Abstract
The effects of nitrogen (N) and sulfur (S) deposition on methane (CH4) and nitrous oxide (N2O) emissions under low (10 cm below soil surface) and high (at soil surface) water tables were investigated in the laboratory. Undisturbed soil columns from the alpine peatland of the Tibetan Plateau were analyzed. CH4 emission was higher and N2O emission was lower at the high water table than those at the low water table regardless of nutrient application. Addition of N (NH4NO3 (ammonium nitrate), 5 g N m−2) decreased CH4 emission up to 57% and 50% at low and high water tables, respectively, but correspondingly increased N2O emission by 2.5 and 10.4 times. Addition of S (Na2SO4 (sodium sulfate), 2.5 g S m−2) decreased CH4 and N2O emission by 64% and 79% at the low water table, respectively, but had a slightly positive effect at the high water table. These results indicated that the responses of CH4 and N2O emissions to the S deposition depend on the water table condition in the high-altitude peatland.
INTRODUCTION
Peatlands naturally contain large amounts of carbon (C) and nitrogen (N) within their soils, and currently serve as sources for methane (CH4) and nitrous oxide (N2O) (Regina et al. Citation1999; Huttunen et al. Citation2003). CH4 and N2O, as two important greenhouse gases, are approximately 25 and 300 times more effective in global warming than carbon dioxide (CO2), respectively (Hou et al. Citation2013). CH4 is produced by methanogenesis, whereas N2O is released mainly from nitrification and denitrification (Aerts and Ludwig Citation1997; Nykänen et al. Citation2002). These soil microbiological processes depend remarkably on the soil water conditions and nutrient status (Aerts and Ludwig Citation1997). Peatlands, similar to other ecosystems, undergo climate changes and nutrient [especially N and sulfur (S)] loading because of atmospheric deposition and other anthropogenic activities (Keller et al. Citation2006). These changes may alter the soil water table and nutrient status (i.e. N and S) and further affect the emission rates of CH4 and N2O from peatland soils. It is therefore urgent to assess the effect of water table conditions, as well as N and S inputs, on greenhouse gas flux in the peatland soils.
Several studies have recently examined the effects of water table on the CH4 gas fluxes in peatland soils (Aerts and Ludwig Citation1997; Dinsmore et al. Citation2009; Chen et al. Citation2011b; Hou et al. Citation2013). These studies have found that lowering the water table decreases CH4 emission. A few studies on the effect of water table depth on peatland soil N2O emissions have been conducted (Aerts and Ludwig Citation1997; Regina et al. Citation1999; Hou et al. Citation2013), in which the lowering of the water table resulted in a net increase in N2O fluxes. However, the effects of N and S input, especially for S, on CH4 and N2O emissions of peatland soils are rare and limited to low-elevation peatlands of the boreal zone (Aerts and Ludwig Citation1997; Granberg et al. Citation2001; Nykänen et al. Citation2002; Melanie and Bridgham Citation2003). Uncertain conclusions have been raised on the CH4 fluxes as affected by N and S amendment (Granberg et al. Citation2001). Studies on the effect of N addition on N2O emission generally show that N stimulates N2O emission from the boreal peatland soil (Nykänen et al. Citation2002; Zhang et al. Citation2007). Although S deposition may alter N2O emission in soils (Sanderson et al. Citation2006), little is known about the impact of S deposition on N2O emission in peatland. Until now the effects of water table on high-altitude peatlands have received less attention, and no information is available about the impact of N or S on the greenhouse gas emissions within these ecosystems.
The Tibetan Plateau is the highest [average 4000 m above sea level (a.s.l.)] plateau in the world, an area that contains the world’s largest extent of high-altitude peatlands. These alpine peatlands contain a large amount of soil organic C and play an important role in the greenhouse gas budget of peatlands in the world (Wang et al. Citation2002). The peatland areas of the Tibetan Plateau are currently receiving elevated rates of atmospheric N and S deposition (Wang et al. Citation2004; Liu et al. Citation2011). Although several studies have investigated the greenhouse gases in the alpine peatlands of the Tibetan Plateau, the majority of these studies have only analyzed the effects of temporal-spatial variations and vegetation on CH4 and N2O fluxes (Chen et al. Citation2011a, Citation2011b). Investigations on the impact of N and S applications and their interactions with different water tables in the alpine peatland are insufficient.
This study was designed to measure the role of N and S deposition on the CH4 and N2O emissions under various water table conditions in the alpine peatland soil on the Tibetan Plateau. We determined the effects of increased N and S addition in alpine peatland soil on CH4 and N2O emissions. We also evaluated whether the observed variations depend on the water table condition.
MATERIALS AND METHODS
Study sites
The study was conducted at Hongyuan County (33°54′N, 102°36′E, at 3500 m a.s.l.), located on the eastern Tibetan Plateau, Southwest China. This area is characterized by a harsh continental climate. The mean annual temperature is 1.1°C. The highest monthly mean temperature is 10.9°C in July and the lowest is –10.3°C in January. The mean annual precipitation is 753 mm, with approximately 86% received from May until September. The vegetation of the study site is dominated by Carex muliensis (Hand.-Mazz.), accompanied by Caltha scaposa (Hook. F. et Thoms), Chamaesium paradoxum (H. Wolff) and Sanguisorba filiformis ((Hook. f.) Hand.-Mazz.). The soil of the study site was classified as peat. The peat layer of the investigated site is more than 2 m deep. The soil characteristics of the study site are shown in .
Table 1 Soil characteristics in the study site
Experimental setup
Forty undisturbed soil columns (11 cm diameter, 30 cm depth) were collected by inserting polyvinyl chloride (PVC) tubes into the peatland in August 2012. Vegetation and litter layer were removed before inserting the tubes. All columns were cooled with freezer blocks and returned to the laboratory in insulated boxes. The soil columns were then randomly divided into two groups, and each group consisted of 20 columns. The water tables in the soil columns in one group were adjusted with distilled water to 10 cm below the soil surface and to the soil surface for the other group. The columns were then pre-incubated in a dark climate room at 15°C for 5 days before the nutrients treatments were applied. Incubation temperature was designated as the soil temperature during soil column sampling in the field. After the pre-incubation period, independent, as well as combined, N and S additions were tested and compared with untreated control columns within each group. The soil columns were supplied once with 10 mL of nutrient solution. The nutrient supply consisted of 1.7 mmol NH4NO3 (ammonium nitrate) or 0.7 mmol Na2SO4 (sodium sulfate), which is equivalent to 5.0 g N m−2 and 2.5 g S m−2, respectively. The amount of N treatment corresponds closely to the amounts supplied by local farmers to grasslands in the study area. The amount of S treatment is close to the atmospheric S deposition in this region (Liu et al. Citation2011). Control columns were supplied with 10 mL of distilled water. Then, the water tables were adjusted with distilled water to 10 cm below the soil surface and to the soil surface in soil columns for the two groups to further compensate for the evaporative water loss from the column during the pre-incubation period. The water tables were then maintained until the end of the experiment by adding distilled water every 2–3 d. To minimize the effects of the water fluctuation caused by water addition on gas emissions, the distilled water was added at least 1 d prior to gas sampling. Each treatment was replicated five times. All soil columns were incubated in a dark climate room at 15°C during the period of this investigation.
Measurements
The gases for CH4 and N2O were determined at day 2, 4, 8, 12, 16, 20, 24, 28, 32, 36 and 40 after nutrient application. For measurements, a PVC cap fitted with a septum sampling valve was securing to the top of each column for 1 h. A needle was inserted into the septum while fitting the cap to minimize pressure disturbance. Gas samples (10 mL) were taken from the columns after 20, 40 and 60 min using a polypropylene syringe. All gas samples were collected between 9:00 AM and 11:00 AM. The concentrations of gases within the samples were analyzed using a gas chromatograph (Hewlett Packard 5890N) within 24 h after the gas sampling. The gas chromatograph was equipped with a flame ionization detector for CH4 analysis and an electron capture detector for N2O analysis.
Calculation and statistical analysis
The fluxes of CH4 and N2O were calculated from the linear changes in the gas concentration of the headspace volume for each soil column (Chen et al. 2011). Cumulative CH4 and N2O emissions from each column were calculated by linear interpolation between sampling dates (Ni et al. Citation2012).
Statistical analyses were performed using SPSS 16.0 (SPSS Inc., Chicago). Data were tested for normality and log-transformed before analysis of variance (ANOVA) was conducted. One-way ANOVA was used to determine the effect of nutrients on the CH4 and N2O emission rates and on cumulative amounts. Differences between the two soil table conditions with the same nutrient addition were tested using Mann-Whitney U tests. Significance was accepted at P < 0.05.
RESULTS
The CH4 emission rates from this alpine peatland soil at low water table were generally lower than those at the high water table during the investigation days ( and ). Addition of N had a significant negative effect on the CH4 fluxes under the two water table conditions for 40 d of incubation. Addition of S decreased the CH4 flux in the low water table, but tended to increase CH4 flux in the high water table.
Figure 1 The methane (CH4) fluxes in control (C), nitrogen (N), sulfur (S) and both nitrogen and sulfur (NS) additions under (a) low water table and (b) high water table during the investigation period. Values are means and standard errors for five replicate samples.
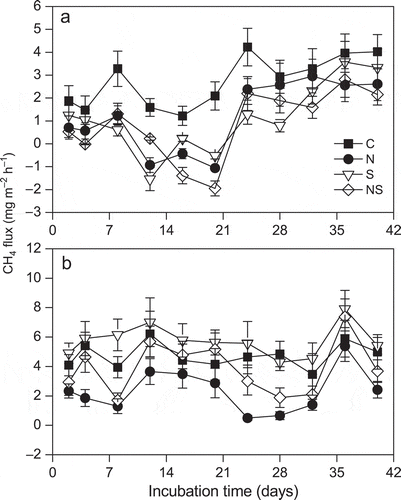
The N2O emission rates of the control soil at low water table were higher than those at high water table over the entire incubation period ( and ). Addition of N had a significant positive impact on N2O fluxes regardless of water levels and resulted in a large increase in the N2O emission rate on the first sampling day ( and ). Addition of S significantly inhibited the N2O fluxes at the low water table during the investigation period ().
Figure 2 The nitrous oxide (N2O) fluxes in control (C), nitrogen (N), sulfur (S) and both nitrogen and sulfur (NS) additions under (a) low water table and (b) high water table during the investigation period. The panel situated in the upper right-hand corner of the figure is to show N2O fluxes from day 8 to day 40 more clearly. Values are means and standard errors for five replicate samples.
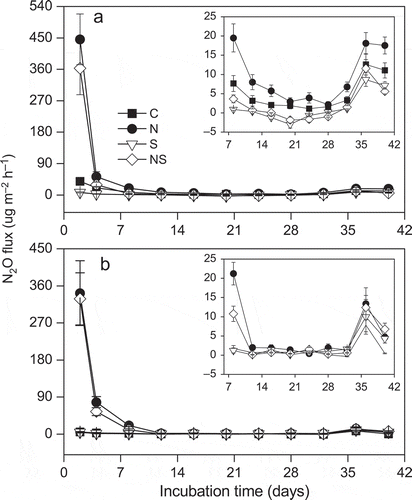
The cumulative emissions of CH4 for 40 d in soils with N addition decreased by 57% and 50% in the low and high water tables, respectively, compared with those of the control soils ( and ). Addition of S reduced cumulative CH4 emissions in the low water table by more than 60% compared with the control soil, but had a slightly positive impact in the high water table ( and ).
Figure 3 The cumulative methane (CH4) and nitrous oxide (N2O) emissions in control (C), nitrogen (N), sulfur (S) and both nitrogen and sulfur (NS) additions under (a, c) low water table and (b, d) high water table during the investigation period. Values are means and standard errors for five replicate samples. Different letters indicate significant differences at P < 0.05 using the least significant difference (LSD) test.
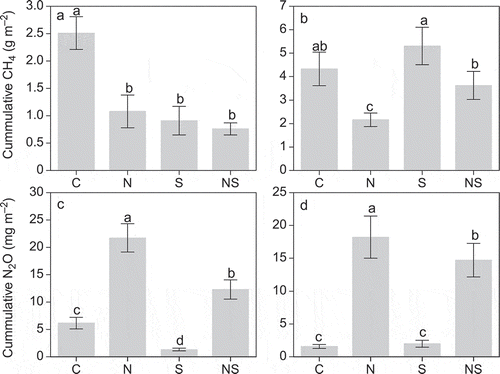
The N2O cumulative emissions during the investigation period were 2.5 and 10.4 times higher in soils with N addition than those in the control at low and high water tables, respectively ( and ). Compared with the control, S addition decreased N2O cumulative emissions up to 79% in the low water tables, but tended to increase N2O cumulative emissions in the high water table ( and ).
DISCUSSION
CH4 emission
The CH4 emission from the peatland soil was found to be higher in the high water table than in the low water table in this study, which was similar to the conclusion from the previous studies (Aerts and Ludwig Citation1997; Dinsmore et al. Citation2009; Yamamoto et al. Citation2011). The amount of CH4 released from peatland soil to the atmosphere is the difference between the production and oxidation of CH4 in soil (Nykänen et al. Citation2002). Increased water table level may result in decreased oxygen availability in the upper soil layer, which would limit methane oxidation and lead to an increase in the soil CH4 emission (Aerts and Ludwig Citation1997; Kotelnikova Citation2002).
N inputs can affect the activity of both CH4-producing and CH4-oxidizing microbes (Nykänen et al. Citation2002). Nitrate (NO3–) can decrease methanogenesis because of microbes that use nitrate as electron acceptors while the microbes are oxidizing organic substrates, and then methanogens cannot compete for their substrates with nitrate reducers (Chowdhury and Dick 2013). Ammonium (NH4+) compounds generally reduce the activity of the CH4-oxidizing bacteria and increase CH4 release in soil (Nykänen et al. Citation2002). However, Rigler and Zechmeister-Boltenstern (Citation1999) and Bodelier et al. (Citation2000) reported that the both NO3– and NH4+ can increase methane oxidation and decrease CH4 release in N-limited conditions. Our study showed that N addition inhibits the CH4 production at the two water tables. Considering that the amounts of NO3– and NH4+ added into the soils are almost equal in our study, the negative effects of N on CH4 production cannot be attributed completely to the decrease in methanogenesis by adding NO3–. Rigler and Zechmeister-Boltenstern (Citation1999) and Bodelier et al. (Citation2000) reported that NO3– and NH4+ can increase methane oxidation and decrease CH4 release in nitrogen-limited conditions. This result could explain the soil CH4 responses to NH4NO3 addition in this alpine peatland where low N is available in the soils because low air temperature causes low rates of organic matter decomposition.
The sulfate ion (SO42–) addition to the peatland soil with the low water table reduced CH4 emission in this study. This result is comparable to those of others who found a decrease in CH4 production caused by SO42– in peat soil under aerobic conditions (Yavitt et al. Citation1987; Dise and Verry Citation2001; Granberg et al. Citation2001). The major effect of SO42– on CH4 production is exerted through its effects on the competition for substrate (i.e. electron donors, hydrogen and acetate) between sulfate-reducing bacteria and methanogens (Gauci et al. Citation2004; Eriksson et al. Citation2010). When SO42– is applied, the sulfate-reducing bacteria in peat, stimulated by the increasing SO42– availability, consumed more substrate and inhibited methanogens. However, the inhibition of CH4 production by SO42– addition in wetland soil could be reversed by increasing acetate or hydrogen (Winfrey and Zeikus Citation1977). Yavitt et al. (Citation1987) also reported that SO42– addition to the deep peat led to an increased production of these substrates by starting a cascade of anaerobic reactions. The increased production of substrates caused the small increase in CH4 emission with SO42– addition, which was measured in the high water table in this study. These results suggested that the water table plays an important role in regulating the effect of S deposition on CH4 in the high-altitude peatland.
N2O emission
N2O is produced mainly during both nitrification and denitrification in soil (Aerts and Ludwig Citation1997; Nykänen et al. Citation2002). Nitrification requires aerobic environments, whereas denitrification requires anaerobic conditions (Aerts and Ludwig Citation1997). In the current study, higher N2O emission was measured at a low water level, similar to previous studies that were performed in both field and laboratory experiments (Aerts and Ludwig Citation1997; Zhu et al. Citation2008; Hou et al. Citation2013). The high N2O emission of the low water table is due to the following: (1) the low water table increased aerobic condition in the upper soil, which enhances nitrification of ammonium (Aerts and Ludwig Citation1997), and (2) the N2O would be reduced to N2 by denitrification at high water table because of low oxygen availability (Regina et al. Citation1999).
Addition of N generally results in the enhancement of N2O fluxes in peatland soils because of the increase in the substrate during nitrification and denitrifcation after NH4NO3 application (Nykänen et al. Citation2002; Zhang et al. Citation2007). A similar result on the effects of N addition on N2O flux was also observed in the present study.
The SO42– addition inhibited N2O production in the low water table, but stimulated N2O emission in the high water table in the present study. To the best of our knowledge, no reports are available on the effects of SO42– on N2O emission in peatland soils. Several studies have investigated the effect of S on N2O emission in aquatic sediments (Brunet and Garcia-Gil Citation1996), agricultural soils (Hasegawa et al. Citation2000) and subtropical forest soils (Cai et al. Citation2012). These studies showed that the effects of S addition on N2O emission in soils varied depending on soil properties. N2O production in SO42–-treated soil is related to sulfur denitrification via sulfate reduction or heterotrophic denitrification (Yamashita et al. Citation2011). Under aerobic condition, sulfate-reducing bacteria simulated by adding SO42– consume substrate (hydrogen and acetate) and inhibit sulfur denitrification (Winfrey and Zeikus Citation1977; Zhu et al. Citation2005). Under anaerobic conditions, the increased substrate simulated sulfur denitrification to reduce nitrate to nitrogen gas (Yavitt et al. Citation1987), indicating that the magnitude of the response of the N2O production in the alpine peatland soil to the S addition is also dependant on the water table level.
Overall, this study demonstrated that N addition inhibits CH4 production and increases N2O emission regardless of water table conditions in the alpine peatland, but the effects of SO42– on CH4 and N2O emissions vary depending on the water table levels. Given the importance of alpine peatland to the global emission of CH4 and N2O, a better understanding of the effects of the N and S inputs with different water tables on the these gases at the ecosystem level should be a strong priority for future studies.
ACKNOWLEDGMENTS
This research was supported by the National Basic Research Program (973) of China (No. 2012CB417101), and the National Science Foundation (No. 40801089 & 41271276). We gratefully acknowledge the assistance of Changbing Zhang and Ping Li during the field work. We greatly appreciate the constructive comments of the editors and two anonymous reviewers on an earlier version of the manuscript.
REFERENCES
- Aerts R, Ludwig F 1997: Water-table changes and nutritional status affect trace gas emissions from laboratory columns of peatland soils. Soil Biol. Biochem., 29, 1691–1698. doi:10.1016/S0038-0717(97)00074-6
- Bodelier PLE, Roslev P, Henckel T, Frenzel P 2000: Stimulation by ammonium-based fertilizers of methane oxidation in soil around rice roots. Nature, 403, 421–424. doi:10.1038/35000193
- Brunet RC, Garcia-Gil LJ 1996: Sulfide-induced dissimilatory nitrate reduction to ammonia in anaerobic freshwater sediments. FEMS Microbiol. Ecol., 21, 131–138. doi:10.1111/j.1574-6941.1996.tb00340.x
- Cai ZC, Zhang JB, Zhu TB, Cheng Y 2012: Stimulation of NO and N2O emissions from soils by SO2 deposition. Glob. Change Biol, 18, 2280–2291. doi:10.1111/j.1365-2486.2012.02688.x
- Chen H, Wang M, Wu N, Wang YF, Zhu D, Gao YH, Peng CH 2011a: Nitrous oxide fluxes from the littoral zone of a lake on the Qinghai-Tibetan Plateau. Environ. Monit. Assess., 182, 545–553. doi:10.1007/s10661-011-1896-y
- Chen H, Wu N, Wang YF, Gao YH, Peng C., Tian JP 2011b: Methane fluxes from Alpine wetlands of Zoige plateau in relation to water regime and vegetation under two scales. Water Air Soil Poll., 217, 173–183. doi:10.1007/s11270-010-0577-8
- Dinsmore KJ, Skiba UM, Billett MF, Rees RM 2009: Effect of water table on greenhouse gas emissions from peatland mesocosms. Plant Soil, 318, 229–242. doi:10.1007/s11104-008-9832-9
- Dise NB, Verry E 2001: Suppression of peatland methane emission by cumulastive sulfate deposition in simulated acid rain. Biogeochemistry, 53, 143–160. doi:10.1023/A:1010774610050
- Eriksson T, Öquist MG, Nilsson MB 2010: Effects of decadal deposition of nitrogen and sulfur, and increased temperature, on methane emssions from a boreal peatland. J. Geophys. Res., 115, G04036. doi:10.1029/2010JG001285
- Gauci V, Matthews E, Dise N, Walter B, Koch D, Granberg G, Vile M 2004: Sulfur pollution suppression of the wetland methane source in the 20th and 21st centuries. Proc. Nat. Acad. Sci., 101, 12583–12587. doi:10.1073/pnas.0404412101
- Granberg G, Sundh I, Svensson BH, Nilsson M 2001: Effects of temperature, and nitrogen and sulfur deposition, on methane emission from a boreal mire. Ecology, 82, 1982–1998. doi:10.1890/0012-9658(2001)082[1982:EOTANA]2.0.CO;2
- Hasegawa K, Hanaki K, Matsuo T, Hidaka S 2000: Nitrous oxide from the agricultural water system contaminated with high nitrogen. Chemosphere - Glob Change Sci., 2, 335–345. doi:10.1016/S1465-9972(00)00009-X
- Hou CC, Song CC, Li YC, Wang JY, Song YY, Wang XW 2013: Effects of water table changes on soil CO2, CH4 and N2O fluxes during the growing season in freshwater marsh of Northeast China. Environ. Earth. Sci., 69, 1963–1971. doi:10.1007/s12665-012-2031-2
- Huttunen JT, Nykänen H, Turunen J, Martikainen PJ 2003: Methane emissions from natural peatlands in the northern boreal zone in Finland, Fennoscandia. Atmos. Environ., 37, 147–151. doi:10.1016/S1352-2310(02)00771-9
- Keller JK, Bauers AK, Bridgham SD, Kellogg LE, Iverson CM 2006: Nutrient control of microbial carbon cycling along an ombrotrophic-minerotrophic peatland gradient. J. Geophys. Res., 111, G03006. doi:10.1029/2005JG000152
- Kotelnikova S 2002: Microbial production and oxidation of methane in deep subsurface. Earth-Sci. Rev., 58, 367–395. doi:10.1016/S0012-8252(01)00082-4
- Liu XJ, Duan L, Mo JM, Du EZ, Shen JL, Lu XK, Zhang Y, Zhou XB, He C, Zhang FS 2011: Nitrogen deposition and its ecological impact in China: An overview. Environ. Poll., 159, 2251–2264. doi:10.1016/j.envpol.2010.08.002
- Melanie A, Bridgham S 2003: Atmospheric sulfur deposition alters pathways of gaseous carbon production in peatlands. Global Biogeochem. Cy, 1(7), 1058–1064.
- Ni K, Ding WX, Cai ZC, Wang YF, Zhang XL, Zhou BK 2012: Soil carbon dioxide emission from intensively cultivated black soil in Northeast China: Nitrogen fertilization effect. J. Soils Sediments, 12, 1007–1018. doi:10.1007/s11368-012-0529-6
- Nykänen H, Vasander H, Huttunen JT, Martikainen P 2002: Effect of experimental nitrogen load on methane and nitrous oxide fluxes on ombrotrophic boreal peatland. Plant Soil, 242, 147–155. doi:10.1023/A:1019658428402
- Regina K, Silvola J, Martikainen PJ 1999: Short-term effects of changing water table on N2O fluxes from peat monoliths from natural and drained boreal peatlands. Glob. Change Biol., 5, 183–189. doi:10.1046/j.1365-2486.1999.00217.x
- Rigler E, Zechmeister-Boltenstern S 1999: Oxidation of ethylene and methane in forest soils – effect of CO2 and mineral nitrogen. Geoderma, 90, 147–159. doi:10.1016/S0016-7061(98)00099-8
- Sanderson MG, Collins WJ, Johnson CE, Derwent RG 2006: Present and future acid deposition to ecosystems: The effect of climate change. Atmos. Environ., 40, 1275–1283. doi:10.1016/j.atmosenv.2005.10.031
- Wang GX, Qian J, Cheng GD, Lai YM 2002: Soil organic carbon pool of grassland soils on the Qinghai-Tibetan Plateau and its global implication. Sci. Total Environ., 291, 207–217. doi:10.1016/S0048-9697(01)01100-7
- Wang TJ, Hu ZY, Xie M, Zhang Y, Xu CK, Chao ZH 2004: Atmospheric sulfur deposition onto different ecosystems over China. Environ. Geochem. Health, 26, 169–177. doi:10.1023/B:EGAH.0000039579.68264.3d
- Winfrey MR, Zeikus JG 1977: Effect of sulfate on carbon and electron flow during microbial methanogenesis in freshwater sediments. Appl. Environ. Microbiol., 33, 272–281.
- Yamamoto A, Hirota M, Suzuki S, Zhang PC, Mariko S 2011: Surrounding pressure controlled by water table alters CO2 and CH4 fluxes in the littoral zone of a brackish-water lake. Appl. Soil Ecol., 47, 160–166. doi:10.1016/j.apsoil.2010.12.010
- Yamashita T, Yamamoto-Ikemoto R, Zhu JQ 2011: Sulfate-reducing bacteria in a denitrification reactor packed with wood as a carbon source. Biores. Technol., 102, 2235–2241. doi:10.1016/j.biortech.2010.10.015
- Yavitt JB, Lang GE, Wieder RK 1987: Control of carbon mineralization to CH4 and CO2 in anaerobic, Sphagnum-derived peat from Big Run Bog, West Virginia. Biogeochemistry, 4, 141–157. doi:10.1007/BF02180152
- Zhang LH, Song CC, Zheng XH, Wang DX, Wang YY 2007: Effects of nitrogen on the ecosystem respiration, CH4 and N2O emissions to the atmosphere from the freshwater marshes in northeast China. Environ. Geol., 52, 529–539. doi:10.1007/s00254-006-0485-9
- Zhu RB, Liu YS, Ma J, Xu H, Sun LG 2008: Nitrous oxide flux to the atmosphere from two coastal tundra wetlands in eastern Antarctica. Atmos. Environ., 42, 2437–2447. doi:10.1016/j.atmosenv.2007.12.016
- Zhu RB, Sun LG, Zhao SP, Xie ZQ, Liu XD, Yin XB 2005: Preliminary studies on nitrous oxide emissions from the ornithogenic soils on Xi-sha atoll, South China Sea. J. Environ. Sci., 17, 551–556.