those in Unburned only until 28 d of incubation (P < 0.05). Those differences in C mineralization among the treatments () were mostly attributable to the difference of the daily rate of C mineralization for the first 3 d (). The rate for the first 3 d significantly increased in Bur L at all the depths compared with Unburned, but only at a depth of 0–5 cm in Bur S ().
Table 4 Daily rate of carbon (C) mineralization in burned and unburned spots
Figure 2 Cumulative carbon (C) mineralization at a depth of 0–15 cm during the incubation experiment. Samples from unburned and spots burned with small trees (Bur S) were collected in October 2008 and samples from spots burned with piles of large trees (Bur L) were collected in October 2009. Bars indicate standard error (n = 3).
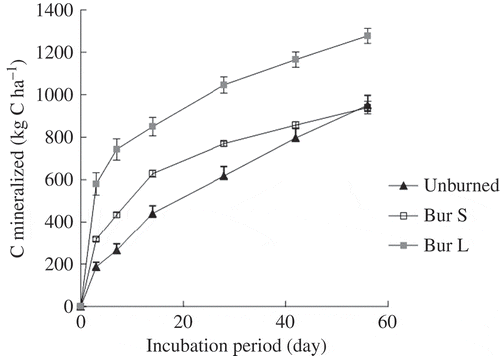
Nitrogen mineralization
shows N mineralization at a depth of 0–15 cm; burning increased the amount of inorganic N during the incubation (P < 0.05). Inorganic N was higher in Bur L than that in Bur S until 42 d of incubation (P < 0.05). Net N mineralization during the incubation, in Unburned, Bur S and Bur L showed no significant difference at 34.1 ± 3.7, 30.6 ± 2.7 and 14.1 ± 7.1 kg N ha−1, respectively.
Figure 3 Net nitrogen (N) mineralization at a depth of 0–15 cm during the incubation experiment. Samples from unburned and spots burned with small trees (Bur S) were collected in October 2008 and samples from spots burned with piles of large trees (Bur L) were collected in October 2009. Bars indicate standard error (n = 3).
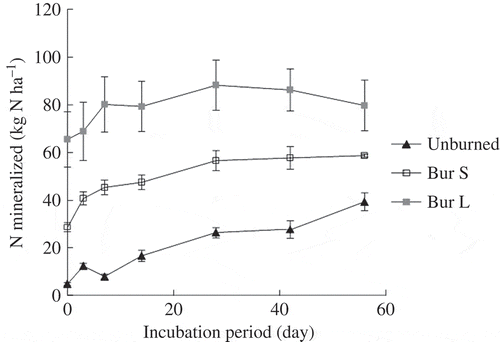
presents inorganic–N at 0 d incubation (In–N0) and the daily rate of change in the amount of inorganic N. While In–N0 increased by soil heating to the same extent between Bur L and Bur S at the depth of 0–5 cm, In–N0 was higher in Bur L than that in Bur S at the depth of 5–10 and 10–15 cm. In–N0 was present only as NH4-N. Positive and negative values of the rate indicated net N mineralization and immobilization, respectively. The rate of N mineralization during the incubation was negative only in Bur L at the depth of 0–5 cm, although it was not significantly different among the treatments. At 0–3 d of incubation, N was immobilized significantly only in Bur L at a depth of 0–5 cm.
Table 5 Inorganic nitrogen (N) at 0 day incubation and daily rate of N mineralization and immobilization in burned and unburned spots
indicates the amount of NH4-N and NO3-N at a depth of 0–15 cm. NO3-N was the predominant form of mineralization in Unburned, while NH4–N was predominant in Bur S and Bur L at the beginning of mineralization. NO3-N increased in Bur S gradually after 14 d of incubation, while NH4-N was present. NO3-N was not present in Bur L until after 7 d of incubation. After 14 d of incubation, standard errors of NO3-N and NH4-N in Bur L became very large because one of the three replicated samples in Bur L started nitrification after 14 d of incubation, while the other two samples in Bur L started after 28 d.
Figure 4 The amounts of (a) ammonium nitrogen (NH4-N) and (b) nitrate nitrogen (NO3-N) at a depth of 0–15 cm during the incubation experiment. Samples from unburned and spots burned with small trees (Bur S) were collected in October 2008 and samples from spots burned with piles of large trees (Bur L) were collected in October 2009. Bars indicate standard error (n = 3).
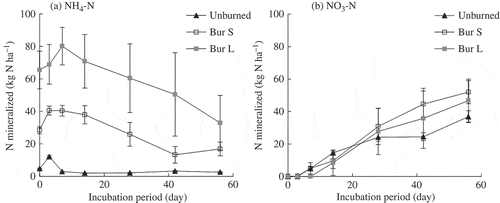
Maize production and weed growth
shows the aboveground biomass of maize and weeds. The increased precipitation in 2009/2010 (986 mm) compared with 2008/2009 (762 mm) resulted in significantly higher production of maize grain and stover, especially for stover in the Unburned. Grain yield and mass of stover increased by burning in both years and grain yield was higher in Bur L than that in Bur S, while the area of Bur L and Bur S was not different, 6.9 and 7.5% of the total cleared field, respectively (). Based on these results, the grain yield in Bur L and Bur S accounted for 21.5 and 15.7% of total yield, respectively. No woody weeds were present in Bur L in both years. The amount of woody weed biomass in Bur S was not significantly different from that in Unburned. The biomass of herbaceous weeds decreased by burning significantly in both years, and was not significantly different between Bur L and Bur S.
Table 6 Aboveground biomass of weed and maize in burned and unburned spots
DISCUSSION
Fire intensity increased with an increase in burned biomass per unit of burned area ( and , ). Soil was heated more deeply and for longer periods at higher temperatures in Bur L than in Bur S (, ). Kendawang et al. (2004; Citation2005) found the maximum soil temperature also increased with an increase in burned biomass in Southeast Asia. The maximum temperature with the same burned biomass in the above study in Southeast Asia, however, was lower than in our study, 100–150°C burning with 200 Mg ha−1 and 40–50°C burning with 100 Mg ha−1 at a depth of 5 cm. High soil moisture content in Southeast Asia (around 20%) may cause the low maximum soil temperature observed there (Giardina et al. Citation2000a; Tanaka et al. Citation2001).
The increase in fire intensity led to a further decrease in total C and N at a depth of 0–5 cm (). Johnson et al. (Citation2011) also showed a decrease in total C by 26 and 50% as well as a decrease in total N by 20 and 38% with low and high fire intensity, respectively, in a semiarid in America. Some of the loss of C caused by soil heating will be compensated in the second cropping year because ash will be incorporated with soil by plowing ().
C mineralization during the first 3 d of incubation and NH4-N (In–N0) at a depth of 0–15 cm increased with an increase in fire intensity (, ). Those increases might be derived from the mortality of microbes; microbe mortality started when temperatures reached 80–120°C (DeBano et al. Citation1998). Therefore, C mineralization and NH4-N (In–N0) was higher in Bur L than in Bur S because microbes were killed in deeper soil in Bur L than that in Bur S because of the deeper heating in the Bur L sites (, ).
An increase in C mineralization caused by soil heating during the early stage of incubation was also observed in Southeast Asia (Kendawang et al. Citation2004). Despite the documented increase in C mineralization with an increase in fire intensity, C mineralization at the end of incubation was higher only in Bur L compared with that in the Unburned (). Presumably, soil heating in Bur S might produce not only mineralizable organic material through volatilization of SOM or mortality of microbial biomass but also recalcitrant forms of C such as char or black C by imperfect combustion of SOM (González-Pérez et al. Citation2004).
An increased release of NH4-N caused by soil heating based on an increase in fire intensity was also found in semiarid America (Johnson et al. Citation2011) and Southeast Asia (Tanaka et al. Citation2001; Citation2004). Although the amount of inorganic N was highest in Bur L during the incubation at a depth of 0–15 cm, net N mineralization was apparently the lowest in Bur L among the three treatments (). Although the rate of C mineralization and In–N0 were the same between samples at a depth of 0–5 cm and 5–10 cm in Bur L ( and ), N was significantly immobilized at a depth of 0–5 cm in Bur L during the first 3 d of incubation (). This might be related to the decrease in the N content of labile organic matter at a depth of 0–5 cm, which was suggested by the significant decrease in total N only at a depth of 0–5 cm.
Recovery of nitrification activity at the depth of 0–15 cm was delayed with an increase in fire intensity () which reflected nitrifier mortality starting at 90°C (Dunn et al. Citation1979; Nearya et al. Citation1999). The time needed for nitrification activity to recover in Bur L varied among the samples (). Ellingson et al. (Citation2000) reported that nitrification activity was promoted by high-intensity burning, while Dunn et al. (Citation1979) showed the activity decreased with high-intensity burning. The increase in soil pH (to 8.1) by burning may not influence nitrifying bacteria because the pH was already neutral before burning (). Ste-Marie and Paré (Citation1999) found that the pH at which nitrifying bacteria were inhibited was less than 5 or more than 10.
The amount of available P increased with fire intensity (). Heat-induced microbial mortality may have been the primary factor leading to the increase in available P (Giardina et al. Citation2000a). In Bur L, available P and exchangeable Ca and K increased because degradation of SOM was promoted by the high maximum temperature and long duration of burning (Giardina et al. Citation2000a). The exchangeable bases and available P also seemed to increase depending on fire intensity in Southeast Asia (Tanaka et al. Citation2004). Total P and bases in ash will gradually be incorporated into the soil through the percolation of rain and by plowing during the second cropping year.
Herbaceous weeds had a low resistance to soil heating (); weed seeds were killed at about 90°C resulting in low herbaceous biomass (Martin et al. Citation1975). In addition, biomass of woody weeds decreased with strong fire intensity () because their large roots could survive only low-intensity fires.
In Unburned areas, accounting for 85% of the total field, maize yield was 0.7 Mg ha−1 because of the low available nutrient ( and ). In Bur S and Bur L, covering 7.5% and 6.9% of the total field, maize yield was 2.3 Mg ha−1 and 3.2 Mg ha−1, respectively (). Those increases in maize yield are due to the increase in available nutrient by the degradation of SOM and leachate from ash, and the decrease in weeds. Those extents were more pronounced in Bur L than in Bur S. However, the content of C and N in SOM decreased more in Bur L than in Bur S (). Thus, the recent slash-and-burn techniques, with the presence of both emergent and bush trees, increased the spatial variability in SOM, available nutrients and weeds, which affected maize grain.
CONCLUSION
In Eastern Province, Zambia, the effects of soil heating on changes of SOM followed by nutrient release depended on fire intensity. The amounts of burned tree biomass, 80 Mg ha−1 (bush trees), and 200 Mg ha−1 (emergent trees), had different impacts on the soil nutrients, which were controlled by the maximum temperature and duration of soil heating. As fire intensity increased, increases in C mineralization, NH4-N, exchangeable Ca and K, and available P were observed through the increased degradation of SOM and mortality of microbes. Net N mineralization did not increase through burning because the N content of labile organic matter decreased by burning. Even though the burned area with spots of bush tree and emergent tree piles covered only 7.5 and 6.9% of total cleared field, grain yield increased to 15 and 21% of the total grain yield for the entire field, respectively. However, a more pronounced decrease in total C and N was observed in spots burned with emergent trees than those with bush trees. Therefore, because more areas are being burned with bush trees owing to the decrease in emergent trees, grain yield may decrease, although the severe decrease in SOM may be alleviated. This study clarifies the effects of the changes in slash-and-burn techniques on SOM and crop production. Further study is needed to elucidate the long-term effects on SOM and crop production in this area.
ACKNOWLEDGMENTS
Our work was financially supported by a Grant-in-Aid for Scientific Research (B) from the Japan Society for the Promotion of Science (No. 23310027), and the projects known as the Vulnerability and Resilience of Social-Ecological Systems, and Desertification and Livelihoods in Semi-Arid Afro-Eurasia, of the Research Institute for Humanity and Nature.
REFERENCES
- Bray RH, Kurtz LT 1945: Determination of total, organic and available forms of phosphorus in soils. Soil Sci., 59, 39–45.
- Cambardella CA, Elliot ET 1992: Particulate soil organic-matter changes across a grassland cultivation sequence. Soil Sci. Soc. Am. J., 56, 777–783.
- Chidumayo EN 1997: Miombo Ecology and Management an Introduction, Intermediate Technology Publications, London.
- Chidumayo EN, Kwibisa L 2003: Effects of deforestation on grass biomass and soil nutrient status in miombo woodland, Zambia. Agric. Ecosyst. Environ., 96, 97–105.
- DeBano LF, Neary DG, Ffolliott PF 1998: Fire’s Effects on Ecosystems, John Wiley and Sons, Inc, New York. 71–80.
- Dunn PH, DeBano LF, Eberlein GE 1979: Effects of burning on Chaparral soils: II. soil microbes and nitrogen mineralization. Soil Sci. Soc. Am. J., 43, 509–514.
- Ellingson LJ, Kauffman JB, Cummings DL, Sanford Jr RL, Jaramillo VJ 2000: Soil N dynamics associated with deforestation, biomass burning, and pasture conversion in a Mexican tropical dry forest. For. Ecol. Manage., 137, 41–51.
- Funakawa S, Hayashi Y, Tazaki I, Sawada K, Kosaki T 2006: The main functions of the fallow phase in shifting cultivation by the Karen people in northern Thailand: a quantitative analysis of soil organic matter dynamics. Tropics, 15, 1–27.
- Giardina CP, Sanford Jr RL, Døckersmith I 2000b: Changes in soil phosphorus and nitrogen during slash burning of a dry tropical forest. Soil Sci. Soc. Am. J., 64, 399–405.
- Giardina CP, Sanford RL, Døckersmith IC, Jaramillo VJ 2000a: The effects of slash burning on ecosystem nutrients during the land preparation phase of shifting cultivation. Plant Soil, 220, 247–260.
- González-Pérez JA, González-Vila FJ, Almendros G, Knicker H 2004: The effect of fire on soil organic matter—a review. Environ. Int., 30, 855–870.
- Hatten JA, Zabowski D 2009: Changes in soil organic matter pools and carbon mineralization as influenced by fire intensity. Soil Sci. Soc. Am. J., 73, 262–273.
- Hertel D, Moser G, Culmsee H, Erasmi S, Horna V, Schuldt B, Ch L 2009: Below- and above-ground biomass and net primary production in a paleotropical natural forest (Sulawesi, Indonesia) as compared to neotropical forests. For. Ecol. Manage., 258, 1904–1912.
- Jaiyeoba IA 2003: Changes in soil properties due to continuous cultivation in Nigerian semiarid Savannah. Soil Till. Res., 70, 91–98.
- Johnson BG, Johnson DW, Miller WW, Carroll-Moore EM, Board DI 2011: The effects of slash pile burning on soil and water macronutrients. Soil Sci., 176, 413–425.
- Kauffman JB, Cummings DL, Ward DE, Babbitt R 1995: Fire in the Brazilian Amazon: 1. Biomass, nutrient pools, and losses in slashed primary forests. Oecologia, 104, 397–408.
- Kauffman JB, Sanford RL, Cummings DL, Salcedo IH, Sampaio EVSB 1993: Biomass and nutrient dynamics associated with slash fires in neotropical dry forest. Ecology, 74, 140–151.
- Kendawang JJ, Tanaka S, Ishihara J, Shibata K, Sabang J, Ninomiya I, Ishizuka S, Sakurai K 2004: Effects of shifting cultivation on soil ecosystems in Sarawak, Malaysia. I. Slash and burning at Balai Ringin and Sabal experimental sites and effect on soil organic matter. Soil Sci. Plant Nutr., 50, 677–687.
- Kendawang JJ, Tanaka S, Shibata K, Yoshida N, Sabang J, Ninomiya I, Sakurai K 2005: Effects of shifting cultivation on soil ecosystems in Sarawak, Malaysia. III. Results of burning practice and changes in soil organic matter at Niah and Bakam experimental sites. Soil Sci. Plant Nutr., 51, 515–523.
- Mapanda F, Munotengwa S, Wuta M, Nyamugafata P, Nyamangara J 2013: Short-term responses of selected soil properties to clearing and cropping of miombo woodlands in central Zimbabwe. Soil Till. Res., 129, 75–82.
- Martin RE, Miller RL, Cushwa CT 1975: Germination response of legume seeds subjected to moist and dry heat. Ecology, 56, 1441–1445.
- Mulvaney RL 1996: Nitrogen-Inorganic forms. In Methods of Soil Analysis, Ed. Sparks DL, pp. 1123–1184. Soil Science Society of America, Madison, WI.
- Nearya DG, Klopatekb CC, DeBanoc LF, Ffolliott PF 1999: Fire effects on belowground sustainability: a review and synthesis. Forest Ecol. Manage., 122, 51–71.
- Okore IK, Tijani-Eniola H, Agboola AA, Aiyelari EA 2007: Impact of land clearing methods and cropping systems on labile soil C and N pools in the humid zone Forest of Nigeria. Agric. Ecosyst. Environ., 120, 250–258.
- Rasul G, Thapa GB 2003: Shifting cultivation in the mountains of South and Southeast Asia: regional patterns and factors influencing the change. Land Degrad. Develop., 14, 495–508.
- Rhine ED, Sims GK, Mulvaney RL, Pratt EJ 1998: Improving the Berthelot reaction for determining ammonium in soil extracts and water. Soil Sci. Soc. Am., 62, 473–480.
- Shinjo H, Ando K, Noro Y, Kuramitsu H, Takenaka S, Miyazaki H, Miura R, Tanaka U, Shibata S, Sokotela S 2010: Impact of land clearing on crop production and soil fertility in a miombo woodland in Eastern Province, Zambia. The report of Vulnerability and Resilience of Social-Ecological Systems (FY2010 FR4 Proposal), pp. 16–20, Research Institute for Human and Nature, Japan.
- Shinjo H, Tanaka U, Shibata S, Miura R, Miyazaki H, Noro Y, Mwale M 2008: Ecological resilience and human activities under variable environment. The report of Vulnerability and Resilience of Social-Ecological Systems (FY2008 FR4 Proposal), pp. 9–14, Research Institute for Human and Nature, Japan.
- Soil Survey Staff 2006: Keys to Soil Taxonomy 10th edn, United States Department of Agriculture Natural Resources Conservation Service, Washington, DC.
- Ste-Marie C, Paré D 1999: Soil, pH and N availability effects on net nitrification in the forest floors of a range of boreal forest stands. Soil Biol. Biochem., 31, 1579–1589.
- Strømgaard P 1984: The immediate effect of burning and ash fertilization. Plant Soil., 80, 307–320.
- Strømgaard P 1992: Immediate and long-term effects of fire and ash fertilization on a Zambian miombo woodland soil. Agric. Ecosyst. Environ., 41, 19–37.
- Tanaka S, Ando T, Funakawa S, Sukhrun C, Kaewkhongkha T, Sakurai K 2001: Effect of burning on soil organic matter content and N mineralization under shifting cultivation system of Karen people in northern Thailand. Soil Sci. Plant Nutr., 47, 547–558.
- Tanaka S, Kendawang JJ, Ishihara J, Shibata K, Kou A, Jee A, Ninomiya I, Sakurai K 2004: Effects of shifting cultivation on soil ecosystems in Sarawak, Malaysia. II. Changes in soil chemical properties and runoff water at Balai Ringin and Sabal experimental sites. Soil Sci. Plant Nutr., 50, 689–699.
- Walker SM, Desanker PV 2004: The impact of land use on soil carbon in Miombo Woodlands of Malawi. Forest Ecol. Manage., 203, 345–360.