Abstract
Boron (B) is an essential micronutrient element for plants, and it exists in many forms in plants. B toxicity is common in arid and semiarid regions, and inappropriate application of B fertilizer can lead to B toxicity. Trifoliate orange [Pincers trifoliata (L.) Raf] is a main citrus rootstock of the world, and is sensitive to excess B. Although it is known that leaf chlorosis is the typical visible symptom of B toxicity, it is still unclear how leaf chlorosis is related to different forms of B in Trifoliate orange seedlings. A hydroponic experiment was carried out with four B levels: B5 (5 μmol L–1), B10 (10 μmol L–1), B20 (20 μmol L–1) and B100 (100 μmol L–1). After a 117-d culture, free B, semi-bound B and bound B in root, stem and leaves were extracted and measured. The results showed that the seedling height, the number of leaves and the dry weight decreased with increasing B supply. Leaf B concentration was generally higher than those of roots and stems. New leaf B concentration showed no difference in different B treatments, but was lower than that of older leaves. B in root and stem was mostly in the form of bound B, and B in leaves was mainly in free B form. Moreover, the free B, semi-bound B and bound B had little changes in roots and stems with various B treatments, but free B in leaves significantly increased as leaf chlorosis was aggravated, indicating that leaf chlorosis is closely related with free B in leaves.
INTRODUCTION
Boron (B) is required for the growth and development of higher plants (Cakmak and Römheld Citation1997). Thellier et al. (Citation1979) used two purified stable isotopes (10B and 11B) and identified four compartments for B in cells: free space (including easily dissociable borate monoesters), cytoplasm, vacuole and the fourth interpreted as corresponding to very stable borate diesters in the cell wall. Hu and Brown (Citation1994) suggested that a substantial amount of B is bound in the cell wall, particularly when B is at a low level. When B is sufficient, up to 60% of the B is in a soluble form (Matoh Citation1997). Evidence shows that the primary function of B is involved in the formation of cell walls through a borate-diol bonding with two rhamnogalacturonan II (RG-II) molecules (Matoh Citation1997; O’Neill et al. Citation2001).
B toxicity is common in arid and semiarid regions, where B level is frequently high in soil or irrigation (Nable et al. Citation1997). The threshold between B deficiency and toxicity is very narrow. Thus, significant B toxicity can occur simply when fertilizers containing B are repeatedly used, or composted refuse and fly ash from the burning of brown coal (lignite) are used to mitigate B deficiency in soil (Nable et al. Citation1997; Günes and Alpaslan Citation2000). Excessive B can reduce plant growth and cause necrosis on leaf margins or tips, depending on the type of leaf venation (Reid and Fitzpatrick Citation2009).
Citrus is one of the most important economic crops in China as well as in the world. Widespread B deficiency in citrus orchard soil in southern China (Wang et al. Citation2011) causes loss of productivity and quality (Jiang et al. Citation2009). However, continuous application of B fertilizer to mitigate B deficiency has resulted in B toxicity in some citrus orchards recently (Ling et al. Citation2010). Trifoliate orange [Pincers trifoliata (L.) Raf] is the main rootstock (Zhou and Xia Citation2005), and it is more sensitive to B toxicity than other rootstocks (Sheng et al. Citation2010; Guidong et al. Citation2011). However, there is limited knowledge about the relationship between leaf chlorosis and different B forms. So, the aim of the present experiment was to study the relationship between leaf chlorosis and different B forms in Trifoliate orange seedlings.
MATERIALS AND METHODS
Plant material and culture
Seeds of Trifoliate orange rootstocks were placed in 50°C water, soaked for 1 h and then rinsed repeatedly with deionized water. The seeds were placed on a glass petri dish with moistened gauze and transferred to an incubator at 28°C. Germinated seeds were transferred into plastic pots filled with deionized water, and seedlings were allowed to grow to 3–4 leaves. The plants were then placed in a greenhouse under fluorescent lamp conditions for continual growth. Some seedlings of uniform size were selected to grow in a 3-L black plastic barrel, each containing three seedlings. After 59 d cultivation, each seedling was transplanted to a 3-L black plastic barrel separately.
Plants were cultured with four boron (B) levels: B5 (5 μmol L–1), B10 (10 μmol L–1), B20 (20 μmol L–1) and B100 (100 μmol L–1). The seedlings were cultivated in 1/4 modified Hoagland and Arnon solution (Citation1950) containing 2 mmol L–1 KNO3, 2.45 mmol L–1 Ca(NO3)2•4H2O, 2 mmol L–1 MgSO4•7H2O, 0.28 mmol L–1 Na2HPO4•12H2O, 0.64 mmol L–1 NaH2PO4•2H2O, 8.9 μmol L–1 MnCl2•4H2O, 1.5 μmol L–1 ZnSO4•7H2O, 0.32 μmol L–1 CuSO4•5H2O, 0.37 μmol L–1 Na2MoO4•2H2O and 57.5 μmol L–1 [CH2N(CH2COO)2]2FeNa·3H2O, Ethylenediamine tetraacetic acid, ferric disodium salt. The pH was adjusted to 5.8–6.2 every day with 0.5 mol L–1 H2SO4 or 1 mol/L NaOH. The culture solution was aerated for 20 min every 4 h, and renewed every 7 d during the whole experiment.
These experimental plants were placed in a greenhouse under fluorescent lamp conditions at Huazhong Agricultural University, Wuhan, China. The experiment started on December 6, 2011, and lasted 117 days.
New leaves in this study were the leaves younger than 1 month, and old leaves were the leaves more than 1 month old.
Determination of boron forms
B forms were extracted by the method described by Du et al. (Citation2002) with some modification. In this experiment, a batch of 0.2 g of fully expanded leaves (including normal and three levels of chlorotic leaves in each treatment for 117 d) and 0.5 g of root and stem was cut into pieces of approximately 1 mm2, and was put into a clean and dry plastic bottle with 10 mL distilled water. The bottle was shaken at 100 rpm at 25°C in a water bath for 24 h. The mixture was then filtered through 0.15-mm gauze and quantitative filter paper. The supernatant was collected to determine B concentration as free B form. The residue was added with 10 mL 1 mol L–1 sodium chloride (NaCl), and shaken at 100 rpm at 25°C in the water bath for 24 h. After filtration, the supernatant was determined for B as semi-bound B form. The residue was then added with 10 mL 1 mol L–1 hydrochloric acid (HCl), and shaken at 100 rpm at 25°C in the water bath for 24 h. The B in the supernatant was bound B.
Sampling, plant analysis and boron determination
When plants were harvested, the plant height was measured from the bottom of the epicotyl along the stem to the shoot tip. The root, stem and leaves were separately sampled. All the samples were washed initially with tap water and then deionized water three times. Samples were dried at 70°C for 3 d. After weights of the fresh and dry samples were recorded, the dry samples were ground to fine powder and stored for subsequent analyses.
The content of B was measured by burning 0.2 g powder sample of root and stem and 0.05 g powder sample of leaves at 550°C in porcelain crucibles. The ash from the burning was added with 10 mL 0.1 mol L–1 HCl and then the solution was filtered using a quantitative filter paper. The filtrate was collected in a 10-mL centrifuge tube. 1 mL of the filtrate was mixed with 4 mL curcumin solution in a quartz crucible, which was dried at 55°C in a water bath kettle. The residue was then dissolved with 10 mL 95% ethanol and centrifuged at 3000 rpm for 8 min. The supernatant was measured for absorbance at a wavelength of 540 nm, and the B content was calculated based on the standard curve.
Data analysis
Statistical analysis was conducted and charts were developed using SPSS software (least significant difference, Tukey’s test at P < 0.05) and Originpro 8.6 (OriginLab Corporation, USA).
RESULTS
Plant growth and symptoms
B concentration in solution affected the seedling height, the number of leaves and the dry weight of leaf, stem, root and the total plant. The treatment B5 (5 μmol L–1) produced the greatest seedling height, the highest leaf number and the highest dry weight (including leaf, stem, root and total) of the plant (). As B concentration in solution increased from 5 to 100 μmol L–1, the seedling height, the number of leaves and the dry weight all decreased. Dry weight in the B100 (100 μmol L–1) treatment was significantly lower than that in the B5 treatment ().
The results from this experiment showed that all treatments resulted in a certain level of chlorosis (). The degrees of leaf chlorosis in this study could be described by four chlorosis levels: None, I, II and III that represented normal leaves, the first level leaf chlorosis, the second level leaf chlorosis and the third level leaf chlorosis, respectively (). The level of leaf chlorosis increased as the B concentration in culture increased. B5 and B10 only caused first-level leaf chlorosis, while B20 resulted in second-level chlorosis and B100 induced third-level leaf chlorosis (). The proportions of chlorotic leaves in B5, B10, B20 and B100 were 11.8, 26.7, 34.2 and 47.7%, respectively (). These results suggested that the proportion and the severity of chlorosis increased accordingly with the increase of B concentration in solution.
Figure 1 Classification of leaf chlorosis (“None” indicates the normal leaf without chlorosis; “I” indicates the first level of leaf chlorosis: the leaf tip was slightly chlorotic; “II” indicates the second level of leaf chlorosis: the leaf tip was completely chlorotic; “III” indicates the third level of leaf chlorosis: the chlorosis along the margins has expanded and the leaves were necrotic).
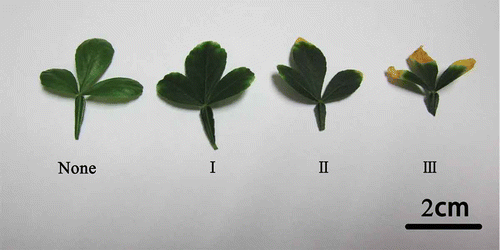
Table 1 Effects of different boron (B) treatments on the seedling height (cm), leaf numbers and dry weight (leaf, stem, root, total) (g) (mean ± standard error, SE)
Table 2 The proportion of different chlorotic levels in chlorotic leaves of different boron (B) treatments (%) (mean ± standard error, SE)
Boron concentration
Boron concentrations in different plant parts showed a common pattern of old leaves > new leaves > root > stem in all treatments. B concentration in the root of seedlings in the B5 treatment was significantly lower than in the other treatments, but as B level increased in solution, the root B concentration did not exhibit a significant difference. Stem B concentration increased evidently with the increase of B level in solution, with the highest B concentration (22.6 mg kg–1) appearing in the B100 treatment (). B concentration in new leaves showed no significant difference in all treatments, whereas B concentration in old leaves increased with the increase of B supply in solution. The highest B concentration in old leaves was 418.9 mg kg–1 in the B100 treatment, and the lowest was 214.7 mg kg–1 in the B5 treatment, which was still considered very high (). These results suggested that B tends to accumulate in old leaves. With the increase of B supply in the environment, B accumulation in old leaves increased significantly, whereas there was no excessive accumulation in new leaves. Although B concentrations in root and stem were also increased as B supply increased, the magnitude of increase remained at a relatively low level.
Boron forms in root and stem
Our results showed that bound B in root and stem was higher than semi-bound B and free B. Free B in roots was not significantly different as B concentration in solution increased from 5 to 20 μmol L–1, but significantly increased when B supply increased to 100 μmol L–1. However, it was still in a relatively low concentration (2.7 mg kg–1). There was no difference in semi-bound B concentration among different B supply levels. As B supply in the environment increased from 5 to 100 μmol L–1, bound B in roots was increased by only 1.94 mg kg–1 (). Free B and semi-bound B in stems were increased by 1.98 and 1.80 mg kg–1 respectively, with B supply increased from 5 to 100 μmol L–1. Bound B in stems showed no difference among different B treatments in this study (), indicating that B solution concentration had a limited impact on different forms of B in root and stem.
Boron forms in chlorotic leaves with different treatments
shows that free B increased from normal leaves (9.6 to 24.1 mg kg–1) to the third-level chlorotic leaves. Free B concentration in normal leaves in B100 was higher than that of B5 and B10. The first-level chlorotic leaves had 30.8 to 42.6 mg kg–1 free B, the second-level chlorotic leaves had 53.4 to 78.3 mg kg–1 and the third-level chlorotic leaves had the highest concentration of free B (141.9 mg kg–1) ().
Table 3 Free boron (B) in different chlorotic leaves with different treatments (mg kg–1 fresh weight, FW) (mean ± standard error, SE)
shows that the normal leaves had 6.4 to 12 mg kg–1 semi-bound B, and there was no significant difference among different B treatments. Semi-bound B concentration in the first-level chlorotic leaves was 20.0 to 26.0 mg kg–1; and there was no statistical significant difference among them either, and the same pattern was apparent in the second level of chlorosis. Clearly the semi-bound B concentration in the third level of chlorosis of the B100 treatment was the highest, but it still had no statistically significant difference from that in the second level of chlorosis (). These results suggest that semi-bound B in chlorotic leaves increased with the increasing degree of leaf chlorosis, though it did not increase significantly among chlorosis levels I, II and III (see semi-bound B in the B100 treatment, ).
Table 4 Semi-bound boron (B) in chlorottic leaves of different treatments (mg kg–1 fresh weight, FW) (mean ± standard error, SE)
Our results showed that the amount of bound B in normal leaves was between 10.9 and 13.0 mg kg–1, and there was no significant difference among the different treatments. Bound B in chlorotic leaves was between 21.9 and 26.3 mg kg–1; although there was no significant difference among different treatments as well as at different degrees of chlorosis, it was significantly higher than that in normal leaves (). This indicates that bound B is not critical to chlorosis.
Table 5 Bound boron (B) in chlorotic leaves of different treatments (mg kg–1 fresh weight, FW) (mean ± standard error, SE)
Different forms of B in different level leaves
showed that all B forms increased to certain extend from normal leaves to chlorotic leaves. However, among the chlorotic leaves, the bound B and the semi-bound B showed no significant difference, while the free B was significantly increased with the level of chlorosis of the leaf.
DISCUSSION
Continuous low level of B supply leads to B toxicity on Trifoliate orange seedlings
Excessive B can reduce citrus growth (Papadakis et al. Citation2004; Sheng et al. Citation2010; Gimeno et al. Citation2012). In this study, the seedling height, the number of leaves and the dry weight of plants were decreased in the B concentration range from 5 to 100 μmol L–1 (). In addition, visible symptoms of B toxicity in old leaves occurred firstly at the leaf tips, later along the margins and finally the leaves were necrotic. Although leaf chlorosis is the typical visible symptom of B toxicity (Nable et al. Citation1997) and has been described in barley (Hordeum vulgare L.), wheat (Triticum aestivum L.) (Reid and Fitzpatrick Citation2009), summer squash (Cucurbita pepo L.) (Francois Citation1992), citrus (Papadakis et al. Citation2003; Han et al. Citation2009; Sheng et al. Citation2010) and others, leaf chlorosis appeared at 5 μmol L–1 B level in this study (), which is unexpected but very interesting. It seems inconsistent with the previously reported data that citrus seedlings grew normally at the 5 μmol L–1 B level and B toxicity symptoms appeared when the external B concentration was over 10 μmol L–1 (Papadakis et al. Citation2003), but our results were acceptable to some extent because Mei et al. (Citation2011) took 0.25 μmol L–1 as the normal B level for Trifolicate orange seedlings. In this study, B concentrations in old leaves were very high in all the treatments, which were over 200 mg kg–1, even at the lowest level of B treatment (214.7 mg kg–1 in B5 treatment, ), which is at a very high level that obviously surpassed the toxicity borderline in citrus (200 mg kg–1) (Obreza and Morgan Citation2008). Furthermore, with the chlorosis aggravated, B concentration in these leaves was increased. In this study, B concentration in old leaves that contained the third level of chlorosis in the B100 treatment was as high as 418.9 mg kg–1 (). So, from the B concentration data in this experiment, B was continuously accumulated in old leaves regardless of whether the B content in the environment was low (5 μmol L–1) or high (100 μmol L–1). It is reported that B is absorbed by plants as an uncharged molecule, and it could easily cross the phospholipid bilayer or via water channel proteins such as NIP5;1 into cells (Miwa and Fujiwara Citation2010). Boric acid has a high capacity of permeability that might cause excess B absorption. Papadakis et al. (Citation2003) also reported B in basal leaves could be accumulated as much as 1286 mg kg–1 dry weight. In addition, the growth of the seedlings is very slow. In the whole period of cultivation (117 d), the average dry weight of the whole plant was only 1.26 g in the 5 μmol L–1 B treatment (). Besides, visible symptoms of B toxicity do not appear in the root, because B concentration in the root remains relatively low compared to that in leaves even at very high levels of B supply (Nable et al. Citation1997). Based on the tissue B concentration data, root B concentrations were kept at a very low level (< 30 mg kg–1) in all treatments, especially B5 (< 15 mg kg–1) (). This might be the reason why it could absorb B from the environment continuously. Furthermore, as is generally known, retranslocation of B is negligible in many species. A recent study revealed that boron in ‘Newhall’ navel orange (Citrus sinensis Osb.) could transport from old leaves to new leaves, but not in great amounts (Liu et al. Citation2012). So, constant B absorbed by roots was mostly uploaded to and accumulated in old leaves, which agreed with a previous report (Marschner Citation2012).
Boron concentration, distribution and utilization
The uptake of B has long been considered to be a passive process that is not facilitated by transport proteins. However, recent physiological studies revealed the presence of channel-mediated facilitated diffusion and energy-dependent active transport against concentration gradients in B transport systems (Miwa and Fujiwara Citation2010). With B uptake from root, it mainly exists as borate, loosely bound to other ligands, e.g. the carbohydrate moiety of glycosylated membrane proteins, sugars and sugars alcohols, cell wall components with the proper vic, cis-diol groups and bound to the pectic fraction, especially to rhamnogalacturonan II (RG II) (Goldbach et al. Citation2000). Dannel et al. (Citation1998) separated B in root and leaf into two compartments: water insoluble residue (WIR) and cell sap. The WIR B was closely bound to cell wall polymers and partially identified as a cross-link between chains of rhamnagalacturonan II (RG II) in the pectic fraction of the cell wall. The cell sap B mainly represents the soluble B in the symplasm, which seems to be directly available for possible physiological functions in the cell. In this experiment, we used the continuous extraction method of Du et al. (Citation2002) to fractionate B in root, stem and leaves into free B, semi-bound B and bound B. Free B is water soluble and can pass the cell membrane freely. It interacts with monosaccharides, and has a vicinal hydroxyl group in the cis position (Chuda et al. Citation1997). Semi-bound B might be the primary form of B before it is integrated into the cell wall as a structural component. It represents soluble B in the symplast that binds with the monosaccharides having vicinal hydroxyl groups in a cis configuration (Du et al. Citation2001), which seems to be directly available for possible physiological functions in the cell. Moreover, it can reflect the capability of plants to use free B and closely connect with B mobility in the plant (Dannel et al. Citation1998, Citation1999). Bound B is B bound in the cell wall probably with pectin polysaccharides, especially to RG-II. It is a critical B form for the stability of cell walls (Hu and Brown Citation1994), and represents the requirement of B by plants.
Our results show that bound B concentration was higher than semi-bound B and free B in root. Both semi-bound B and free B in root changed slightly (1.25 and 2.31 fold respectively) among B supply levels from 5 to 100 μmol L–1 (), implying that roots retained little B in semi-bound B and free B forms and responded little to B oversupply. Besides, bound B in root increased only by a factor of 1.94; similar results were observed in sunflower (Helianthus annuus L. cv. Frankasol) by Dannel et al. (Citation1998), who found that WIR B concentration in root increased by a factor of 2.8 when the external B supply increased from 0.1 to 1600 μmol L–1. Some reports claimed that cell wall pectin content was correlated with the variability in B requirements between plant species (Hu et al. Citation1996). The relative proportion of cell wall-bound B varies with species, organs and B supply levels. It suggests that there is a constant B demand for cell wall formation and a limited number of binding sites for B, and therefore the cell wall does not contribute effectively to detoxify excessive B taken up by plants by providing additional binding sites in response to B toxicity (Dannel et al. Citation1998).
Boron forms and boron toxicity
When the B concentration in solution increased from 5 to 100 μmol L–1, the level and the proportion of leaf chlorosis increased (). B concentration in old leaves significantly increased with increased B supply, and B concentration in B100 was up to 418.9 mg kg–1 (). The results of bound B and semi-bound B in leaves significantly increased from normal leaves to chlorotic leaves, but showed little change among different levels of chlorotic leaves ( and ). A similar result was reported by Dannel et al. (Citation1998) in sunflower and Liu (Citation2011) in citrange [C. sinensis (L.) Osb. × P. trifoliata (L.) Raf.], who found that the external B supply increased from 0.1 to 1600 μmol L–1 and 0.5 to 50 mg L–1, respectively. Our results suggested that bound B and semi-bound B did not contribute effectively to detoxify excessive B absorbed by plants. Moreover, the free B content in leaves was much higher than bound B and semi-bound B (), and increased significantly as the leaf chlorosis was aggravated (), hinting that leaf chlorosis was closely related with excessive levels of free B. Goldbach et al. (Citation2000) suggested that the amount of easily extractable B including free boric or soluble B complexes might be used for diagnosing the B status of plants.
CONCLUSIONS
With increased external B supply, most B in roots and stems exists in bound B and semi-bound B forms, but B in leaves is mostly in the free B form, which is closely related to leaf chlorosis caused by B toxicity.
ACKNOWLEDGMENTS
This research project was financially supported by the Chinese State Natural Science Foundation (41271320) and the Fundamental Research Funds for the Central Universities in China (2011PY150; 2013YB093).
REFERENCES
- Cakmak I, Römheld V 1997: Boron deficiency-induced impairments of cellular functions in plants. Plant Soil, 193, 71–83. doi:10.1023/A:1004259808322
- Chuda Y, Ohnishi-Kameyama M, Nagata T 1997: Identification of the forms of boron in seaweed by 11B NMR. Phytochemistry, 46, 209–213. doi:10.1016/S0031-9422(97)00284-7
- Dannel F, Pfeffer H, Römheld V 1998: Compartmentation of boron in roots and leaves of sunflower as affected by boron supply. J. Plant Physiol., 153, 615–622. doi:10.1016/S0176-1617(98)80212-5
- Dannel F, Pfeffer H, Römheld V 1999: Distribution within the plant or compartmentation does not contribute substantially to the detoxification of excess boron in sunflower (Helianthus annuus). Funct. Plant Biol., 26, 95–99.
- Du CW, Wang YH, Xu FS 2001: Study on relation between boron efficiency and column chromatography sugar in different rape cultivars (Brassica napus). Plant Physiol. Commun., 37, 508–510.
- Du CW, Wang YH, Xu FS, Yang YH, Wang HY 2002: Study on the physiological mechanism of boron utilization efficiency in rape cultivars. J. Plant Nutr., 25, 231–244. doi:10.1081/PLN-100108832
- Francois LE 1992: Effect of excess boron on summer and winter squash. Plant Soil, 147, 163–170. doi:10.1007/BF00029068
- Gimeno V, Simón I, Nieves M, Martínez V, Cámara-Zapata JM, García AL, García-Sánchez F 2012: The physiological and nutritional responses to an excess of boron by Verna lemon trees that were grafted on four contrasting rootstocks. Trees-Struct. Funct, 26, 1513–1526. doi:10.1007/s00468-012-0724-5
- Goldbach HE, Wimmer MA, Findeklee P 2000: Discussion paper: boron—How can the critical level be defined?. J. Plant Nutr. Soil Sci., 163, 115–121. doi:10.1002/(SICI)1522-2624(200002)163:1<115::AID-JPLN115>3.0.CO;2-#
- Guidong L., Cuncang J., Yunhua W. 2011: Distribution of boron and its forms in young “Newhall” navel orange (Citrus sinensis Osb.) plants grafted on two rootstocks in response to deficient and excessive boron. Soil Sci. Plant Nutr., 57, 93–104. doi:10.1080/00380768.2010.551299
- Günes A, Alpaslan M 2000: Boron uptake and toxicity in maize genotypes in relation to boron and phosphorus supply. J. Plant Nutr., 23, 541–550. doi:10.1080/01904160009382038
- Han S, Tang N, Jiang HX, Yang LT, Li Y, Chen LS 2009: CO2 assimilation, photosystem II photochemistry, carbohydrate metabolism and antioxidant system of citrus leaves in response to boron stress. Plant Sci., 176, 143–153. doi:10.1016/j.plantsci.2008.10.004
- Hoagland DR, Arnon DI. 1950: The water-culture method for growing plants without soil. California Agricultural Experiment Station Circular 347. The college of Agriculture, University of California, Berkeley, CA.
- Hu H, Brown PH 1994: Localization of boron in cell walls of squash and tobacco and its association with pectin (evidence for a structural role of boron in the cell wall). Plant Physiol., 105, 681–689.
- Hu H, Brown PH, Labavitch JM 1996: Species variability in boron requirement is correlated with cell wall pectin. J. Exp. Bot., 47, 227–232. doi:10.1093/jxb/47.2.227
- Jiang CC, Wang YH, Liu GD, Xia Y, Peng SA, Zhong BL, Zeng QL 2009: Effect of boron on the leaves etiolation and fruit fallen of newhall navel orange. Plant Nutr. Fert. Sci, 15, 656–661.
- Ling LL, Peng LZ, Chun CP, Cao L, Jiang CL 2010: Characteristics analysis of microelement contents in newhall navel orange leaves in southern Jiangxi province. Acta Hortic. Sin., 37, 1388–1394.
- Liu GD, Wang RD, Wu LS, Peng SA, Wang YH, Jiang CC, 2012: Boron distribution and mobility in navel orange grafted on citrange and trifoliate orange [J]. Plant Soil, 360, 123–133.
- Marschner H 2012: Mineral nutrition of higher plants. Third edition. Academic Press, London.
- Matoh T 1997: Boron in plant cell walls. Plant Soil, 193, 59–70. doi:10.1023/A:1004207824251
- Mei L, Sheng O, Peng SA, Zhou GF, Wei QJ, Li QH 2011: Growth, root morphology and boron uptake by citrus rootstock seedlings differing in boron-deficiency responses. Sci. Hortic., 129, 426–432. doi:10.1016/j.scienta.2011.04.012
- Miwa K, Fujiwara T 2010: Boron transport in plants: co-ordinated regulation of transporters. Ann. Bot., 105, 1103–1108. doi:10.1093/aob/mcq044
- Nable RO, Bañuelos GS, Paull JG 1997: Boron toxicity. Plant Soil, 193, 181–198. doi:10.1023/A:1004272227886
- O’Neill MA, Eberhard S, Albersheim P, Darvill AG 2001: Requirement of borate cross-linking of cell wall rhamnogalacturonan II for Arabidopsis growth. Science, 294, 846–849. doi:10.1126/science.1062319
- Obreza T, Morgan K 2008: Nutrition of Florida citrus trees. Soil and Water Sci. 2th edition. IFAS, SL 253. University of Florida, Gainesville.
- Papadakis IE, Dimassi KN, Bosabalidis AM, Therios IN, Patakas A, Giannakoula A 2004: Boron toxicity in ‘Clementine’mandarin plants grafted on two rootstocks. Plant Sci., 166, 539–547. doi:10.1016/j.plantsci.2003.10.027
- Papadakis IE, Dimassi KN, Therios IN 2003: Response of two citrus genotypes to six boron concentrations: concentration and distribution of nutrients, total absorption, and nutrient use efficiency. Aust. J. Agric. Res., 54, 571–580. doi:10.1071/AR02163
- Reid R, Fitzpatrick K 2009: Influence of leaf tolerance mechanisms and rain on boron toxicity in barley and wheat. Plant Physiol., 151, 413–420. doi:10.1104/pp.109.141069
- Sheng O, Zhou GF, Wei QJ, Peng SA, Deng XX 2010: Effects of excess boron on growth, gas exchange, and boron status of four orange scion–rootstock combinations. J. Plant Nutr. Soil Sci., 173, 469–476. doi:10.1002/jpln.200800273
- Thellier M, Duval Y, Demarty M 1979: Borate exchanges of Lemna minor L. as studied with the help of the enriched stable isotopes and of a (n, α) nuclear reaction. Plant Physiol., 63, 283–288. doi:10.1104/pp.63.2.283
- Wang RD, Jiang CC, Liu GD, Wang YH, Peng SA, Zeng QL 2011: Status and analysis on available boron content in soil of Gannan navel orange orchards. South China Fruits, 40(1–3), 7.
- Zhou KB, Xia RX 2005: The proceedings and tendencies in the study on the choice of rootstocks for citrus in China. Chin. Agric. Sci. Bull., 21, 213–218.