Abstract
Boron (B) is an essential microelement for the growth and development of plants, and B deficiency affects many biochemical and physiological processes. Brassica napus L. has a high demand for B and is extremely sensitive to B deficiency. Seed yields and oil quality of B. napus are often limited by the low availability of B in soils. Developing new cultivars of B. napus with high B efficiency is therefore required, which requires a greater understanding of responses to B deficiency. Significant genotypic differences in response to low soil B have been observed among varieties of B. napus. B-efficient genotypes can grow and yield normally and usually have a larger root system than B-inefficient genotypes at low B conditions. The mechanisms for B efficiency in B. napus are attributed to B absorption, transportation and utilization. In addition, the cell wall component plays an important role in the tolerance of B. napus to B deficiency, and the B-efficient line presents fewer B-binding sites in the cell walls compared with the B-inefficient line. Genetic and proteomic analyses in B. napus revealed the modulation of a complex network in response to B deficiency. This review gives a comparative overview of the physiological and genetic responses to B deficiency in B. napus and discusses the possible underlying mechanisms of B efficiency.
INTRODUCTION
The physiological role of boron (B) involved in the formation and structural integrity of the cell wall by cross-linking rhamnogalacturonan II (RG-II) has been clearly demonstrated (Ishii and Matsunaga Citation1996; Kobayashi et al. Citation1996; O’Neill et al. Citation1996; Ishii et al. Citation2001; O’Neill et al. Citation2001; O’Neill et al. Citation2004). In addition, roles of B in plant membranes (Schon et al. Citation1990; Wimmer et al. Citation2009), pollen tube growth (Cheng and Rerkasem Citation1993) and signaling (Redondo-Nieto et al. Citation2012) have been reported; however, the mechanisms for most of these functions are still unclear. For a long time, B uptake in higher plants was believed to be a passive process (Hu and Brown Citation1997). Increasing evidence suggests that B transport can also be an active process (Hu et al. Citation1997; Stangoulis et al. Citation2001; Dannel et al. Citation2002), and the B transporters have been identified in Arabidopsis (Takano et al. Citation2002, Citation2006; Tanaka et al. Citation2008). Boron uptake in Arabidopsis root under B limitation has been shown to be co-ordinated by regulation of NIP5;1 and BOR1. AtNIP5;1 facilitates the influx of B into root cells from the soil, and then AtBOR1 exports B out of the cells toward the xylem (Takano et al. Citation2008; Tanaka and Fujiwara Citation2008; Miwa and Fujiwara Citation2010).
Brassica napus L. is one of the world’s major oilseed crops and provides oil for human consumption and industrial co-products, including bio-fuels (Meyer Citation2009). In China, the planted area of B. napus is more than 7.37 million hectares, accounting for 22.9% of the world production area (FAO Citation2010). Among the crop species, dicotyledons including B. napus, cotton (Gossypium spp.) and sugar beet (Beta vulgaris L.) are generally considered to have a high requirement for B and are sensitive to B deficiency (Marschner Citation2012). However, B deficiency for crop yield is a worldwide agricultural problem (Shorrocks Citation1997). The symptom of “flowering without seed setting” in B. napus was first reported in China in the 1970s, and was soon proven to be caused by B deficiency. Since then, a series of studies on B nutrition and the application of B fertilizers have been reported (Wang et al. Citation2007). For the lower levels of RG-II in the cell walls, a lower requirement for B and less sensitivity to B deficiency were observed in graminaceous species than in dicotyledons (Marschner Citation2012; Chormova et al. Citation2014). However, B deficiency affecting grain set has also been reported in rice (Oryza sativa L.) (Lordkaew et al. Citation2013), maize (Zea mays L.) (Lordkaew et al. Citation2011), wheat (Triticum aestivum L.) and barley (Hordeum vulgare L.) (Cheng and Rerkasem Citation1993; Rerkasem et al. Citation1993; Rerkasem and Jamjod Citation1997a, Citation2004). The critical concentrations of soil B for B. napus and wheat to grow normally have been estimated at 0.58 and 0.4 mg kg−1 of hot water-extracted B (HWB), respectively (Rerkasem and Jamjod Citation2004; Zou et al. Citation2008).
The application of B fertilizers can alleviate the problem of B deficiency in soils. However, B is a limited and non-renewable mineral resource, and excessive applications can cause environmental problems. Breeding crop cultivars with enhanced B efficiency is a more sustainable way to manage the problem of B deficiency in soils. B efficiency is defined as the ability of a genotype to grow well and produce a high yield in soils with a given HWB which would be deficient for standard cultivars (Graham Citation1984). Studies on B efficiency in B. napus began in the 1990s, and have since spanned germplasm screening, proteomics analysis, genetic analysis and the cloning and characterization of B transporter genes. Here, we review the recent advances in these studies of B efficiency in B. napus and discuss the underlying mechanisms.
NATURAL VARIATION IN B EFFICIENCY
Genotypic differences have been observed among the B. napus lines in their responses to B deficiency in several studies. A two-pace method of seedling biomass screens and seed yield assessments at maturity was used by Xu et al. (Citation2002b) to evaluate the B efficiency of 210 B. napus lines. Firstly, all lines were grown at B levels of 0.10 mg kg−1 (B1) and 1.0 mg kg−1 (B3) in pots for 35 d, and the ratio of B1:B3 dry weight was calculated. Lines with ratios of < 0.50 or > 0.90 were then grown at B levels of 0.25 mg kg−1 (B2) and 1.0 mg kg−1 (B3) and their seed yields were determined. Boron efficiency coefficients (BEC, the ratio of seed yield at B2:B3) of these lines were calculated. Lines with BEC > 0.90 were deemed to be the B-efficient lines, and those with BEC < 0.10 were defined as the B-inefficient lines. In total, eight B-efficient lines and two B-inefficient lines were obtained from the initial 210 B. napus lines. Xue et al. (Citation1998) used a sandy loam soil to determine the genotypic range in B efficiency of 16 B. napus lines, and found that leaf area was the early indicator which correlated best with subsequent seed yield and so might be useful for evaluating the responses of lines to low B supply. Stangoulis et al. (Citation2000a, Citation2000b) also observed that genotypic variation existed in oilseed rape (Brassica napus L.) using field, pot and solution methods.
There are significant genotypic differences in plant phenotype and growth response to B deficiency between the B-efficient and B-inefficient lines. The B-efficient lines '9589' and '9118' grew well and produced seed yield at low B stress, while the B-inefficient line '95105' showed the typical B-deficient symptom “flowering without seed setting” and '9141' even died at an early stage because of the severe B deficiency (). At the seedling stage, the B-inefficient lines Bakow (B), Westar 10 (W) and Huyou 16 (HY16) showed small leaves with dark green color and downward curvature at the margins, and even died at the critical 0.25 µM B concentration. On the contrary, the B-efficient lines Huashuang 5 (HS5), Qingyou 10 (QY10) and Huyou 18 (HY18) did not show these dramatic symptoms of B deficiency (). There was no significant difference in the root development of the B-efficient line QY10 and the B-inefficient line W at normal B supply. However, the root development was significantly inhibited by B deficiency, and the root of the B-inefficient line W was more sensitive to B deficiency than that of the B-efficient line QY 10 ().
Figure 1 Response of boron (B)-efficient and B-inefficient lines of Brassica napus L. to B stress. (a) seed setting at low B; (b) shoot growth at low B (0.25 μM); (c) root growth at normal B (+B; 25 μM) and low B (–B; 0.25 μM). HS5: Huashuang 5, QY10; Qingyou 10, B: Bakow, W: Westar 10, HY18: Huyou 18, HY16, Huyou 16.
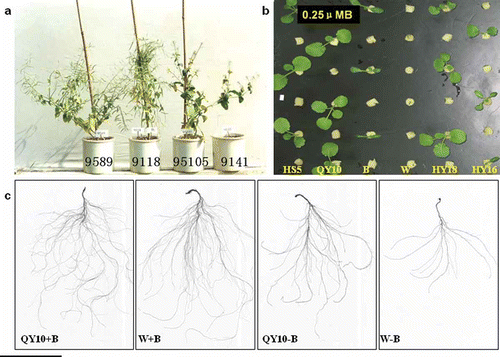
PHYSIOLOGICAL MECHANISM OF B EFFICIENCY
Root system and B uptake efficiency
As the main organ for plants to take up nutrients, the ability of the root to access nutrients from soils depends on the root physiological and morphological properties (Lynch Citation1995). Differences in root morphology among a panel of B. napus genotypes will affect B uptake, resulting in differences in the growth and development of plants under low-B conditions. Xu et al. (Citation2002b) observed that the root lengths of the two genotypes of B. napus were decreased with the decline of B concentrations in solution. In our studies, the root elongation of a B-inefficient line, '95105', was much more restricted than that of the B-efficient line '9590' under low B concentrations. Moreover, root volume, total absorption area and active absorption area were also lower in '95105' than in '9590'. Yang et al. (Citation2013) also reported that the B-efficient lines HZ and HQ had longer root length and larger root surface and root volume than the B-inefficient lines LW and LB at the level of 0.25 µM B. The larger root system of the B-efficient line could sustain a stronger ability to absorb B from soil when the external B is deficient. Yang et al. (Citation2013) used 10B as a tracer to investigate B uptake in B. napus, and found that the total B and 10B accumulations of the B-efficient lines HZ and HQ were significantly higher than those of the B-inefficient lines LW and LB under low-B conditions.
Further evidence that root function directly affects the B efficiency of B. napus has been shown by a grafting experiment (Yang et al. Citation2013). The B-efficient line HZ and the B-inefficient line LW were used to obtain four combinations of grafted plants, HZ/HZ, LW/HZ, HZ/LW and LW/LW (scion/stock). When HZ was used as the stock, the grafted plants showed normal growth associated with higher shoot dry weight and the amount of 10B transferred to the shoots regardless the scion. However, the role of the root in regulating B efficiency in B. napus is still not fully clear. Thus, it is not easy to determine whether B efficiency is controlled by a large root system or by higher levels of expression of BnBOR1s and BnNIP5;1 in the root. It is possible that there are additional genes encoding B transporters, and transcription factors regulating the expressions of BnBOR1s and BnNIP5;1, or regulating the development of the root. For example, a novel gene (PSTOL1) regulating root growth to enable plants to acquire more phosphorus and other nutrients was reported recently in rice (Gamuyao et al. Citation2012).
B translocation and utilization
Perhaps uniquely among the essential plant nutrients, B has restricted mobility in the majority of plant species while being freely mobile in the phloem of some species (Brown and Shelp Citation1997). The ability to re-translocate B from older tissues to young leaves enables some plant species to avoid deficiency when external B supply is low. Interestingly, Xu et al. (Citation2002b) reported that a B-efficient line had a higher B re-translocation index than a B-inefficient line under B limitation at the bolting stage, suggesting it was able to avoid deficiency by recycling B into new growing regions. Genotypes of wheat that are tolerant to B deficiency also have greater capacity to recycle B to meet reproductive demand than inefficient cultivars (Rawson Citation1996; Huang et al. Citation2001).
B plays an important role in cell wall synthesis and integrity. Irregular cell wall formation was observed in B. napus under B limitation, but cell walls could regenerate completely when B was resupplied. Moreover, the capacity for cell wall regeneration of a B-efficient line was stronger than that of a B-inefficient line (Yang et al. Citation2002). Studies have shown that B in cell walls is present as a borate ester of RG-II, a polysaccharide in pectin. In the bor1-1 mutant of Arabidopsis, B concentrations in the cell wall of shoot tissues were lower than those in the wild type (WT); however, the sugar composition of cell wall fractions was similar with WT, suggesting that the polysaccharide composition in the cell wall was not strongly affected by the bor1-1 mutation (Noguchi et al. Citation2003). Unlike the bor1-1 mutant, the differences in the concentration of cell wall pectin have been observed in B. napus lines of contrasting B efficiency. The B-inefficient line Westar 10 had a greater proportion of cell wall pectin and higher cell wall glycosyls contents than the B-efficient line Qingyou 10 (). Moreover, the expression of Kdo-8-p synthase gene (KDOPS), regulating the biosynthesis of a constituent monosaccharide 2-keto-3-deoxy-D-manno-octulosonic acid (Kdo) in the side chain C of RG-II (Delmas et al. Citation2008), was higher in Westar 10 than in Qingyou 10. These results imply that Westar 10 might have more B-binding sites in its cell wall and thereby a greater B requirement for normal growth and development. However, the concentrations of B in both pectin and cell wall fractions of Westar 10 were significantly lower than those of Qingyou 10 when grown under low-B conditions (). Therefore, the proportion of pectin in the cell wall and the capacity to distribute B to it might be key factors affecting the B efficiency in B. napus (Pan et al. Citation2012). A positive relationship between the concentration of cell wall pectin and tissue-B requirement has been reported in different species (Hu et al. Citation1996). Recently, a B efflux transporter, BOR2, was reported to facilitate efficient borate cross-linking of RG-II in cell walls and root cell elongation under B deficiency in Arabidopsis. The proportion of B-crosslinked RG-II in bor2 mutants was reduced under low-B conditions (Miwa et al. Citation2013).
Figure 2 Glycosyl compositions of boron (B)-efficient line, Qingyou 10, and B-inefficient line, Westar 10, under normal and low-B conditions. Gal: Galactose, Ara: Arabinose, GalA: Galacturonic acid, Xyl: Xylose, Rha: Rhamnose, GlcA: Glucuronic Acid, Fuc: Fucose, Man: Mannose. Source: Pan et al. (Citation2012).
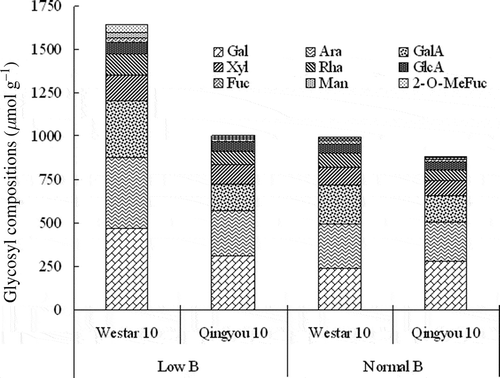
Table 1 Distribution of boron (B) in B-efficient line Qingyou 10 and B-inefficient line Westar 10 under low-B conditions
GENETIC BASIS OF B EFFICIENCY
Differentially expressed proteins responding to B limitation
B deficiency disturbs the equilibrium of reactive oxygen species (ROS) in plants and triggers an oxidative burst that can stress membranes or other cell compartments (Cakmak and Römheld Citation1997). The activities of peroxidase (POD), catalase (CAT) and superoxide dismutase (SOD) and the concentration of malonyl dialdehyde (MDA) are usually used as indicators to evaluate the ability to resist environmental stress in plants. Under B limitation, the activities of SOD and CAT decreased, and the concentration of MDA and membrane permeability increased in the B-inefficient lines (Xu and Wang Citation2007).
Proteomic analyses of B. napus roots in response to long-term (12 d) and short-term (1–5 d) B deficiency have revealed effects on proteins involved in carbohydrate and energy metabolism, signaling and regulation, cell wall structure, protein process, amino acid and fatty acid metabolism, nucleic acid metabolism, antioxidant activity and detoxification of reactive oxygen species, defense-related proteins and transporter (Wang et al. Citation2010, Citation2011b). A time course proteomic analysis of B. napus root found the energy metabolism pathway and certain biosynthesis pathways were maintained at a relatively low level, while signaling, regulation and stress response pathways were dramatically increased in the absence of B (Wang et al. Citation2011b). Similar results were reported in other species, such as Arabidopsis (Chen et al. Citation2014), Lupinus albus L. geranium (Pelargonium graveolens L.Her.) (Alves et al. Citation2011) and geranium (Mishra et al. Citation2009). Based on these B stress-induced proteins, it can be inferred that a stable cell wall structure, resistance to oxidative damage and a complex signaling network might contribute to the tolerance of B deficiency (Wang et al. Citation2011b).
Cloning and characteristics of B transporter genes
The first B transporter, AtBOR1, was identified as having a role in B xylem loading under low-B conditions in Arabidopsis (Takano et al. Citation2002). Recently, BOR1 has been reported to have a similar function in other species, including OsBOR1 in rice (Nakagawa et al. Citation2007), TaBOR1 in wheat (Leaungthitikanchana et al. Citation2013), VvBOR1 in grape (Vitis vinifera L.) (Perez-Castro et al. Citation2012) and CmBOR1 in Citrus macrophylla Wester. (Cañon et al. Citation2013). In B. napus, six genes, BnBOR1;1a, BnBOR1;1c, BnBOR1;2a, BnBOR1;2c, BnBOR1;3a and BnBOR1;3c, have now been isolated and characterized (Sun et al. Citation2012). The phylogenic analysis revealed that the six BnBOR1s were the orthologues of AtBOR1 and were divided into three groups. Each group comprised two members, which were from Brassica rapa L. and Brassica oleracea L., respectively, implying that BnBOR1s were formed during the triplication and allotetra-ploidization of the B. napus. BnBOR1;3a and BnBOR1;3c shared the same number (11) and positioning of introns as AtBOR1, and the other four BnBOR1s had only nine introns, suggesting that BnBOR1s had undergone a divergent evolution pattern in the genome of B. napus after divergence from Arabidopsis (Sun et al. Citation2012). However, there were no significant differences in the expression levels of these genes between the B-efficient lines HZ and HQ, and the B-inefficient lines LW and LB at the seedling stage, implying that the B efficiency at B. napus seedling might be regulated by the other B transporters, such as NIP5;1 and NIP6;1, or unknown genes (Yang et al. Citation2013).
Identifying and dissecting quantitative trait locus (QTL) for B efficiency
Genetic controls of B efficiency have been reported to be monogenic in celery (Apium graveolens L.) and tomato (Lycopersicon esculentum Mill.), and polygenic in sunflower (Helianthus annuus L.) and wheat (Rerkasem and Jamjod Citation1997b). For B. napus, the phenotype of F1 population, derived from the cross between a B-efficient line, Qingyou 10, and a B-inefficient line, Bakow, had an identical phenotype with Qingyou 10 under B limitation, indicating that B efficiency in B. napus is a dominant trait (Xu et al. Citation2002a). The frequency distribution of seed yield in the F2 population showed a bimodal distribution, with the ratio of B-efficient individuals to B-inefficient individuals fitting an expected Mendelian ratio of 3:1 under low-B conditions. A major gene, BE1, was mapped on the ninth linkage group, equivalent to the A2 linkage group of B. napus, and explained 64.0% of the phenotypic variance (Xu et al. Citation2001).
Genetic analyses for seed yield and yield-related traits under low-B conditions have been reported in two populations (Zhao et al. Citation2012). Co-localizations of the QTLs for BEC and seed yield located on A2 were observed in both the TN double haploid (DH) (Tapidor × Ningyou 7) and BQ DH (Qingyou 10 × Bakow) populations. The phenotypic variations of the QTLs detected using the DH populations were significantly lower than that of BE1, which might be because of the lack of the dominance effect. In contrast, additive and dominance effects of alleles in the F2 and F2:3 populations could contribute to the phenotypic variation (Shi et al. Citation2009). Furthermore, due to the small population size and low marker density, the phenotypic variation explained by BE1 might be overestimated.
The relationship between B efficiency and plant growth has also been investigated by QTL analysis (Shi et al. Citation2012). The QTLs for shoot boron accumulation (SBA) have usually been observed to overlap with QTLs for plant growth [i.e. shoot dry weight (SDW) and root dry weight (RDW)] under normal B conditions. Unfortunately, there was no region of overlap between the QTL for SBA and the QTL for plant growth traits under low-B conditions. In silico mapping of these QTLs has revealed the co-location of orthologues of some B-related genes of Arabidopsis (Shi et al. Citation2012).
Zhao et al. (Citation2008) reported a second B-efficient locus, BnBE2, responsible for B utilization efficiency at the seedling stage and screened six molecular markers linking to this locus by the bulked segregant analysis (BSA) method. The chromosome region of BnBE2 was mapped on C4 of the B. napus genetic map by comparing linked marker between the TN DH and BQ DH genetic maps.
The QTLs for B efficiency-related traits, summarized in , are distributed on 15 chromosomes except for A8, A9, A10 and C1. For all traits except for root B accumulation (RBA) and the ratio of RBA to SBA [(R/S)BA], more than one QTL was detected. Most of these loci affected the B efficiency at either the seedling or maturity stages, except for the region on the top of chromosome C3, which simultaneously controlled RBA and SDW at the seedling stage and plant height (PH) at the maturity stage. It is not surprising that the loci affecting B efficiency at the seedling and maturity stages were inconsistent because different molecular mechanisms are being expressed. Taken together, these results offer insights into the genetic basis of B efficiency in B. napus and provide a basis to fine map in the future, and ultimately to clone the major QTLs for B efficiency.
Figure 3 QTLs for boron (B) efficiency related traits detected at Brassica napus L. seedling stage (left) and maturity stage (right). IPRL: increment of primary root length, SDW: shoot dry weight, RDW: root dry weight, R/S: ratio of RDW to SDW, SBA: shoot boron accumulation, RBA: root B accumulation, (R/S) BA: the ratio of RBA to SBA, BEC: boron efficiency coefficient, SY: seed yield, PH: plant height, BN: branch number, PN: pod number, SN: seed number, SW: seed weight, QTL; quantitative trait locus, TN DH population, derived from the cross between Tapidor and Ningyou 7; BQ DH population, derived from the cross between Qingyou 10 and Bakow.
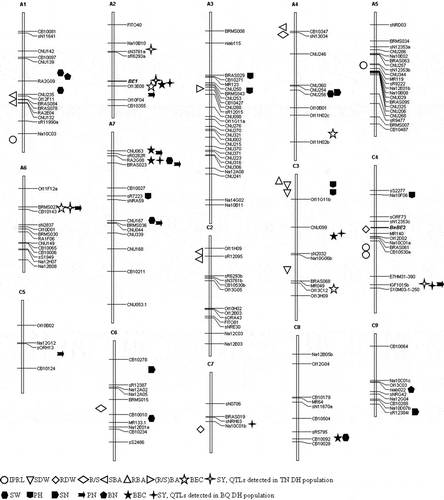
CONCLUSION
The response of B. napus to B deficiency is genotype-dependent and relates to B uptake, translocation and requirement in the cell walls, biomass allocation and plant physiological development. Proteomics analyses have revealed that B deficiency affects a series of metabolic processes, notably carbohydrate metabolism, antioxidant activity and cellar detoxification of reactive oxygen species, cell wall development and signaling networks. Genetic analyses have shown that B efficiency of B. napus is polygenic. The QTLs for B efficiency detected in different populations and environments have revealed the genetic basis of B efficiency in B. napus and have provided the basis for molecular breeding by marker-assisted selection.
PROSPECTIVE
Large knowledge gaps remain in understanding the mechanisms of B efficiency in B. napus under low-B conditions. However, map-based cloning will play a major role in cloning QTLs. The publication of the B. rapa genome sequence (Wang et al. Citation2011a) has provided more information to screen for candidate genes within target QTL. With the global development of bioinformatics, analysis combining QTL mapping based on bin maps developed using next-generation sequencing (NGS) or SNP chips, with functional genomics, proteomics, metabolomics and transcriptomics, provides a powerful strategy for accelerating the cloning of QTL (Trick et al. Citation2012).
With the rapid development of NGS technologies, it is now feasible to genotype large collections of B. napus for genome-wide association study (GWAS) (Yan et al. Citation2010), and to predict the most favorable alleles in a diverse panel of B. napus germplasm for genetic improvement and marker assisted selection (MAS) for B efficiency. GWAS would facilitate the pyramiding of multiple B-efficient loci with small effects from genetic backgrounds into a single B. napus line. Besides the designed molecular breeding, GWAS will greatly improve our understanding of the genetic architecture of the B efficiency in B. napus.
Six B transporter genes (BnBOR1s) have now been isolated in B. napus and showed differential expression patterns (Sun et al. Citation2012). However, the specific functions of these genes still need to be clarified. Additional B transporters, such as NIP5;1 (Takano et al. Citation2006) and NIP6;1 (Tanaka et al. Citation2008), still need to be isolated and characterized in B. napus.
Proteomic analysis provides a crucial and complementary tool to dissect molecular mechanisms underlying crop adaptation to mineral nutrient deficiency (Liang et al. Citation2013). Wimmer et al. (Citation2009) isolated B-interacting proteins using a proteomic technique in Arabidopsis and maize, supporting the hypothesis that B plays a role in plant membranes through the cross-linking of glycoproteins. To our knowledge, there are no reports about membrane-associated proteins in B. napus for B efficiency, and these should be targets of future work.
ACKNOWLEDGMENTS
This work was supported serially by grants from the Natural Science Foundation of China (31372129, 31172018, 30971861, 30771283, 30170549, 39770439), and the Fundamental Research Funds for the Central Universities (2011PY150). We greatly acknowledge the effort of Martin R. Broadley (University of Nottingham) for editing the language of the manuscript.
REFERENCES
- Alves M, Moes S, Jenö P, Pinheiro C, Passarinho J, Ricardo CP 2011: The analysis of Lupinus albus root proteome revealed cytoskeleton altered features due to long-term boron deficiency. J. Proteomics, 74, 1351–1363. doi:10.1016/j.jprot.2011.03.002
- Brown PH, Shelp BJ 1997: Boron mobility in plants. Plant Soil, 193, 85–101. doi:10.1023/A:1004211925160
- Cakmak I, Römheld V 1997: Boron deficiency-induced impairments of cellular functions in plants. Plant Soil, 193, 71–83. doi:10.1023/A:1004259808322
- Cañon P, Aquea F, Rodríguez-Hoces de la Guardia A, Arce-Johnson P 2013: Functional characterization of Citrus macrophylla BOR1 as a boron transporter. Physiol. Plant, 149, 329–339.
- Chen M, Mishra S, Heckathorn SA, Frantz JM, Krause C 2014: Proteomic analysis of Arabidopsis thaliana leaves in response to acute boron deficiency and toxicity reveals effects on photosynthesis, carbohydrate metabolism, and protein synthesis. J. Plant Physiol., 171, 235–242. doi: 10.1016/j.jplph.2013.07.008.
- Cheng C, Rerkasem B 1993: Effects of boron on pollen viability in wheat. In: Barrow NJ (ed) Plant Nutr. Genet. Eng. Field Pract. pp. 405–407. Springer, Netherlands.
- Chormova D, Messenger DJ, Fry SC 2014: Boron bridging of rhamnogalacturonan-II, monitored by gel electrophoresis, occurs during polysaccharide synthesis and secretion, but not post-secretion. Plant J., 77, 534–546. doi: 10.1111/tpj.12403
- Dannel F, Pfeffer H, Römheld V 2002: Update on boron in higher plants - uptake, primary translocation and compartmentation. Plant Biol., 4, 193–204. doi:10.1055/s-2002-25730
- Delmas F, Seveno M, Northey JGB, Hernould M, Lerouge P, McCourt P, Chevalier C 2008: The synthesis of the rhamnogalacturonan II component 3-deoxy-D-manno-2-octulosonic acid (Kdo) is required for pollen tube growth and elongation. J. Exp. Bot., 59, 2639–2647. doi:10.1093/jxb/ern118
- FAO, 2010: FAOSTAT Database. Food and Agriculture Organization of the United Nations, Rome, Available from: http://faostat.fao.org/.
- Gamuyao R, Chin JH, Pariasca-Tanaka J, Pesaresi P, Catausan S, Dalid C, Slamet-Loedin I, Tecson-Mendoza EM, Wissuwa M, Heuer S 2012: The protein kinase Pstol1 from traditional rice confers tolerance of phosphorus deficiency. Nature, 488, 535–539. doi:10.1038/nature11346
- Graham RD 1984: Breeding for nutritional characteristics in cereals. Adv. Plant Nutr., 1, 57–102.
- Hu H, Brown PH 1997: Absorption of boron by plant roots. Plant Soil, 193, 49–58. doi:10.1023/A:1004255707413
- Hu H, Brown PH, Labavitch JM 1996: Species variability in boron requirement is correlated with cell wall pectin. J. Exp. Bot., 47, 227–232. doi:10.1093/jxb/47.2.227
- Hu H, Penn SG, Lebrilla CB, Brown PH 1997: Isolation and characterization of soluble boron complexes in higher plants. The mechanism of phloem mobility of boron. Plant Physiol., 113, 649–655. doi:10.1104/pp.113.2.649
- Huang LB, Bell RW, Dell B 2001: Boron supply into wheat (Triticum aestivum L. cv. Wilgoyne) ears whilst still enclosed within leaf sheaths. J Exp. Bot., 52, 1731–1738. doi:10.1093/jexbot/52.361.1731
- Ishii T, Matsunaga T 1996: Isolation and characterization of a boron-rhamnogalacturonan-II complex from cell walls of sugar beet pulp. Carbohydr. Res., 284, 1–9. doi:10.1016/0008-6215(96)00010-9
- Ishii T, Matsunaga T, Hayashi N 2001: Formation of rhamnogalacturonan II-borate dimer in pectin determines cell wall thickness of pumpkin tissue. Plant Physiol., 126, 1698–1705. doi:10.1104/pp.126.4.1698
- Kobayashi M, Matoh T, Azuma J 1996: Two chains of rhamnogalacturonan II are cross-linked by borate-diol ester bonds in higher plant cell walls. Plant Physiol., 110, 1017–1020.
- Leaungthitikanchana S, Fujibe T, Tanaka M, Wang S, Sotta N, Takano J, Fujiwara T 2013: Differential expression of three BOR1 genes corresponding to different genomes in response to boron conditions in hexaploid wheat (Triticum aestivum L.). Plant Cell Physiol., 54, 1056–1063. doi:10.1093/pcp/pct059
- Liang C, Tian J, Liao H 2013: Proteomics dissection of plant responses to mineral nutrient deficiency. Proteomics, 13, 624–636. doi:10.1002/pmic.201200263
- Lordkaew S, Dell B, Jamjod S, Rerkasem B 2011: Boron deficiency in maize. Plant Soil, 342, 207–220. doi:10.1007/s11104-010-0685-7
- Lordkaew S, Konsaeng S, Jongjaidee J, Dell B, Rerkasem B, Jamjod S 2013: Variation in responses to boron in rice. Plant Soil, 363, 287–295. doi:10.1007/s11104-012-1323-3
- Lynch J 1995: Root architecture and plant productivity. Plant Physiol., 109, 7–13.
- Marschner P 2012: Function of mineral nutrients: micronutrients. In Marschner’s Mineral Nutrition of Higher Plants, Ed Marschner P, pp. 191–243. Academic Press, New York.
- Meyer M 2009: Rapeseed oil fuel - the crisis-proof home-made eco-fuel. Agrarforschung, 16, 262–267.
- Mishra S, Heckathorn S, Frantz J, Yu F, Gray J 2009: Effects of boron deficiency on geranium grown under different nonphotoinhibitory light levels. J. Am. Soc. Hortic. Sci., 134, 183–193.
- Miwa K, Fujiwara T 2010: Boron transport in plants: co-ordinated regulation of transporters. Ann. Bot., 105, 1103–1108. doi:10.1093/aob/mcq044
- Miwa K, Wakuta S, Takada S, Ide K, Takano J, Naito S, Omori H, Matsunaga T, Fujiwara T 2013: Roles of BOR2, a boron exporter, in crosslinking of rhamnogalacturonan II and root elongation under boron limitation in Arabidopsis thaliana. Plant Physiol., 163, 1699–1709. doi:10.1104/pp.113.225995
- Nakagawa Y, Hanaoka H, Kobayashi M, Miyoshi K, Miwa K, Fujiwara T 2007: Cell-type specificity of the expression of OsBOR1, a rice efflux boron transporter gene, is regulated in response to boron availability for efficient boron uptake and xylem loading. The Plant Cell Online, 19, 2624–2635. doi:10.1105/tpc.106.049015
- Noguchi K, Ishii T, Matsunaga T, Kakegawa K, Hayashi H, Fujiwara T 2003: Biochemical properties of the cell wall in the Arabidopsis mutant bor1-1 in relation to boron nutrition. J. Plant Nutr. Soil Sci, 166, 175–178. doi:10.1002/jpln.200390025
- O’Neill MA, Warrenfeltz D, Kates K, Pellerin P, Doco T, Darvill AG, Albersheim P 1996: Rhamnogalacturonan-II, a pectic polysaccharide in the walls of growing plant cell, forms a dimer that is covalently cross-linked by a borate ester In vitro conditions for the formation and hydrolysis of the dimer. J. Biol. Chem., 271, 22923–22930. doi:10.1074/jbc.271.37.22923
- O’Neill MA, Eberhard S, Albersheim P, Darvill AG 2001: Requirement of borate cross-linking of cell wall rhamnogalacturonan II for Arabidopsis growth. Science, 294, 846–849. doi:10.1126/science.1062319
- O’Neill MA, Ishii T, Albersheim P, Darvill AG 2004: Rhamnogalacturonan II: structure and function of a borate cross-linked cell wall pectic polysaccharide. Annu. Rev. Plant Biol., 55, 109–139. doi:10.1146/annurev.arplant.55.031903.141750
- Pan Y, Wang ZH, Yang L, Wang ZF, Shi L, Naran R, Azadi P, Xu FS 2012: Differences in cell wall components and allocation of boron to cell walls confer variations in sensitivities of Brassica napus cultivars to boron deficiency. Plant Soil, 354, 383–394. doi:10.1007/s11104-011-1074-6
- Perez-Castro R, Kasai K, Gainza-Cortes F, Ruiz-Lara S, Casaretto JA, Pena-Cortes H, Tapia J, Fujiwara T, Gonzalez E 2012: VvBOR1, the grapevine ortholog of AtBOR1, encodes an efflux boron transporter that is differentially expressed throughout reproductive development of Vitis vinifera L. Plant Cell Physiol., 53, 485–494. doi:10.1093/pcp/pcs001
- Rawson HM 1996: The developmental stage during which boron limitation causes sterility in wheat genotypes and the recovery of fertility. Aust. J. Plant Physiol., 23, 709–717. doi:10.1071/PP9960709
- Redondo-Nieto M, Maunoury N, Mergaert P, Kondorosi E, Bonilla I, Bolaños L 2012: Boron and calcium induce major changes in gene expression during legume nodule organogenesis. Does boron have a role in signalling?. New Phytologist, 195, 14–19. doi:10.1111/j.1469-8137.2012.04176.x
- Rerkasem B, Jamjod S 1997a: Boron deficiency induced male sterility in wheat (Triticum aestivum L.) and implications for plant breeding. Euphytica, 96, 257–262. doi:10.1023/A:1003093532561
- Rerkasem B, Jamjod S 1997b: Genotypic variation in plant response to low boron and implications for plant breeding. Plant soil, 193, 169–180.
- Rerkasem B, Jamjod S 2004: Boron deficiency in wheat: a review. Field Crops Res., 89, 173–186. doi:10.1016/j.fcr.2004.01.022
- Rerkasem B, Netsangtip R, Lordkaew S, Cheng C 1993: Grain set failure in boron deficient wheat. In Plant Nutr. Genet. Eng. Field Pract, Ed Barrow NJ pp. 401–404. Springer, Netherlands.
- Schon MK, Novacky A, Blevins DG 1990: Boron induces hyperpolarization of sunflower root cell membranes and increases membrane permeability to K+. Plant Physiol., 93, 566–571. doi:10.1104/pp.93.2.566
- Shi L, Wang Y., Nian FZ, Lu JW, Meng JL, Xu FS 2009: Inheritance of boron efficiency in oilseed rape. Pedosphere, 19, 403–408. doi:10.1016/S1002-0160(09)60132-6
- Shi L, Yang JP, Liu J, Li RY, Long Y, Xu FS, Meng JL 2012: Identification of quantitative trait loci associated with low boron stress that regulate root and shoot growth in Brassica napus seedlings. Mol. Breed., 30, 393–406. doi:10.1007/s11032-011-9629-z
- Shorrocks VM 1997: The occurrence and correction of boron deficiency. Plant Soil, 193, 121–148. doi:10.1023/A:1004216126069
- Stangoulis JCR, Grewal HS, Bell RW, Graham RD 2000a: Boron efficiency in oilseed rape: I. Genotypic variation demonstrated in field and pot grown Brassica napus L. and Brassica juncea L. Plant Soil, 225, 243–251. doi:10.1023/A:1026593528256
- Stangoulis JCR, Reid RJ, Brown PH, Graham RD 2001: Kinetic analysis of boron transport in Chara. Planta, 213, 142–146. doi:10.1007/s004250000484
- Stangoulis JCR, Webb MJ, Graham RD 2000b: Boron efficiency in oilseed rape: II. Development of a rapid lab-based screening technique. Plant Soil, 225, 253–261. doi:10.1023/A:1026595012326
- Sun JH, Shi L, Zhang CY, Xu FS 2012: Cloning and characterization of boron transporters in Brassica napus. Mol. Biol. Rep., 39, 1963–1973. doi:10.1007/s11033-011-0930-z
- Takano J, Miwa K, Fujiwara T 2008: Boron transport mechanisms: collaboration of channels and transporters. Trends Plant Sci., 13, 451–457. doi:10.1016/j.tplants.2008.05.007
- Takano J, Noguchi K, Yasumori M, Kobayashi M, Gajdos Z, Miwa K, Hayashi H, Yoneyama T, Fujiwara T 2002: Arabidopsis boron transporter for xylem loading. Nature, 420, 337–340. doi:10.1038/nature01139
- Takano J, Wada M, Ludewig U, Schaaf G, Wirén N, Fujiwara T 2006: The Arabidopsis major intrinsic protein NIP5;1 is essential for efficient boron uptake and plant development under boron limitation. The Plant Cell Online , 18, 1498–1509. doi:10.1105/tpc.106.041640
- Tanaka M, Fujiwara T 2008: Physiological roles and transport mechanisms of boron: perspectives from plants. Pflügers Archiv Integr. Eur. J. Physiol., 456, 671–677. doi:10.1007/s00424-007-0370-8
- Tanaka M, Wallace IS, Takano J, Roberts DM, Fujiwara T 2008: NIP6;1 is a boric acid channel for preferential transport of boron to growing shoot tissues in Arabidopsis. The Plant Cell Online, 20, 2860–2875. doi:10.1105/tpc.108.058628
- Trick M, Adamski NM, Mugford SG, Jiang CC, Febrer M, Uauy C 2012: Combining SNP discovery from next-generation sequencing data with bulked segregant analysis (BSA) to fine-map genes in polyploid wheat. BMC Plant Biol., 12, 14–30. doi:10.1186/1471-2229-12-14
- Wang X, Wang H, Wang J et al. 2011a: The genome of the mesopolyploid crop species Brassica rapa. Nat. Genet., 43, 1035–1039.
- Wang YH, Shi L, Cao XY, Xu FS 2007: Studies on plant boron nutrition and boron fertilization in China. In Advances in Plant and Animal Boron Nutrition, Eds Xu FS, Goldbach HE, Brown PH, Bell RW, Fujiwara T, Hunt CD, Goldberg S, Shi L, pp. 93–101. Springer press, Netherlands.
- Wang ZF, Wang ZH, Shi L, Wang LJ, Xu FS 2010: Proteomic alterations of Brassica napus root in response to boron deficiency. Plant Mol. Biol., 74, 265–278. doi:10.1007/s11103-010-9671-y
- Wang ZH, Wang ZF, Chen SS, Shi L, Xu FS 2011b: Proteomics reveals the adaptability mechanism of Brassica napus to short-term boron deprivation. Plant Soil, 347, 195–210. doi:10.1007/s11104-011-0838-3
- Wimmer MA, Lochnit G, Bassil E, Muhling KH, Goldbach HE 2009: Membrane-associated, boron-interacting proteins isolated by boronate affinity chromatography. Plant Cell Physiol., 50, 1292–1304. doi:10.1093/pcp/pcp073
- Xu FS, Wang YH 2007: Physiological and genetic bases of boron utilization efficiency in Brassica napus. In Advances in Plant and Animal Boron Nutrition, Eds Xu FS, Goldbach HE, Brown PH, Bell RW, Fujiwara T, Hunt CD, Goldberg S, Shi L, pp. 205–211. Springer press, Netherlands.
- Xu FS, Wang YH, Meng JL 2001: Mapping boron efficiency gene(s) in Brassica napus using RFLP and AFLP markers. Plant Breed., 120, 319–324. doi:10.1046/j.1439-0523.2001.00583.x
- Xu FS, Wang YH, Ying WH, Meng JL 2002a: Inheritance of boron nutrition efficiency in Brassica napus. J. Plant Nutr., 25, 901–912. doi:10.1081/PLN-120002968
- Xu FS, Yang YH, Wang YH, Wu LS 2002b: Boron uptake and retranslocation in cultivars of Brassica napus differing in boron efficiency. In Boron in Plant and Animal Nutrition, Eds Goldbach HE, Brown PH, Rerkasem B, Thellier M, Wimmer MA, Bell RW, pp. 127–135. Kluwer Academic/Plenum Publisher press, New York.
- Xue JM, Lin MS, Bell RW, Graham RD, Yang XE, Yang YA 1998: Differential response of oilseed rape (Brassica napus L.) cultivars to low boron supply. Plant Soil, 204, 155–163. doi:10.1023/A:1004326217479
- Yan JB, Yang XH, Shah T, Sánchez-Villeda H, Li JS, Warburton M, Zhou Y, Crouch JH, Xu YB 2010: High-throughput SNP genotyping with the GoldenGate assay in maize. Mol. Breed., 25, 441–451. doi:10.1007/s11032-009-9343-2
- Yang L, Zhang Q, Dou JN, Li L, Guo LF, Shi L, Xu FS 2013: Characteristics of root boron nutrition confer high boron efficiency in Brassica napus cultivars. Plant Soil, 371, 95–104. doi:10.1007/s11104-013-1669-1
- Yang YH, Wang LJ, Yu M, Du CW, Wang YH, Wu LS 2002: Effect of B on cell wall regeneration from protoplasts of B-efficient and B-inefficient rape (Brassica napus L.) cultivars. In Boron in Plant and Animal Nutrition, Eds Goldbach HE, Brown PH, Rerkasem B, Thellier M, Wimmer MA, Bell RW, pp. 289–298. Kluwer Academic/Plenum Publisher press, New York.
- Zhao H, Shi L, Duan XL, Xu FS, Wang YH, Meng JL 2008: Mapping and validation of chromosome regions conferring a new boron-efficient locus in Brassica napus. Mol. Breed., 22, 495–506. doi:10.1007/s11032-008-9193-3
- Zhao ZK, Wu LK, Nian FZ, Ding GD, Shi TX, Zhang DD, Shi L, Xu FS, Meng JL 2012: Dissecting quantitative trait loci for boron efficiency across multiple environments in Brassica napus. PLoS ONE, 7, e45215. doi:10.1371/journal.pone.0045215
- Zou J, Lu JW, Liao ZW, Gong XM, Wang H, Zhou YG, Zhou H 2008: Study on response of rapeseed to boron application and critical level of soil available B in Hubei province. Sci. Agric. Sin., 41, 752–759. (in Chinese).