Abstract
Climate warming exhibits strong diurnal variations, with higher warming rates being observed at nighttime, which significantly affects rice (Oryza sativa L.) growth and grain yield. The objective of this study was to determine the effects of asymmetric warming (all-day warming, AW; daytime warming from 07:00 to 19:00, DW; nighttime warming from 19:00 to 07:00, NW, and a control, CK) on rice nitrogen (N) dynamics and productivity. Two rice bucket warming experiments were performed in Nanjing in Jiangsu Province, China, using the free air temperature increase (FATI) technique. The daily mean temperatures in the rice canopy in the AW, DW and NW plots were 2.0, 1.1 and 1.3ºC higher than those in the rice canopy in the CK plots, respectively. The results indicated that the total N accumulation of rice was 8.27–40.53% higher in the warming treatment than in the control during the jointing, anthesis and maturity stages. However, there was no significant difference detected among the three warming treatments. The warming treatment substantially decreased N translocation efficiency, leading to the retention of more N in the plant stems during grain filling. The warming treatment also decreased the N harvest index, N utilization efficiency based on grain yield and N utilization efficiencies based on biomass in both growing seasons. The warming treatment significantly increased the aboveground biomass (9.26–16.18%) in the jointing stage but decreased it (2.75–9.63%) in the maturity stage. Although DW increased the carbon (C) gain by photosynthesis and NW increased the C loss by night respiration, the daytime higher-temperature treatment affected rice photosynthesis and reduced its photosynthetic rate and product. This effect may be one of the primary reasons for the insignificant difference in the aboveground biomass between the DW and NW treatments. In the AW, DW and NW plots, the grain yield was reduced by an average of 10.07, 5.05 and 7.89%, respectively, across both years. The effective panicles and grains per spike tended to decrease in the warmed plots, whereas irregular changes in the 1000-grain weight were observed. Our results suggest that under the anticipated climate warming, rice productivity would further decline in the Yangtze River Basin.
INTRODUCTION
Climate warming is one of the most significant environmental problems in the modern world. The global average temperature has increased by 0.56 to 0.92°C over the past century, and it is now predicted that the global temperature will be 1.4 to 5.8°C warmer by the year 2100 (IPCC Citation2007). Indeed, air temperatures have been observed to increase rapidly, a process that shows distinct asymmetry: the increase of the minimum temperature at night is nearly twice that of the maximum temperature in the daytime (Harvey Citation1995; Easterling et al. Citation1997). Such unprecedented changes in the differential increase of the daytime/nighttime temperature could have important effects on crop production (Lobell and Asner Citation2003; Tao et al. Citation2008; Fang et al. Citation2012).
Rice (Oryza sativa L.) is the major grain produced in China, accounting for approximately 40% of the total grain output. However, rice production is markedly affected by elevated air temperature. Safe rice production has aroused an extremely large amount of interest from the Chinese government and researchers (Cheng et al. Citation2008; Dong et al. Citation2011). To date, many studies have been conducted to examine the effects of elevated air temperature on rice growth and yield (Lobell et al. Citation2008; Cheng et al. Citation2010). For example, Peng et al. (Citation2004) showed that the rice yield decreased by 10% under a 1°C increase in the daily minimum temperature. Sheehy et al. (Citation2006) found that the rice yield declined by 13.70% under a 1°C elevation of the daily minimum temperature by analyzing a crop model. Dong et al. (Citation2011) also observed that nocturnal warming significantly decreased rice yield and aboveground biomass. These results show that warming treatments shorten the rice growth stage, decrease photosynthetic capacity and reduce grain yield. On the other hand, elevated air temperature could potentially enhance nitrogen (N) soil mineralization and thereby alter the N uptake of plant roots (Patil et al. Citation2010). Furthermore, internal nutrient utilization would likely be affected by the difference in physiological processes as a result of elevated temperature (Prieto et al. Citation2009), which exerts a profound impact on plant production (Li et al. 2011). Few studies have reported on the effects of elevated air temperature on N uptake in crops (Jonassona et al. Citation2004; Yang et al. Citation2010). Hence, experimental data on the effect of asymmetric warming on rice N dynamics and productivity are crucial for developing agricultural adaptation measures to cope with the potential impacts of climate warming on the agro-ecosystem on a regional and global scale.
N uptake is an important plant process that determines photosynthetic capacity and plant productivity. Numerous studies, mostly conducted in natural ecosystems without N fertilization, have shown that warming treatments can either increase or decrease the N accumulation of the studied plants. For example, An et al. (Citation2005) observed that a 4-year experimental warming period increased grass N accumulation by 5–20% due to the increases in the aboveground biomass exceeding the decreases in the leaf N concentration. However, other studies, conducted in growth chambers, have demonstrated that high temperatures decrease both aboveground biomass and leaf N concentration (Xu and Zhou Citation2005), leading to a reduction in plant N accumulation. Recently, studies conducted with rice crops in controlled-environment chambers have indicated that high nighttime temperatures have no impact on whole-plant N concentration but significantly increase N absorption (Cheng et al. Citation2010). However, no study has examined the effects of asymmetric warming on N accumulation and translocation in rice.
Artificial simulation experiments have recently been conducted in plant growth chambers or greenhouses, but the temperature increases generated by these devices are set in a manner that is inconsistent with actual climate warming. It is notably difficult to simulate the features of real climate warming (Klein et al. Citation2005; Cheng et al. Citation2009). Thus, a field experiment was performed in Nanjing, China, to investigate the effects of asymmetric warming on the rice biomass and grain yield and the nutrient utilization dynamics of the Huaidao5 rice cultivar using a free air temperature increase (FATI) apparatus. Our principal objectives were (1) to determine the effects of asymmetric warming on rice grain yield and yield components and (2) to characterize the N dynamics in response to asymmetric warming.
MATERIALS AND METHODS
Experimental design
Two experiments (Experiment 1 in 2011 and Experiment 2 in 2012) were conducted at the agro-meteorological experimental station (32°07’N, 118°50’E) of Nanjing University of Information Science and Technology in Jiangsu Province, China, during the rice-growing season from mid-May to October. This region has a warm, semi-humid monsoon climate. The average yearly precipitation is 1100 mm. The average air temperature from 2000 to 2010 was 16.6°C, which is 1.4 and 0.7°C warmer than that in the 1980s and 1990s, respectively. The average sunshine period is over 1900 h, and the frost-free period is 237 d.
The rice cultivar used in this study was Huaidao5 (conventional Japonica rice), which is widely cultivated in Nanjing, China. In both study years, sowing was carried out on May 18. One seedling was transplanted in each plastic bucket (25 cm inside diameter, 28 cm height) filled with 8.0 kg Hapli-stagnic gleysol on June 20. The soil was collected from the plow layer (~15 cm of the top layer) of a rice field in Pukou, Nanjing, China, that contained 9.28 g kg−1 organic carbon (C), 1.06 g kg−1 total N, 6.89 mg kg−1 available phosphorus (P) and 125 mg kg−1 exchangeable potassium (K). A total of 0.68 g N per bucket [CO(NH2)2] carbamide was split-applied: 50% applied at transplanting, 25% at jointing and 25% at booting. P and K were applied after planting as calcium superphosphate and potassium chloride at a rate of 0.15 g P per bucket and 0.37 g K per bucket, respectively. Hand weeding was conducted before sowing to control weeds. Pesticides (imidacloprid) and fungicides (tebuconazole) were sprayed to control pests and diseases as needed.
Experimental design and warming treatment
Following the FATI apparatus design developed by Nijs et al. (Citation1996), Kimball (Citation2005) and Dong et al. (Citation2011), we designed an experimental warming apparatus with two 1-kW far-infrared heating tubes (1.5 m long, 60 cm apart) made by Shanghai Halo Infrared Technology Co. Ltd., China. They were placed 0.5 m (transplanting stage) to 1.7 m (flowering stage) apart on steel column pipe supports and surrounded by a resin film that allowed for 98% light transmittance and was open on the top. The experiment involved four treatments (all-day warming, AW; daytime warming from 07:00 to 19:00, DW; nighttime warming from 19:00 to 07:00, NW, and a control, CK) and covered the time from transplanting to maturation. Each treatment included three replicate plots, which were placed in a randomized block design. Twenty-four buckets of rice (four buckets of rice were placed in width, six buckets of rice were placed in length) were grown in one plot (1.2 m width × 2.0 m length). Also, all buckets’ positions were not changed during the growth period. The apparatus had a 4-m2 heating area and was able to induce remarkable increases in temperature (Dong et al. Citation2011). The daily mean temperatures of the crop canopy in the AW, DW and NW plots were 2.0, 1.1 and 1.3°C higher than those in the CK plots (Experiment 1), respectively, and 2.0, 0.9 and 1.2°C higher than those in the CK plots (Experiment 2), respectively. The canopy temperature data were obtained using a temperature recorder instrument (Hangzhou Zeda Instrument Co. Ltd., China), which could automatically record the instantaneous value every 30 min.
Taking Experiment 2 as an example, a displays the trends of canopy temperature variation during the flowering stage. It was observed that the changes in the canopy temperature under the three warming treatments were similar to those in the CK plots, which showed that the warming systems did not change the diurnal variation of the field temperature. The relative canopy temperatures under various scenarios were AW > NW > DW > CK, and the temperature variation trends for the remaining development stages were similar to those for the flowering stage. The daily mean temperatures of the crop canopy throughout the entire growth stage in the AW, DW and NW plots were 2.0, 1.0 and 1.3°C higher than those in the CK plots, respectively (b).
Sampling and analytical procedures
Sampling and measurements were performed at the jointing, anthesis and maturation stages. Three buckets of rice for each treatment (one bucket of rice was selected from one plot) were cut at ground level and separated into leaves and stems at jointing, leaves, stems and spike at anthesis, and leaves, stems, chaff and grain at maturity. All plant parts were oven-dried at 80°C to constant weight for dry weight determination. The plant samples were analyzed for N (micro-Kjeldahl) concentration, and N accumulation was calculated by multiplying the N concentration by the dry weight. The yield and yield components in each plot were determined by harvesting 16 buckets of rice after maturity. These plants were further air-dried for 3 weeks to a constant weight, after which the number of panicles was counted and the plants were carefully threshed. The 1000-grain weight was determined by randomly weighing 1000 grains. The actual yield was determined by weighing all grain for three replications. Afterwards, the grain was soaked in tap water, and the numbers of sunken and floating grains were counted to determine the grain-filling rate.
The following parameters related to N accumulation and remobilization within the rice plants during grain filling were calculated (Zhang et al. Citation2013):
Post-anthesis N accumulation = N content of the aboveground biomass at harvest- N content of the aboveground biomass at anthesis.
N translocation (NT) = N content of the aboveground biomass anthesis – N content of the leaves, stems and chaff at maturity.
N translocation efficiency (NTE) (%) = (N translocation amount/N content of the aboveground biomass at anthesis) × 100%.
Contribution of translocated N to grain (CTN) (%) = (N translocation amount/N content of grains at maturity) × 100.
N harvest index = (N content of grain/N content of aerial plant part)
N utilization efficiency based on grain yield = grain yield/N content of aerial plant part
N utilization efficiency based on biomass = aboveground biomass/N content of aerial plant part
Data analysis
Statistical analyses were performed using SPSS 12.0 (SPSS Inc., Chicago, IL, USA). Statistically significant differences were identified via least significant difference (LSD) calculations at p = 0.05. The standard errors of the means were also calculated and are presented in the graphs as error bars.
RESULTS
N accumulation and translocation
The total N accumulation in the three growth stages is presented in . In both growing seasons, the three warming treatments significantly increased the total N accumulation during the jointing, anthesis and maturity stages (p < 0.05), resulting in the following order of total N accumulation: DW > NW > AW > CK. In the AW, NW and DW treatments, the total N accumulation in the jointing stage increased by 20.56, 23.33 and 26.41% in 2011 and by 33.71, 35.80 and 40.53% in 2012, respectively. In the maturity stage, the warming treatment significantly increased the N concentration and accumulation in stems in both years (p < 0.05) but had no significant effect on the other biomass fractions ( and ).
Figure 2 Effect of asymmetric warming on the total nitrogen (N) accumulation of rice (Oryza sativa L.) in three growing stages. The vertical bars indicate the standard error (n = 3). Different lowercase letters between the treatments in each growth stage indicate significant differences at p < 0.05.
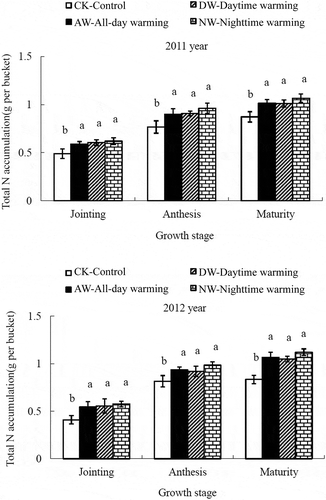
Figure 3 Effect of asymmetric warming on the nitrogen (N) concentrations in different plant organs at maturity. The vertical bars indicate the standard error (n = 3). Different lowercase letters treatments in each growth stage indicate significant differences at p < 0.05.
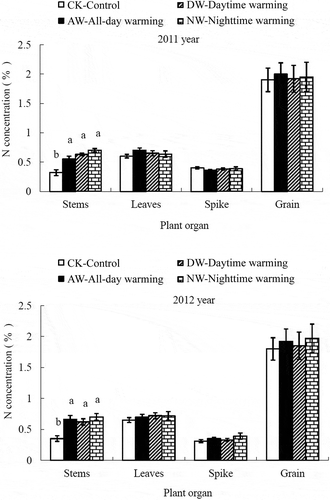
Figure 4 Effects of asymmetric warming on the nitrogen (N) accumulations in different plant organs at maturity. The vertical bars indicate the standard error (n = 3). Different lowercase letters between the treatments in each growth stage indicate significant differences at p < 0.05.
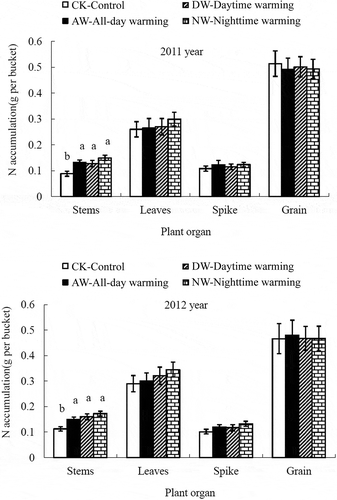
In both growing seasons, the warming treatment increased the amount of N translocation and significantly enhanced the contribution of translocated N to grain N. However, the N translocation efficiencies were lower in the warming plots than those in the CK plots. For example, in the AW, NW and DW treatments, the NTE decreased by 10.12, 10.72 and 15.35% in 2011 and by 14.87, 10.65 and 19.92% in 2012, respectively ().
Table 1 Effects of asymmetric warming on nitrogen (N) translocation from vegetative organs to grain after anthesis under a free air temperature increase (FATI) facility
Biomass, grain yield and yield components
The total aboveground biomass in the jointing stage varied between 27.79 and 30.11 g per bucket in the CK plots and between 31.06 and 34.98 g per bucket in the warming plots (). Significant effects of the warming treatment were observed on the aboveground biomass in the jointing and maturity stage in both growing seasons but not in the anthesis stage in 2012. In the AW, DW and NW treatments, the warming decreased aboveground biomass in the maturity stage by 9.63, 4.86 and 2.75% in 2011 and by 9.40, 8.58 and 4.31% in 2012, respectively.
Table 2 Effects of asymmetric warming on the aboveground biomass of rice (Oryza sativa L.) in three growing stages under a free air temperature increase (FATI) facility
In the AW, DW and NW treatments, the grain yield of the Huaidao5 cultivar declined by 9.58, 5.23 and 7.81% in 2011 and by 10.55, 4.88 and 7.97% in 2012, respectively (). Furthermore, the panicle number, grain number per panicle and grain filling rate decreased in the warmed plots, whereas the 1000-grain weight did not exhibit consistent changes. Compared with the CK treatment, the actual yield, panicle number and grain filling rate were only significantly different in the AW treatment in both years, whereas other yield components exhibited no significant differences among the four treatments (p < 0.05).
Table 3 Effects of asymmetric warming on rice (Oryza sativa L.) grain yield and components at maturity in both years
N utilization efficiency
The warming treatment significantly decreased the N harvest index and N utilization efficiency based on grain yield in both growing seasons (p < 0.05) and the N utilization efficiencies based on biomass in 2012 (). For example, in the AW, DW and NW treatments, warming decreased N utilization efficiency based on grain yield by 13.30, 16.07 and 9.32% in 2011 and 16.39, 19.44 and 12.37% in 2012, respectively.
Table 4 Effects of asymmetric warming on nitrogen (N) harvest indexes and N utilization efficiencies under a free air temperature increase (FATI) facility
DISCUSSION
Effects of asymmetric warming on grain yield and aboveground biomass
The results of the present study show that the warming treatments decreased the actual rice yield from 4.88 to 13.05%. Similarly, a previous study demonstrated that a high temperature of 36°C significantly decreased rice grain yield at the heading stage (Zheng Citation2003). Furthermore, Mohammed and Tarpley (Citation2011) observed that increasing the nighttime temperature by 5°C decreased rice grain yield by 90.00%. However, the elevated carbon dioxide (CO2) associated with climate change increased rice photosynthetic rate, biomass and grain yield (Yang et al. Citation2010). To accurately assess the response of rice growth and yield to potential climate change, a complex impact study on rice growth and yield for both elevated CO2 and air temperature is being conducted.
Grain yield is determined by the panicle number, grain number per panicle and 1000-grain weight. In the present study, the warming treatments decreased the panicle number, grain number per panicle and 1000-grain weight as well as the grain-filling rate. The results for the two study years were similar, and the relative reductions followed the order CK > DW > NW > AW. However, the actual yield, panicle number and grain filling rate were only significantly different for AW and CK in both years (p < 0.05). The grain number per panicle and 1000-grain weight were not significantly different among the four treatments. Nighttime warming not only shortened the growth stage but also increased respiration consumption (Fang et al. Citation2012). The adverse impacts of nighttime warming were greater than those of daytime warming (Peng et al. Citation2004) but less than those of all-day warming. Zhang et al. (Citation2005) observed that early- and late-season rice yields would be reduced by 3.60 and 2.80%, respectively, in 2100 using a crop model. Although the yield reduction trends determined by analyzing the abovementioned models were similar to the trend observed in the present study, the extent of the reductions was associated with the significant temperature differences between daytime and nighttime. It was also shown that environmental temperature differences, especially daytime and nighttime differences, should be considered when applying crop models to reduce the uncertainty in the model predictions.
The warming treatment significantly increased the aboveground biomass by 9.26–16.18% at the jointing stage but decreased it by 2.75–9.63% at the maturity stage in both growing seasons. Similarly, previous studies reported that a night-warming treatment caused an 18.00% increase in daily net C accumulation despite strongly increasing the nighttime plant leaf C release via respiration (Wan et al. Citation2009). This result could be explained by the stimulatory effects of the warming treatment on the photosynthetic capacity of plant leaves during the vegetative growth stage. The sink-source hypothesis of plant photosynthesis indicates that warming treatment would stimulate plant photosynthesis through an increased drawdown of leaf carbohydrates, resulting in a much greater compensatory enhancement of the photosynthesis rate in subsequent days (Prieto et al. Citation2009). Another possible reason for this stimulating effect is the low temperatures in the jointing stage, which may have been below the threshold of the negative temperature effect. In both growing seasons, the above-ground biomass at maturity was lower in the warming plots than in the CK plots, mainly due to a strong reduction in productive spikes. In both growing seasons, higher pre-anthesis dry matter accumulation in the warming treatment did not translate to higher grain yields compared with the control. Lower grain yields in the warming treatment may have been associated with lower dry matter accumulation in the period from post-anthesis to maturity. These results are consistent with those of previous studies regarding rice plants, which indicated that warming treatments significantly decreased the percentage of new C assimilated during the reproductive stage in the ears but increased it in the stems (Cheng et al. Citation2010). The decrease in the harvest index resulted from the high C allocation to the vegetative organs under the high-warming treatment and may potentially threaten future rice production.
Effects of warming treatment on N accumulation and translocation
This study revealed a clear pattern in which warming treatment generally enhanced the N concentrations of rice stems at maturity relative to the CK plots. However, long-term warming experiments, which have mostly been conducted in natural ecosystems without N fertilization, have shown that high nighttime temperatures decrease the N concentrations of grass in prairies as a result of the dilution effect caused by increased dry matter accumulation. Recently, Cheng et al. (Citation2010) observed that the whole-plant N concentrations of rice plants were unaffected by nighttime warming treatment, whereas the living leaf N concentration increased significantly. The N concentration in plant leaves is generally closely associated with photosynthetic capacity. In our study, increased N concentration in living leaves under the warming treatment may have enhanced the net photosynthetic rate, thereby promoting the accumulation of carbohydrates, as supported by the 9.26–16.18% higher dry matter accumulation in the jointing period under the warming treatment compared with the CK plots.
We observed a significant increase in the total N accumulations in the whole plant under the warming treatment compared with that under the CK treatment at each growing stage. The results are consistent with previous studies conducted with rice crops and prairie grass (An et al. Citation2005; Cheng et al. Citation2010). The warming treatment increases plant N accumulation, likely due to elevated soil N mineralization in the warming plots and/or the increased N uptake ability of plant roots (Tingey et al. Citation2003). Although DW increased the C gain by photosynthesis and NW increased the C loss by night respiration, the higher-temperature treatment decreased the chlorophyll content of the rice, inhibited its photosynthesis and reduced its photosynthetic rate and photosynthetic product (Li Citation2003; Kanno et al. Citation2009; Kanno and Makino Citation2010). The continuous daytime average temperature during 3 d, which was over 32°C/5 h, would affect the growth and development of conventional rice varieties, and b shows that an average temperature above 32°C was commonly observed from the middle of July to the end of July in this study. This effect may be a primary cause of the insignificant difference in above-ground biomass between the DW treatment and NW treatment. Plant N concentration is mainly affected by the plant’s natural features (such as physiological characteristics or gene) and environmental factors (e.g. temperature), but the former factor is stronger than the latter (Mae Citation2011). In addition, the total N content of the soil used in this experiment was 1.06 g kg−1 (corresponding to very poor soil), and the amount of N fertilizer used in our experiment was 13.80 g m−2 (relatively low N fertilizer application; the amount of N fertilizer in the Yangtze River Basin was over 30.00 g m−2, Wang et al. Citation2003), and the N concentration of the rice plants was almost the same under the warming treatment due to the lower soil N content. The plant N accumulation was mainly affected by the N concentration and aboveground biomass. However, the impact of aboveground biomass was higher than that of N concentration (Zhang et al. Citation2013). The N accumulation of the rice plants did not differ significantly among the three warming treatments due to the insignificant difference in above-ground biomass.
The warming treatment exerted a profound effect on the seasonal patterns of N uptake processes, with warming increasing pre-anthesis N accumulation by 33.42–49.86% in both growing seasons but decreasing post-anthesis N accumulation by 10.88–26.55%. Generally, a higher pre-anthesis N accumulation is favorable for obtaining high grain yields because grain N mainly originates from the remobilization of N stored temporarily in vegetative plant parts (Masoni et al. Citation2007; Dordas Citation2009). However, in the present study, the warming treatment significantly decreased N translocation efficiency despite substantially increasing the absolute translocation efficiency. Thus, more N was retained in the vegetative parts during grain filling, which has also been reported in rice plants (Cheng et al. Citation2010). On the other hand, previous studies have shown that post-anthesis N uptake that goes directly to the grain is significantly correlated with grain yield for both low- and high-N conditions (Cox et al. Citation1985). In our study, reduced post-anthesis N uptake may have contributed to the decrease in grain yield under the warming treatment. Therefore, decreased N translocation efficiency together with reduced post-anthesis N uptake led to the reduction in the internal N utilization efficiency.
CONCLUSIONS
Warming treatments decreased the rice grain yield and yield components. The results for the two study years were similar, the measured properties, except for the 1000-grain weight, ranking as follows: CK > DW > NW > AW. The actual yield and effective panicles were only significantly different between the AW and CK plots in both years, whereas other yield components exhibited no significant differences among the four treatments. At the jointing, anthesis and maturity stages, the total N accumulation in the whole plant was 8.27to 40.53% higher in the warming treatment than in the CK plots. The warming treatment substantially increased pre-anthesis N accumulation and reduced post-anthesis N uptake.
ACKNOWLEDGMENTS
The authors acknowledge experimental help provided by Siqi Qiu, Lu Liu and Chenyu Xie. This study was supported by the Natural Science Foundation of China (Nos. 41205087, 41103039 and 41001190), the University Students Innovation Training Project in Jiangsu Province (No. 201310300064Y), the Natural Science Project of the University in Jiangsu Province (No. 12KJB170009) and the National Special Research Fund for the Non-profit Sector (No. GYHY201206020). We also acknowledge the funding support provided by the Priority Academic Development Program for Jiangsu Higher Education Institutions (PAPD) and Jiangsu Government Scholarship for Overseas Studies.
References
- An Y, Wan SQ, Zhou XH, Subedar AA, Wallace LL, Luo YQ 2005: Plant nitrogen concentration, use efficiency, and contents in a tall grass prairie ecosystem under experimental warming. Global Change Biol., 11, 1733–1744.
- Cheng WG, Sakai H, Yagi K, Hasegawa T 2009: Interactions of elevated CO2 and night temperature on rice growth and yield. Agric. For. Meteorol., 149, 51–58.
- Cheng WG, Sakai H, Yagi K, Hasegawa T 2010: Combined effects of elevated [CO2] and high night temperature on carbon assimilation, nitrogen absorption, and the allocations of C and N by rice (Oryza sativa L.). Agric. For. Meteorol., 150, 1174–1181. doi:10.1016/j.agrformet.2010.05.001
- Cheng GF, Zhang JH, Li BB, Zhang JH, Yang SB, Xie XJ 2008: Hyper-spectral and red edge characteristics for rice under different temperature stress levels. Jiangsu J. Agric. Sci., 5, 573–580.
- Cox MC, Qualset CO, Rains DW 1985: Genetic variation for nitrogen assimilation and translocation in wheat. II. Nitrogen assimilation in relation to grain yield and protein. Crop Sci., 25, 435–440. doi:10.2135/cropsci1985.0011183X002500030003x
- Dong WJ, Chen J, Zhang B, Tian YL, Zhang WJ 2011: Responses of biomass growth and grain yield of midseason rice to the anticipated warming with FATI facility in East China. Field Crops Res., 123, 259–265. doi:10.1016/j.fcr.2011.05.024
- Dordas C 2009: Dry matter, nitrogen and phosphorus accumulation, partitioning and remobilization as affected by N and P fertilization and source-sink relations. Eur. J. Agron., 30, 129–139. doi:10.1016/j.eja.2008.09.001
- Easterling DR, Horton B, Jones PD et al. 1997: Maximum and minimum temperature trends for the globe. Science, 277, 364–367. doi:10.1126/science.277.5324.364
- Fang SB, Tan KY, Ren SX, Zhang, X, Zhao, J 2012: Fields experiments in North China show no decrease in winter wheat yields with night temperature increased by 2.0–2.5°C. Sci. China Earth Sci., 55, 1021–1027. doi:10.1007/s11430-012-4404-5
- Harvey LD 1995: Warm days, hot nights. Nature, 377, 15–16. doi:10.1038/377015a0
- Intergovernmental Panel on Climate Change (IPCC) 2007: Summary for policy makers. In Climate Change 2007:Mitigation.Contribution of Working Group III to the Fourth Assessment Report of the intergovernmental Panel on Climate Change, Ed. Metz B, Davidson OR, Bosch PR, Dave R, Meyer LA. Cambridge University Press, Cambridge.
- Jonassona S, Castro J, Michelsen A 2004: Litter, warming and plants affect respiration and allocation of soil microbial and plant C,N and P in arctic mesocosms. Soil Biol. Biochem., 36, 1129–1139. doi:10.1016/j.soilbio.2004.02.023
- Kanno K, Mae T, Makino A 2009: High night temperature stimulates photosynthesis biomass production and growth during the vegetative stage of rice plants. Soil Sci. Plant Nutr., 55, 124–131. doi:10.1111/j.1747-0765.2008.00343.x
- Kanno K, Makino A 2010: Increased grain yield and biomass allocation in rice under cool night temperature. Soil Sci.Plant Nutr., 56, 412–417. doi:10.1111/j.1747-0765.2010.00473.x
- Kimball BA 2005: Theory and performance of an infrared heater for ecosystem warming. Global Change Biol., 11, 2041–2056.
- Klein JA, Harte J, Zhao XQ 2005: Dynamic and complex micro-climate responses to warming and grazing manipulations. Global Change Biol., 11, 1440–1451. doi:10.1111/j.1365-2486.2005.00994.x
- Li CD 2003: Analysis of numerous unfilled grain appeared in rice under high temperature. Shanxi J. Agric. Sci., 5, 45–47.
- Lobell DB, Asner GP 2003: Climate and management contributions to recent trends in US agricultural yields. Science, 299, 1032. doi:10.1126/science.1077838
- Lobell DB, Burke MB, Tebaldi C, Mastrandrea MD, Falcon WP, Naylor RL 2008: Prioritizing climate change adaptation needs for food security in 2030. Science, 319, 607–610. doi:10.1126/science.1152339
- Mae T 2011: Nitrogen acquisition and its relation to growth and yield in recent high-yielding cultivars of rice (Oryza Sativa L.) in Japan. Soil Sci. Plant Nutr., 57, 625–635. doi:10.1080/00380768.2011.602626
- Masoni A, Ercoli L, Mariotti M, Arduini I 2007: Post-anthesis accumulation and remobilization of dry matter, nitrogen and phosphorus in durum wheat as affected by soil type. Eur. J. Agron., 26, 179–186. doi:10.1016/j.eja.2006.09.006
- Mohammed AR, Tarpley L 2011: High night temperature and plant growth regulator effects on spikelet sterility, grain characteristics and yield of rice (Oryza sativa L.) plants. Can. J. Plant Sci., 91, 283–291. doi:10.4141/CJPS10038
- Nijs I, Kockelbergh F, Teughels H, Blum H, Hendrey G, Impens I 1996: Free air temperature increase (FATI): a new tool to study global warming effects on plants in the field. Plant Cell Environ., 19, 495–502. doi:10.1111/j.1365-3040.1996.tb00343.x
- Patil RH, Laegdsmand M, Olesen JE, Porter JR 2010: Effect of soil warming and rainfall patterns on soil N cycling in Northern Europe. Agric. Ecosyst. Environ., 139, 195–205. doi:10.1016/j.agee.2010.08.002
- Peng SP, Huang JL, Sheehy JE, Laza RC, Visperas RM, Zhong XH, Centeno GS, Khush GS, Cassman KG 2004: Rice yields decline with higher night temperature from global warming. Proc. Natl. Acad. Sci. U.S.A, 101, 9971–9975. doi:10.1073/pnas.0403720101
- Prieto P, Peñuelas J, Llusià J, Asensio D, Estiarte M 2009: Effects of long term experimental nighttime warming and drought on photosynthesis, Fv/Fm and stomatal conductance in the dominant species of a Mediterranean shrub land. Acta Physiol. Plant, 31, 729–739. doi:10.1007/s11738-009-0285-4
- Sheehy JE, Mitchell PL, Ferrer AB 2006: Decline in rice grain yields with temperature: models and correlations can give different estimates. Field Crops Res., 98, 151–156. doi:10.1016/j.fcr.2006.01.001
- Tao F, Hayashi Y, Zhang Z, Sakamoto T, Yokozawa T, Yokozawa, M 2008: Global warming, rice production, and water use in China: developing a probabilistic assessment. Agric. For. Meteorol., 148, 94–110. doi:10.1016/j.agrformet.2007.09.012
- Tingey DT, Mckane RB, Olszyk DM, Johnson MG, Rygiewicz PT, Lee EH 2003: Elevated CO2 and temperature alter nitrogen allocation in Douglas-fir. Global Change Biol., 9, 1038–1050. doi:10.1046/j.1365-2486.2003.00646.x
- Wan SQ, Xia JY, Liu WX, Niu SL 2009: Photosynthetic overcompensation under nocturnal warming enhances grassland carbon sequestration. Ecology, 90, 2700–2710. doi:10.1890/08-2026.1
- Wang SH, Cao WX, Ding YF, Liu SH, Wang QS 2003: Effects of planting density and nitrogen application rate on absorption and utilization of nitrogen in rice. J. Nanjing Agric. Univ., 26, 1–4.
- Xu XX, Zhou GS 2005: Effects of water stress and high nocturnal temperature on photosynthesis and nitrogen level of aperennialgrass Leymuschinensis. PlantSoil, 269, 131–139.
- Yang LX, Wang YX, Zhu JG, Hasegawa T, Wang YL 2010: What have we learned from 10 years of free-air CO2 enrichment (FACE) experiments on rice? Growth and development. Acta Ecol. Sin., 30, 1573–1585.
- Zhang YH, Li RY, Wang YL 2013: Night-time warming affects N and P dynamics and productivity of winter wheat plants. Can. J. Plant Sci., 93, 397–406. doi:10.4141/cjps2012-044
- Zhang JP, Zhao YX, Wang CY, He Y 2005: Effects of climate change on the growth and yields of double-harvest rice in the southern China. Adv. Climate Change Res., 1, 151–156.
- Zheng ZG 2003: The influence of temperature and light on grain-filling, dry matter production of rice. J. Beijing Agric. Coll., 18, 14–16.