Abstract
The aim of this study was to assess the mitigating effects of lime nitrogen (calcium cyanamide) and dicyandiamide (DCD) application on nitrous oxide (N2O) emissions from fields of green tea [Camellia sinensis (L.) Kuntze]. The study was conducted in experimental tea fields in which the fertilizer application rate was 544 kg nitrogen (N) ha−1 yr−1 for 2 years. The mean cumulative N2O flux from the soil between the canopies of tea plants for 2 years was 7.1 ± 0.9 kg N ha−1 yr−1 in control plots. The cumulative N2O flux in the plots supplemented with lime nitrogen was 3.5 ± 0.1 kgN ha−1, approximately 51% lower than that in control plots. This reduction was due to the inhibition of nitrification by DCD, which was produced from the lime nitrogen. In addition, the increase in soil pH by lime in the lime nitrogen may also be another reason for the decreased N2O emissions from soil in LN plots. Meanwhile, the cumulative N2O flux in DCD plots was not significantly different from that in control plots. The seasonal variability in N2O emissions in DCD plots differed from that in control plots and application of DCD sometimes increased N2O emissions from tea field soil. The nitrification inhibition effect of lime nitrogen and DCD helped to delay nitrification of ammonium-nitrogen (NH4+-N), leading to high NH4+-N concentrations and a high ratio of NH4+-N /nitrate-nitrogen (NO3–-N) in the soil. The inhibitors delayed the formation of NO3–-N in soil. N uptake by tea plants was almost the same among all three treatments.
1. INTRODUCTION
The concentration of nitrous oxide (N2O) in the atmosphere has increased over recent decades because of human activities. The global warming potential of N2O is approximately 300 times that of carbon dioxide. N2O is involved in the destruction of the ozone layer (Crutzen Citation1970). The majority of anthropogenic N2O is emitted by agricultural activities (Bouwman et al. Citation1995; Mosier et al. Citation1996), among which agricultural soil is a major source of N2O (Davidson and Kingerlee Citation1997; Mosier et al. Citation1998). N2O is produced during nitrification (Bremner et al. Citation1980; Wrage et al. Citation2001) and denitrification (Firestone et al. Citation1980) in the soil.
Tea [Camellia sinensis (L.) Kuntze] plants are cultivated in Japan for production of green tea. More nitrogen (N) fertilizer is used to cultivate tea plants than to cultivate other crops. For example, the recommended amount of N fertilizer for green tea fields in Shizuoka, Japan, is 540 kg N ha−1 yr−1. The main reason for the high rate of fertilizer application is that it can increase the concentrations of free amino acids in tea leaves, one of the most important quality indexes of Japanese green tea. However, heavy application of N fertilizer does not always increase the yield and quality of tea products, but instead can cause problems such as an increased level of nitrate-nitrogen (NO3–-N) in the surrounding water systems (Nagai Citation1991; Hirono et al. Citation2009), acidification of soil and emission of high levels of N2O, one of the major greenhouse gasses (Tokuda and Hayatsu Citation2004). Among these problems, increased N2O emission is of particular interest. Akiyama et al. (Citation2006) reviewed previous studies and reported that the estimated mean N2O emission and estimated annual fertilizer-induced emission factors for tea fields in Japan were 24.3 ± 16.3 kg N ha−1 and 2.82 ± 1.80%, respectively. These emission factors were much higher than those of other upland fields (0.62 ± 0.48%) and paddy fields (0.31 ± 0.31%). Because of the high rate of N application and the high N2O emission factor, reducing N2O emissions from green tea fields would be a significant step towards reducing N2O emissions from agricultural soil.
In recent years, the amount of fertilizer applied to tea fields in Japan has been reduced in an effort to reduce environmental impacts. Some new methods of fertilizer application have been proposed to increase the efficiency of N uptake by tea plants. Among the new methods of fertilizer application, application of lime nitrogen and dicyandiamide (DCD), one of the nitrification inhibitors used in tea cultivations in Japan, are promising methods to increase the N use efficiency of tea plants. Lime nitrogen consists primarily of calcium cyanamide; it contains approximately 20% N and 50% lime in the form of calcium oxide (CaO) (Klasse Citation1996). In the soil, calcium cyanamide is broken down into urea and DCD. DCD inhibits ammonia oxidation by microbes and is also degraded by some microbes. The inhibition of nitrification by DCD allows ammonium-nitrogen (NH4+-N) to remain the soil for longer. This condition is favorable for the growth of tea plants because tea plants prefer NH4+-N to nitrate-nitrogen (NO3–-N) (Ishigaki Citation1978) and because the amount of N leached from the soil is reduced. In addition, N2O emissions are also reduced by directly limiting the nitrification process and by indirectly limiting the denitrification process by suppressing rapid formation of NO3–-N (Aulakh et al. Citation1984; Bhatia et al. Citation2010).
In a previous study, application of lime nitrogen reduced N2O emissions from tea field soil (Tokuda Citation2005; Yamamoto et al. Citation2014). In those studies, the effects of lime nitrogen on N2O emissions were, however, evaluated based on the results of 1 year of monitoring. Interannual variations of N2O emissions have not been assessed. In addition, there is no report on the effect of DCD application on N2O emissions from tea field soil. Thus, further detailed information was required to clarify the effects of lime nitrogen and DCD on N2O emission from tea fields. Therefore, in this study, we assessed the mitigating effects of lime nitrogen and DCD on N2O emissions from tea fields based on detailed results obtained in a 2-year monitoring experiment.
2. MATERIALS AND METHODS
2.1. Site description
The experimental tea field was located at the Tea Experimental Station (34°48'N, 138°08'E), National Agriculture and Food Research Organization (NARO) Institute of Vegetable and Tea Science (NIVTS), Shizuoka, Japan. Shizuoka is classified as a Humid subtropical climate (Cfa) by the Köppen climate classification system. The soil at the experimental site is classified as Yellow soil (clay loam). The width of the canopy was 1.5 m in this field. The distance between the canopies of tea plants was 0.3 m.
2.2. Experimental setup
Experimental plots were established with three replicates of three treatments. Each plot was 1.8 m wide and 9 m long. The treatments applied to the plots included conventional fertilizer (control), lime nitrogen (LN), and dicyandiamide (DCD). The timing of fertilizer application and the kinds and amounts of fertilizers applied to the plots are shown in . Since tea is a perennial crop and requires N for growing throughout the year, multiple N fertilizer applications were needed. As lime nitrogen and DCD contain N, the amounts of urea and ammonium sulfate were proportionately decreased in these treatments to ensure that N levels were the same among the treatments. Application rates of lime nitrogen and DCD were 26% and 6% of the annual N application rate, respectively. All fertilizers were applied by hand to the soil surface between the canopies of tea plants. After fertilizer application, the soil between canopies was cultivated to a depth of approximately 0.1 m below the soil surface. Other cultivation management followed normal management practices.
Table 1 Timing of fertilizer application and the kinds and amounts of nitrogen (N) fertilizers applied to each treatment plot
2.3. N2O flux measurements
N2O fluxes from the soil surface were measured using a closed-chamber method from September 4, 2010 to September 7, 2012. Cylindrical chamber sets were used for these measurements. The chamber set consisted of a stainless-steel base, 0.3 m in diameter and 0.06 m high, and a vinyl-chloride chamber, 0.3 m in diameter and 0.14 m high. At each of nine plots, the chamber base was inserted into the soil between the canopies of tea plants and under the canopy of tea plants to a depth of 0.05 m. Urethane foam was applied around the join between the vinyl-chloride chamber and the base to ensure airtightness. The bases between the canopies of tea plants were removed during harvesting and fertilizer application and were replaced immediately after harvesting and fertilizer application. N2O fluxes were measured at 1- to 7-day intervals. Almost all of the gas sampling was conducted between 10:00 AM and 11:30 AM. To acquire samples, chambers were placed on the bases and tightly clamped using clips. Gas samples (20 mL) were withdrawn from each chamber two or three times at approximately 12-min intervals into evacuated 10-mL glass vials using plastic syringes. The vials were taken to the laboratory and stored at room temperature until analysis.
A gas chromatograph (GC-14B, Shimadzu Corp., Kyoto, Japan) equipped with an electron capture detector (ECD) was used to measure N2O concentrations as described in detail by Hirono and Nonaka (Citation2012).
N2O flux was calculated from the linear increase in gas concentration during the time the chamber was closed. Cumulative N2O fluxes were calculated by the trapezoidal integration method.
2.4. Soil sampling and analysis
Soil samples were taken from a depth of 0–0.2 m after removing visible branches and leaves on the soil surface between the canopies of tea plants at nine plots. The samples were sieved through a 2-mm mesh and stored at approximately 4°C until analysis. The samples were extracted with 2 mol L−1 potassium chloride (KCl) [wet soil to KCl solution = 1:10 weight/volume (w/v)] to determine the concentrations of NO3–-N and NH4+-N, and were extracted with pure water (wet soil to water = 1:5 w/v) to determine the dicyandiamide concentration. The concentrations of NO3–-N and NH4+-N were determined colorimetrically according to the methods of Hayashi et al. (Citation1997) and Rhine et al. (Citation1998), respectively. A DU-7500 spectrophotometer (Beckman, Fullerton, CA) was used to measure absorbance. The DCD concentrations were determined by high-performance liquid chromatography (LC-10AD, Shimadzu, Japan) according to Yamamoto et al. (Citation2012). DCD concentrations were first measured on August 26, 2011. Soil pH was measured by the 1:2.5 soil-water extraction method using a glass electrode.
2.5. Meteorological data acquisition
The soil temperature was measured at a depth of 0.1 m with a digital thermometer (PC-9215, Sato Keiryoki Mfg. Co., Ltd., Japan). The volumetric water content was measured at a depth of 0.1 m with a soil moisture sensor (CS610, Campbell Scientific, Inc., UT). The water-filled pore space (WFPS) was calculated by dividing the volumetric water content by porosity. Oxygen (O2) concentration was measured at a depth of 0.1 m with a soil O2 sensor (MIJ-03, Environmental Measurement Japan Co., Ltd., Japan). The soil temperature, water-filled pore space (WFPS), and O2 concentration were measured in the soil between the canopies of tea plants in a single plot, and measurements of WFPS and O2 concentrations started in November, 2010. A dataset of daily precipitation was obtained from the meteorological monitoring system in the NIVTS.
2.6. Yield and N content of new shoots of tea plants
New shoots in the first-crop season were harvested at each plot on May 13, 2011 and May 11, 2012, and those in the second-crop season were harvested on June 29, 2011 and June 27, 2012. Yield was determined gravimetrically. Subsamples (50 g) were taken from tea plants in each plot, heated in a microwave oven for 1 min to deactivate enzymes, and then dried at 70°C in a convection oven. The sample was weighed to determine dry weight, and then milled. The total N content in tea leaf samples was determined using a carbon (C) and N analyzer (Flash EA 1112, Thermoquest, Milan, Italy).
2.7. Statistical analysis
Data for cumulative N2O emissions, yield and N content of new shoots were analyzed using Tukey’s highly significant difference (HSD) test, with the statistical package R (R Foundation for Statistical Computing).
3. RESULTS
3.1. N2O emissions
The mean cumulative N2O flux for 2 years was 7.1 ± 0.9 kg N ha−1 yr−1 (7.1 ± 0.6 and 7.1 ± 1.3 kg N ha−1 yr−1 in the first and second year, respectively) in control plots and 3.5 ± 0.1 kg N ha−1 yr−1 (2.5 ± 0.04 and 4.5 ± 0.2 kg N ha−1 yr−1 in the first and second year, respectively) in LN plots (). Therefore, the cumulative N2O flux was significantly decreased (by approximately 51%) in LN plots compared with control plots. The mean cumulative N2O flux for 2 years in DCD plots was 8.3 ± 0.6, and the cumulative N2O flux in DCD plots in the first year was 4.7 ± 0.9 kg N ha−1 yr−1, which was not significantly different from those in control plots (). However, in the second year, the cumulative N2O flux in DCD plots (12.0 ± 0.3 kg N ha−1 yr−1) was significantly greater than that in control plots.
Table 2 Cumulative nitrous oxide (N2O) emissions from three treatment plots
N2O fluxes in control plots showed similar seasonal variations in the first and second years (). N2O fluxes increased after fertilizer application in September, and then declined to trace levels before December. From December to February, low N2O fluxes were observed. N2O fluxes increased again after fertilizer application in late February and peaked in late April. After fertilizer application in May, N2O fluxes increased again, peaked in June or July, and declined to low levels before September. To assess the effects of LN and DCD on N2O emissions considering these seasonal variations, we divided the year into four periods, as follows: Period I, from immediately before fertilizer application in September until the end of November; Period II, from December until immediately before fertilizer application in February; Period III, from fertilizer application in February until immediately before fertilizer application in May; Period IV, from fertilizer application in May until immediately before fertilizer application in September (, ).
Figure 1 Time series data for N2O flux from soil between canopy of tea plants and daily rainfall. Means of three replicates +standard error of means. LN, plot supplemented with lime nitrogen; DCD, plot supplemented with dicyandiamide. Dotted line shows timing of nitrogen fertilizer application. Gray background shows periods I and III.
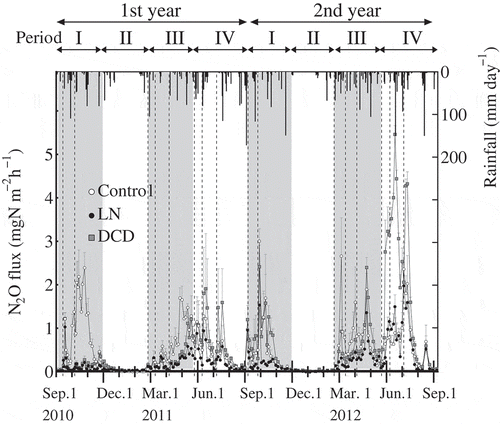
In Period I of both years, N2O flux sharply increased in control plots after rainfall following the first fertilizer application (). Then, the N2O flux in control plots increased after a heavy rainfall event, increased again after the second fertilizer application in Period I, peaked and then gradually declined to almost trace levels. N2O emissions in the LN and DCD plots were significantly lower than those in control plots in Period I of the first year; however, there were no significant differences in N2O emissions among the three treatments in Period I of the second year ().
In Period II of both years, there were low N2O fluxes from all treatments, and only small differences in N2O fluxes among the three treatments.
In Period III of both years, N2O fluxes gradually increased after fertilizer application in late February and showed temporal variations after rainfall events in control plots. N2O fluxes in DCD plots were lower than those in control plots until early May in both years. After that, the N2O fluxes in DCD plots increased to levels similar to (the first year), or even higher (the second year) than, those in control plots. The N2O fluxes in LN plots were lower than those in control plots early May, but the differences in N2O fluxes between LN and control plots became smaller from late May in the both years.
In Period IV, N2O fluxes in control plots peaked in June or July, then gradually decreased to trace levels by the end of this period. N2O fluxes in LN plots were similar to, or sometimes higher than, those in control plots. N2O fluxes in DCD plots tended to be higher than those in control plots.
In this study, the effects of application of lime nitrogen and DCD on N2O emissions from tea field soils were assessed, and N2O flux from soil under the canopy of tea plants, where no fertilizer was applied, was not subject to evaluation. In fact, there were no significant differences in N2O flux from soil under the canopy of tea plants among the fertilizer treatments (data not shown). Thus, the effect of the application of lime nitrogen and DCD on N2O flux only from soil between canopies was discussed later.
3.2. Rainfall, soil temperature, WFPS and O2 concentration
The cumulative rainfall in the first and second year was 2626 and 2510 mm, respectively. The 10-cm soil temperature ranged from 5.8°C on March 11, 2011 to 24.7°C on August 17, 2012 (a). Because of the monsoon climate, there were some periods of heavy rainfall. For example, there were heavy rainfall events in Period I (from September to October), Period III (late February to March) and the early part of Period IV (from June to July) during the study period. In contrast, there was less rain in Period II (from January to February) and the late part of Period IV (August). WFPS showed temporal fluctuations and ranged from 52 to 97% (b). It increased following rainfall events and gradually decreased thereafter. The O2 concentration in the soil changed seasonally; it tended to be high in Period II, low in Period IV and showed sharp decreases following heavy rainfall events (b).
3.3. Soil properties
In Period I, the concentrations of NH4+-N in control plots rapidly increased after fertilizer application in September and decreased to trace levels before winter in both years (a). The concentration of NH4+-N in DCD and LN plots increased after fertilizer application on September 13 and remained at an elevated level until December in the first year. In Period II, the concentration of NH4+-N in DCD and LN plots was higher than that in control plots in the first year. In Period III, after fertilizer application in late February, the concentrations of NH4+-N in all plots increased, peaking in April and June. The peak concentrations of NH4+-N differed among the three treatments after fertilizer applications; the NH4+-N concentrations in LN and DCD plots were higher than that in control plots. In Period IV of both years, the concentrations of NH4+-N declined, and were almost at trace levels by late August.
Figure 3 Time series data for (a) ammonium-nitrogen (NH4+-N) concentration in soil, (b) nitrate-nitrogen (NO3--N) concentration in soil, (c) ratio of NH4+-N to NO3–-N concentration in soil, (d) soil pH (H2O), and (e) dicyandiamide (DCD) concentration in soil. Means of three replicates +standard error of means. LN, plot supplemented with lime nitrogen; DCD, plot supplemented with DCD. Dotted line shows timing of nitrogen fertilizer application. (-) means dimensionless.
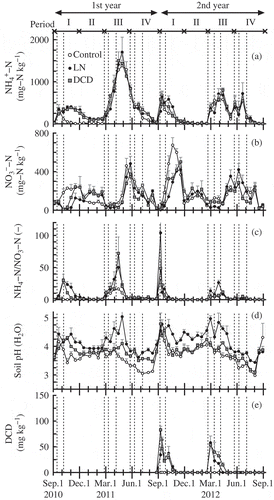
The timing of the increase in NO3–- N concentrations coincided with that of the decrease in NH4+-N concentrations (a–b). The peak in the NO3–-N concentration after fertilizer application in control plots in Periods I and III of both years was earlier than that in LN and DCD plots after applications of lime nitrogen and DCD, respectively.
The ratio of NH4+-N to NO3–-N is shown in c. This ratio is an index of the effectiveness of nitrification inhibition, according to Guthrie and Bomke (Citation1980). High values of the NH4+-N to NO3–-N ratio observed in Periods I and III of the first year indicated that lime nitrogen and DCD effectively inhibited nitrification. The effect of lime nitrogen on nitrification inhibition was still high in the second year, whereas the effect of nitrification inhibition in DCD plots in the second year became lower than that in the first year.
Among the three treatments, the soil pH values were lowest in the control plots almost throughout the study period, ranging from 3.0 to 4.2 (d). There was a large decrease in soil pH in the control plots in Periods I and III of both years. The decrease in soil pH in the DCD plots in Periods I and III of the first year was slower than that in the control plots, while differences between soil pH in the DCD plots and that in the control plots were slight in the second year. The soil pH in the LN plots was highest almost throughout the study period, and the highest pH value recorded in LN plots was 5.0. The decreases in soil pH in the LN plots in Periods I and III of both years were delayed compared with those in the control plots.
DCD concentrations in LN and DCD plots are shown in e. DCD concentrations increased just after application of lime nitrogen and DCD, and decreased to undetectable levels within approximately 2 months. DCD remained at detectable levels in soil for about 2 weeks longer in DCD plots than in LN plots.
3.4. Yield and N content of new shoots
There were no significant differences in the yield and N content of new shoots harvested in the first- and second-crop seasons in 2011 and 2012 among the fertilizer treatments (data not shown).
4. DISCUSSION
We conducted a 2-year field experiment to assess the effects of lime nitrogen and DCD on N2O emissions from soil in green tea fields. Application of lime nitrogen significantly reduced N2O emissions from soil in tea fields (). The average reduction in N2O emissions from tea field soil was approximately 51% over 2 years, consistent with the results reported by Tokuda (Citation2005) and Yamamoto et al. (Citation2014). This reduction rate was greater than those reported for arable land soils (Yamamoto et al. Citation2012). Meanwhile, the cumulative N2O flux in DCD plots was not significantly different from those in control plots (). However, looking at several-week periods after application of lime nitrogen and DCD in Periods I and III of the first year and Period III of the second year, N2O emissions from both LN and DCD plots were lower than those from control plots. The period during which reduced N2O emissions were recorded in LN and DCD plots was consistent with the period in which DCD concentrations were higher in the soil (e). During the period in which DCD could be detected, the decreases in NH4+-N concentrations in LN and DCD plots were delayed compared with those in the control plots after fertilizer application in Period I of the second year (a). In addition, the earliest increase in NO3–-N concentration was in control plots, followed by DCD and LN plots (b). The nitrification inhibition effect of DCD led to a high ratio of NH4+-N/NO3-N in soil in LN and DCD plots (c), similar to the results reported by Guthrie and Bomke (Citation1980). In the changes in soil pH in the present study, the delay before soil pH decreased after fertilizer application in autumn coincided with the period in which soil NH4+-N concentrations decreased. This result showed that the production of protons (hydrogen ions, H+) via nitrification was more limited in LN and DCD plots than in control plots, providing further evidence that nitrification was inhibited in the LN and DCD plots. Therefore, the reduced N2O emissions in these periods were attributed to inhibition of nitrification by DCD. Inhibition of nitrification reduced N2O emissions via nitrification and presumably lowered NO3–-N concentration, resulting in a reduction in N2O emissions via denitrification (Bhatia et al. Citation2010).
The mitigating effect of lime nitrogen and DCD on N2O emissions differed depending on the season when these fertilizers were applied (). After the application of lime nitrogen and DCD in early Period III (February and March) of both years, there were small differences in the inorganic N concentrations and N2O fluxes among the three plots ( and a–b). In contrast, there were large differences in these parameters among the three plots after fertilizer application in Period I (September and October) of both years, although the high DCD concentrations in LN and DCD plots were detected in Period I and Period III (e). The differences between N transformation in Period I and Period III of both years were probably caused by the differences in soil temperature. The soil temperature in Period III was lower than that in Period I (a), resulting in lower activities of microorganisms, including nitrifiers. The DCD would have disappeared from the soil via leaching before microbes became more active with rising soil temperature. Thus, DCD and LN applications have less of a nitrification inhibition effect in colder seasons, when microorganisms have lower activities.
The period during which N2O flux was lower than that in control plots was longer in LN plots than in DCD plots (). During this period, the DCD concentrations in LN plots were lower than those in DCD plots (e). Thus, nitrification inhibition alone could not explain the decreased N2O emissions from LN plots. Tokuda and Hayatsu (Citation2001) reported a negative exponential relationship between soil pH and N2O emission potential. In the present study, the soil pH values in LN plots were higher than those in other plots most of the time; this may be a reason for the decreased N2O emissions from soil in LN plots. Besides the effects of DCD and soil pH, the effects of calcium cyanamide and cyanamide on soil microorganisms could be another reason for the decreased N2O emissions from soil in LN plots. Application of lime nitrogen affects soil microorganisms (Haenseler and Moyer Citation1937; Klasse Citation1996). As reported by Yamamoto et al. (Citation2014), cyanamide may contribute to the decrease in N2O emissions from tea field soil.
In LN and DCD plots, the N2O emissions sometimes reached the same level as or a higher level than those from control plots in the late Period III of the second year and Period IV of the first and second year. By 2 months after application of lime nitrogen and DCD, DCD was not present at detectable concentrations in the soil in LN and DCD plots (e). The 2-month period before DCD became undetectable was consistent with reports that the partial inhibition of nitrification by DCD lasts for 4–10 weeks (Zaman and Blennerhassett Citation2010) and that the reduction in NO3–-N concentration in the soil lasts for 60–80 d after DCD application (Cookson and Cornforth Citation2002; Di and Cameron Citation2012). This was because of the decreased concentration of DCD by degradation and leaching, that is, a weaker nitrification inhibition effect. In the soil, DCD is degraded via enzymatic mineralization by microbes (Hauser and Haselwandter Citation1990; Schwarzer and Haselwandter Citation1991) and by interactions with metal oxides (Amberger Citation1986). The degradation rate of DCD in soils increases with increasing temperature (Bronson et al. Citation1989; Schwarzer and Haselwandter Citation1991; Rajbanshi et al. Citation1992; Di and Cameron Citation2004; Kelliher et al. Citation2008). Degradation of DCD caused a decrease in the nitrification inhibition effect (c) in Period IV of both years. Hence, NO3–-N produced by nitrification was likely accumulated in shallow soil layers. In addition, there was higher rainfall in late Period III and Period IV than in other periods (1237 mm and 926 mm rainfall in Period IV of the first and second year, respectively). Rainfall can leach DCD from the soil (Vogeler et al. Citation2007) and increase WFPS. Some studies reported that denitrification can become a major source of N2O when NO3 –-N concentrations in soil increase after nitrification with higher WFPS after heavy rainfall (Kumar et al. Citation2000; Luo et al. Citation2008; Saggar et al. Citation2008). The O2 concentrations were lower in Period IV than in other periods (b). Our data indicated that the conditions in Period IV of both years were suitable for denitrification, resulting in higher N2O emissions observed even in LN and DCD plots as well as control plots.
In our study, application of DCD increased the cumulative N2O emissions compared to that from control plots in the second year (), even though other studies have reported that DCD effectively reduced N2O emissions from agricultural soils (Akiyama et al. Citation2009). In particular, N2O emissions from DCD plots tended to increase in Period IV and were much higher in the second year than those in the first year (). One of the possible causes of increase in N2O emissions in DCD plots was the effect of repeated application of DCD. The repeated application of DCD to tea field soil was reported to affect the composition of microorganisms, resulting in a decreased effect of nitrification inhibition of DCD year by year (Miura et al. Citation2007). Rajbanshi et al. (Citation1992) reported that repeated application of DCD enhanced its degradation in soil. Our results also showed that effectiveness of nitrification inhibition of DCD in the second year was lower than that in the first year (c), suggesting that repeated application of DCD affected soil microorganisms involved in N transformation. However, there are conflicting reports on the effects of repeated applications of DCD on soil microorganisms. In some studies, repeated application of DCD had little effect on the DCD degradation rate and enzyme activity (Rodgers Citation1986; McTaggart et al. Citation1997). It is difficult to clarify the reason for the increased N2O emissions in DCD plots in the rainy season (Period IV) based on our data. Therefore, further research is required to study in detail the effects of repeated application of DCD on soil microorganisms and on pathways of N2O production in tea field soils.
Application of lime nitrogen and DCD did not increase yield and N content of new tea shoots in our study. In contrast, many studies conducted with different cultivation systems reported that the use of nitrification inhibitors increased yield and N uptake (Di and Cameron Citation2002; Zaman and Blennerhassett Citation2010). This has also been reported for tea crops. In another study, application of calcium cyanamide allowed for lower levels of N to be applied to tea fields, and reduced the amount of NO3–-N leaching from the soil (Kawai et al. Citation1953). A nitrification inhibitor prevented soil acidification and maintained or increased yield, even when N was applied at lower levels than conventional levels (Katsuki Citation2005; Oh et al. Citation2006). Watabe (Citation1991) reported that when DCD was applied to tea fields, the N content and amino acid content in tea leaves increased as a result. It was also reported that application of DCD can reduce the amount of N leaching from the soil (Morita et al. Citation2002), and that coated urea with DCD improved the quality of tea leaves compared with those cultivated with conventional fertilizer (Miura et al. Citation2007; Sakaida et al. Citation2010).
5. CONCLUSIONS
Our results showed that application of lime nitrogen can effectively reduce N2O emissions from tea field soil. The mechanisms of this reduction were the inhibition of nitrification by DCD, and the increase in soil pH as a result of lime in the lime nitrogen. However, the effectiveness of DCD in reducing N2O emissions from tea fields strongly depends on the timing of DCD application and on climate conditions, including rainfall and temperature. Application of lime nitrogen and DCD did not negatively affect the yield and N content of new fresh leaves of tea plants. Because of nitrification inhibition, NH4+-N remained in the soil for a long time after applications of lime nitrogen and DCD. Since tea plants prefer NH4+-N to NO3–-N, the nitrification inhibition effects of lime nitrogen and DCD make them suitable fertilizers for tea plants.
ACKNOWLEDGMENTS
This research was supported by a grant for “Development of mitigation and adaptation techniques to global warming in the sectors of agriculture, forestry, and fisheries” from the Ministry of Agriculture, Forestry and Fisheries of Japan. The authors thank Megumi Takagi, Eiko Satou, Akihiro Nishizaka and Norishige Kondou for their research assistance, and Shunji Suzuki, Yasuaki Tamura, Hirofumi Iwakiri, Hiromasa Tanaka and Yoshiaki Seki of the Research Support Center, NIVTS for their assistance in experimental field management.
REFERENCES
- Akiyama H, Yan X, Yagi K 2009: Evaluation of effectiveness of enhanced-efficiency fertilizers as mitigation options for N2O and NO emissions from agricultural soils: Meta-analysis. Glob. Chang. Biol., 16, 1837–1846. doi:10.1111/j.1365-2486.2009.02031.x
- Akiyama H, Yan XY, Yagi K 2006: Estimations of emission factors for fertilizer-induced direct N2O emissions from agricultural soils in Japan: Summary of available data. Soil Sci. Plant Nutr., 52, 774–787. doi:10.1111/j.1747-0765.2006.00097.x
- Amberger A 1986: Potentials of nitrification inhibitors in modern N-fertilizer management. Zeitschrift für Pflanzenernährung und Bodenkunde, 149, 469–484. doi:10.1002/jpln.19861490410
- Aulakh MS, Rennie DA, Paul EA 1984: Gaseous nitrogen losses from soils under zero-till as compared with conventional-till management systems. J. Environ. Qual., 13, 130–136. doi:10.2134/jeq1984.00472425001300010024x
- Bhatia A, Sasmal S, Jain N, Pathak H, Kumar R, Singh A 2010: Mitigating nitrous oxide emission from soil under conventional and no-tillage in wheat using nitrification inhibitors. Agric. Ecosyst. Environ., 136, 247–253. doi:10.1016/j.agee.2010.01.004
- Bouwman A, Van der Hoek K, Olivier J 1995: Uncertainties in the global source distribution of nitrous oxide. J. Geophys. Res, 100, 2785–2800. doi:10.1029/94JD02946
- Bremner JM, Blackmer AM, Waring SA 1980: Formation of nitrous oxide and dinitrogen by chemical decomposition of hydroxylamine in soils. Soil Biol. Biochem., 12, 263–269. doi:10.1016/0038-0717(80)90072-3
- Bronson KF, Touchton JT, Hauck RD 1989: Decomposition rate of dicyandiamide and nitrification inhibition. Commun. Soil Sci. Plant Anal., 20, 2067–2078. doi:10.1080/00103628909368201
- Cookson WR, Cornforth IS 2002: Dicyandiamide slows nitrification in dairy cattle urine patches: Effects on soil solution composition, soil pH and pasture yield. Soil Biol. Biochem., 34, 1461–1465. doi:10.1016/S0038-0717(02)00090-1
- Crutzen PJ 1970: The influence of nitrogen oxides on the atmospheric ozone content. Q. J. Royal Meteorol. Soc., 96, 320–325. doi:10.1002/qj.49709640815
- Davidson EA, Kingerlee W 1997: A global inventory of nitric oxide emissions from soils. Nutr. Cycl. Agroecosyst., 48, 37–50. doi:10.1023/A:1009738715891
- Di HJ, Cameron KC 2002: The use of a nitrification inhibitor, dicyandiamide (DCD), to decrease nitrate leaching and nitrous oxide emissions in a simulated grazed and irrigated grassland. Soil Use Manage., 18, 395–403. doi:10.1111/j.1475-2743.2002.tb00258.x
- Di HJ, Cameron KC 2004: Effects of temperature and application rate of a nitrification inhibitor, dicyandiamide (DCD), on nitrification rate and microbial biomass in a grazed pasture soil. Aust. J. Soil Res, 42, 927–932. doi:10.1071/SR04050
- Di HJ, Cameron KC 2012: How does the application of different nitrification inhibitors affect nitrous oxide emissions and nitrate leaching from cow urine in grazed pastures?. Soil Use Manage., 28, 54–61. doi:10.1111/j.1475-2743.2011.00373.x
- Firestone MK, Firestone RB, Tiedje JM 1980: Nitrous oxide from soil denitrification: Factors controlling its biological production. Science, 208, 749–751. doi:10.1126/science.208.4445.749
- Guthrie TF, Bomke AA 1980: Nitrification inhibition by N-serve and ATC in soils of varying texture. Soil Sci. Soc. Am. J., 44, 314–320. doi:10.2136/sssaj1980.03615995004400020023x
- Haenseler CM, Moyer TR 1937: Effect of calcium cyanamide on the soil microflora with special reference to certain plant parasites. Soil Sci., 43, 133–152. doi:10.1097/00010694-193702000-00003
- Hauser M, Haselwandter K 1990: Degradation of dicyandiamide by soil bacteria. Soil Biol. Biochem., 22, 113–114. doi:10.1016/0038-0717(90)90069-C
- Hayashi A, Sakamoto K, Yoshida T 1997: A rapid method for determination of nitrate in soil by hydrazine reduction procedure. Jpn. J. Soil Sci. Plant Nutr., 68, 322–326. (in Japanese).
- Hirono Y, Nonaka K 2012: Nitrous oxide emissions from green tea fields in Japan: Contribution of emissions from soil between rows and soil under the canopy of tea plants. Soil Sci Plant Nutr., 58, 384–392. doi:10.1080/00380768.2012.686434
- Hirono Y, Watanabe I, Nonaka K 2009: Trends in water quality around an intensive tea-growing area in Shizuoka, Japan. Soil Sci. Plant Nutr., 55, 783–792. doi:10.1111/j.1747-0765.2009.00413.x
- Ishigaki K 1978: Studies on the nutritive characteristics of tea plant. Bull. Natl. Res. Inst. Tea, 14, 1–152. (in Japanese with English summary).
- Katsuki T 2005: Low nitrogen input of tea cultivation using lime nitrogen. Chagyo Kenkyu Hokoku (Tea Res. J.), 100, 80–82. (in Japanese).
- Kawai S, Ishigaki K, Okamoto N 1953: On the percolation loss of nitrogen nutrients from the tea garden soil. Chagyo Kenkyu Hokoku (Tea Res. J.), 2, 66–68. in Japanese with English summary). doi:10.5979/cha.1953.2_66
- Kelliher FM, Clough TJ, Clark H, Rys G, Sedcole JR 2008: The temperature dependence of dicyandiamide (DCD) degradation in soils: A data synthesis. Soil Biol. Biochem., 40, 1878–1882. doi:10.1016/j.soilbio.2008.03.013
- Klasse HJ 1996: Calcium cyanamide -An effective tool to control clubroot- A review. Acta Hort., 407, 403–409.
- Kumar U, Jain MC, Pathak H, Kumar S, Majumdar D 2000: Nitrous oxide emission from different fertilizers and its mitigation by nitrification inhibitors in irrigated rice. Biol. Fertil. Soils, 32, 474–478. doi:10.1007/s003740000278
- Luo J, Ledgard SF, de Klein CAM, Lindsey SB, Kear M 2008: Effects of dairy farming intensification on nitrous oxide emissions. Plant Soil, 309, 227–237. doi:10.1007/s11104-007-9444-9
- McTaggart IP, Clayton H, Parker J, Swan L, Smith KA 1997: Nitrous oxide emissions from grassland and spring barley, following N fertiliser application with and without nitrification inhibitors. Biol. Fertil. Soils, 25, 261–268. doi:10.1007/s003740050312
- Miura N, Uchimura K, Kaji T, Nakamura T, Yoshida S, Katsuda M, Karasuyama M 2007: Effects of application using coated urea and dicyandiamide for tea field. Chagyo Kenkyu Hokoku (Tea Res. J.), 103, 41–50. (in Japanese with English summary). doi:10.5979/cha.2007.41
- Morita A, Takano H, Oota M, Yoneyama T 2002: Nitrification and denitrification in an acidic soil of tea (Camellia sinensis L.) field estimated by δ15N values of leached nitrogen from the soil columns treated with ammonium nitrate in the presence or absence of a nitrification inhibitor and with slow-release fertilizers. Soil Sci. Plant Nutr., 48, 585–593. doi:10.1080/00380768.2002.10409242
- Mosier AR, Delgado JA, Keller M 1998: Methane and nitrous oxide fluxes in an acid Oxisol in western Puerto Rico: Effects of tillage, liming and fertilization. Soil Biol. Biochem., 30, 2087–2098. doi:10.1016/S0038-0717(98)00085-6
- Mosier AR, Duxbury JM, Freney JR, Heinemeyer O, Minami K 1996: Nitrous oxide emissions from agricultural fields: Assessment, measurement and mitigation. Plant Soil, 181, 95–108. doi:10.1007/BF00011296
- Nagai S 1991: Hydrochemical approach on groundwater pollution. State and issue of inorganic pollution. J. Groundwater Hydrol., 33, 145–154. (in Japanese).
- Oh K, Kato T, Li Z., Li F. 2006: Environmental problems from tea cultivation in Japan and a control measure using calcium cyanamide. Pedosphere, 16, 770–777. doi:10.1016/S1002-0160(06)60113-6
- Rajbanshi SS, Benckiser G, Ottow JCG 1992: Effects of concentration, incubation temperature, and repeated applications on degradation kinetics of dicyandiamide (DCD) in model experiments with a silt loam soil. Biol. Fertil. Soils, 13, 61–64. doi:10.1007/BF00337336
- Rhine ED, Mulvaney RL, Pratt EJ, Sims GK 1998: Improving the Berthelot reaction for determining ammonium in soil extracts and water. Soil Sci. Soc. Am. J., 62, 473–480. doi:10.2136/sssaj1998.03615995006200020026x
- Rodgers GA 1986: Potency of nitrification inhibitors following their repeated application to soil. Biol Fertil. Soils, 2, 105–108. doi:10.1007/BF00257587
- Saggar S, Tate KR, Giltrap DL, Singh J 2008: Soil-atmosphere exchange of nitrous oxide and methane in New Zealand terrestrial ecosystems and their mitigation options: A review. Plant Soil, 309, 25–42. doi:10.1007/s11104-007-9421-3
- Sakaida T, Yoshioka T, Kubota A, Nitabaru J, Nakazono K 2010: Effects of the application of coated urea and dicyandiamide and reduction in the amount of nitrogen fertilizer on tea garden for gyokuro. Tea Res. J, 110, 9–18. (in Japanese with English summary).
- Schwarzer CH, Haselwandter K 1991: Enzymatic degradation of the nitrification inhibitor dicyandiamide by a soil bacterium. Soil Biol. Biochem., 23, 309–310. doi:10.1016/0038-0717(91)90070-Z
- Tokuda S 2005: Mitigation options for nitrous oxide emission from tea field. Chagyo Kenkyu Hokoku (Tea Res. J.), 100, 45–48. (in Japanese). doi:10.5979/cha.2005.100_45
- Tokuda S, Hayatsu M 2001: Nitrous oxide emission potential of 21 acidic tea field soils in japan. Soil Sci. Plant Nutr., 47, 637–642. doi:10.1080/00380768.2001.10408427
- Tokuda S, Hayatsu M 2004: Nitrous oxide flux from a tea field amended with a large amount of nitrogen fertilizer and soil environmental factors controlling the flux. Soil Sci. Plant Nutr., 50, 365–374. doi:10.1080/00380768.2004.10408490
- Vogeler I, Blard A, Bolan N 2007: Modelling DCD effect on nitrate leaching under controlled conditions. Aust. J. Soil Res, 45, 310–317. doi:10.1071/SR06177
- Watabe N 1991: Influence of nitrification inhibitor and calcium on growth of tea plant and amino acid content of the new shoots. Jpn. J. Soil Sci. Plant Nutr., 62, 493–499. (in Japanese with English summary).
- Wrage N, Velthof GL, van Beusichem ML, Oenema O 2001: Role of nitrifier denitrification in the production of nitrous oxide. Soil Biol. Biochem., 33, 1723–1732. doi:10.1016/S0038-0717(01)00096-7
- Yamamoto A, Akiyama H, Naokawa T, Miyazaki Y, Honda Y, Sano Y, Nakajima Y, Yagi K 2014: Lime-nitrogen application affects nitrification, denitrification, and N2O emission in an acidic tea soil. Biol. Fertil. Soils, 50, 53–62. doi:10.1007/s00374-013-0830-6
- Yamamoto A, Akiyama H, Naokawa T, Yagi K 2012: Effect of lime-nitrogen application on N2O emission from an Andosol vegetable field. Soil Sci. Plant Nutr., 58, 245–254. doi:10.1080/00380768.2012.667766
- Zaman M, Blennerhassett JD 2010: Effects of the different rates of urease and nitrification inhibitors on gaseous emissions of ammonia and nitrous oxide, nitrate leaching and pasture production from urine patches in an intensive grazed pasture system. Agric. Ecosyst. Environ., 136, 236–246. doi:10.1016/j.agee.2009.07.010